Hot Jupiter
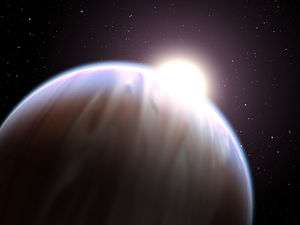

Hot Jupiters (also called roaster planets,[1] epistellar jovians,[2][3] pegasids[4][5] or pegasean planets) are a class of gas giant exoplanets that are inferred to be physically similar to Jupiter but that have very short orbital period (P<10 days)[6]. The close proximity to their stars and high surface-atmosphere temperatures resulted in the moniker "hot Jupiters".[7]
Hot Jupiters are the easiest extrasolar planets to detect via the radial-velocity method, because the oscillations they induce in their parent stars' motion are relatively large and rapid compared to those of other known types of planets. One of the best-known hot Jupiters is 51 Pegasi b. Discovered in 1995, it was the first extrasolar planet found orbiting a Sun-like star. 51 Pegasi b has an orbital period of about 4 days.
General characteristics
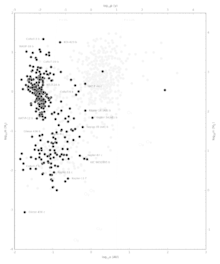
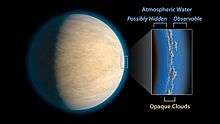
Though there is diversity among hot Jupiters, they do share some common properties.
- Their defining characteristics are their large masses and short orbital periods, spanning 0.36–11.8 Jupiter masses and 1.3–111 Earth days.[9] The mass cannot be greater than approximately 13.6 Jupiter masses because then the planet would start burning deuterium and become a brown dwarf.[10]
- Most have nearly circular orbits (low eccentricities). It is thought that their orbits are circularized by perturbations from nearby stars or tidal forces.[11]
- Many have unusually low densities. The lowest one measured thus far is that of TrES-4 at 0.222 g/cm3.[12] The large radii of hot Jupiters are not yet fully understood but it is thought that the expanded envelopes can be attributed to high stellar irradiation, high atmospheric opacities, possible internal energy sources, and orbits close enough to their stars for the outer layers of the planets to exceed their Roche limit and be pulled further outward.[12][13]
- They are likely to have extreme and exotic atmospheres due to their short periods, relatively long days, and tidal locking. Atmospheric dynamics models predict strong vertical stratification with intense winds and super-rotating equatorial jets driven by radiative forcing and the transfer of heat and momentum.[14][15] The day-night temperature difference at the photosphere is predicted to be substantial, approximately 500 K for a model based on HD 209458b.[15]
- They appear to be more common around F- and G-type stars and less so around K-type stars. Hot Jupiters around red dwarfs are very rare.[16] Generalizations about the distribution of these planets must take into account the various observational biases.
Formation and evolution
There are two general schools of thought regarding the origin of hot Jupiters: formation at a distance followed by inward migration and in-situ formation at the distances at which they're currently observed. The prevalent view is migration.
Migration
In the migration hypothesis, a hot Jupiter forms beyond the frost line, from rock, ice, and gases via the core accretion method of planetary formation. The planet then migrates inwards to the star where it eventually forms a stable orbit.[17][18] The planet may have migrated inward smoothly via type II orbital migration.[19][20] Or it may have migrated more suddenly due to gravitational scattering onto eccentric orbits during an encounter with another massive planet, followed by the circularization and shrinking of the orbits due to tidal interactions with the star. A hot Jupiter's orbit could also have been altered via the Kozai mechanism, causing an exchange of inclination for eccentricity resulting in a high eccentricity low perihelion orbit, in combination with tidal friction. This requires a massive body -- another planet or a stellar companion -- on a more distant and inclined orbit; approximately 50% of hot Jupiters have distant Jupiter-mass or larger companions, which can leave the hot Jupiter with an orbit inclined relative to the star's rotation.[21]
The type II migration happens during the solar nebula phase, i.e. when gas is still present. Energetic stellar photons and strong stellar winds at this time remove most of the remaining nebula. Migration via the other mechanism can happen after the loss of the gas disk.
In situ
Instead of being gas giants that migrated inward, in an alternate hypothesis the cores of the hot Jupiters began as more common super-Earths which accreted their gas envelopes at their current locations, becoming gas giants in situ. The super-Earths providing the cores in this hypothesis could have formed either in situ or at greater distances and have undergone migration before acquiring their gas envelopes. Since super-Earths are often found with companions, the hot Jupiters formed in situ could also be expected to have companions. The increase of the mass of the locally growing hot Jupiter has a number of possible effects on neighboring planets. If the hot Jupiter maintains an eccentricity greater than 0.01, sweeping secular resonances can increase the eccentricity of a companion planet, causing it to collide with the hot Jupiter. The core of the hot Jupiter in this case would be unusually large. If the hot Jupiter's eccentricity remains small the sweeping secular resonances could also tilt the orbit of the companion.[22] Traditionally, the in situ mode of conglomeration has been disfavored because the assembly of massive cores, which is necessary for the formation of hot Jupiters, requires surface densities of solids ≈ 104 g/cm2, or larger.[23][24][25] Recent surveys, however, have found that the inner regions of planetary systems are frequently occupied by super-Earth type planets.[26][27] If these super-Earths formed at greater distances and migrated closer, the formation of in situ hot Jupiters is not entirely in situ.
Atmospheric loss
If the atmosphere of a hot Jupiter is stripped away via hydrodynamic escape, its core may become a chthonian planet. The amount of gas removed from the outermost layers depends on the planet's size, the gases forming the envelope, the orbital distance from the star, and the star's luminosity. In a typical system, a gas giant orbiting at 0.02 AU around its parent star loses 5–7% of its mass during its lifetime, but orbiting closer than 0.015 AU can mean evaporation of a substantially larger fraction of the planet's mass.[28] It should be noted that no such objects have been found yet and they are still hypothetical.
Terrestrial planets in systems with hot Jupiters
Simulations have shown that the migration of a Jupiter-sized planet through the inner protoplanetary disk (the region between 5 and 0.1 AU from the star) is not as destructive as one might assume. More than 60% of the solid disk materials in that region are scattered outward, including planetesimals and protoplanets, allowing the planet-forming disk to reform in the gas giant's wake.[29] In the simulation, planets up to two Earth masses were able to form in the habitable zone after the hot Jupiter passed through and its orbit stabilized at 0.1 AU. Due to the mixing of inner-planetary-system material with outer-planetary-system material from beyond the frost line, simulations indicated that the terrestrial planets that formed after a hot Jupiter's passage would be particularly water-rich.[29]
In 2015, two planets were discovered around WASP-47. One was potentially a large terrestrial planet, of less than 22 Earth masses and 1.8 Earth radii. The other is of similar mass at 15.2 Earth masses, but with 3.6 Earth radii it is almost certainly a gas giant. They orbit on either side of a previously discovered hot Jupiter, with the smaller, terrestrial planet closer in.[30] A similar orbital architecture is also exhibited by the Kepler-30 system.[31]
Retrograde orbit
It has been found that several hot Jupiters have retrograde orbits and this calls into question the theories about the formation of planetary systems,[32] although rather than a planet's orbit having been disturbed, it may be that the star itself flipped over early in their system's formation due to interactions between the star's magnetic field and the planet-forming disc.[33] By combining new observations with the old data it was found that more than half of all the hot Jupiters studied have orbits that are misaligned with the rotation axis of their parent stars, and six exoplanets in this study have retrograde motion.
Recent research has found that several hot Jupiters are in misaligned systems.[34][35] This misalignment may be related to the heat of the photosphere the hot Jupiter is orbiting. There are many proposed theories as to why this might occur. One such theory involves tidal dissipation and suggests there is a single mechanism for producing hot Jupiters and this mechanism yields a range of obliquities. Cooler stars with higher tidal dissipation damps the obliquity (explaining why hot Jupiters orbiting cooler stars are well aligned) while hotter stars do not damp the obliquity (explaining the observed misalignment).[9]
Ultra-short-period Jupiters
Ultra-short-period Jupiters are a class of hot Jupiters with orbital periods below one day and occur only around stars of less than about 1.25 solar masses.[36]
Five ultra-short-period planet candidates have been identified in the region of the Milky Way known as the galactic bulge. They were observed by the Hubble Space Telescope and first described by researchers from the Space Telescope Science Institute, the Universidad Catolica de Chile, Uppsala University, the High Altitude Observatory, the INAF–Osservatorio Astronomico di Padova, and the University of California, Los Angeles.[36]
Confirmed transiting hot Jupiters that have orbital periods of less than one day include WASP-18b, WASP-19b, WASP-43b, and WASP-103b.[37]
Puffy planets
Gas giants with a large radius and very low density are sometimes called "puffy planets"[38] or "hot Saturns", due to their density being similar to Saturn's. Puffy planets orbit close to their stars so that the intense heat from the star combined with internal heating within the planet will help inflate the atmosphere. Six large-radius low-density planets have been detected by the transit method. In order of discovery they are: HAT-P-1b,[39][40] COROT-1b, TrES-4, WASP-12b, WASP-17b, and Kepler-7b. Some hot Jupiters detected by the radial-velocity method may be puffy planets. Most of these planets are below two Jupiter masses as more massive planets have stronger gravity keeping them at roughly Jupiter's size.
Even when taking surface heating from the star into account, many transiting hot Jupiters have a larger radius than expected. This could be caused by the interaction between atmospheric winds and the planet's magnetosphere creating an electric current through the planet that heats it up, causing it to expand. The hotter the planet, the greater the atmospheric ionization, and thus the greater the magnitude of the interaction and the larger the electric current, leading to more heating and expansion of the planet. This theory matches the observation that planetary temperature is correlated with inflated planetary radii.[41]
Moons
Theoretical research suggests that hot Jupiters are unlikely to have moons, due to both a small Hill sphere and the tidal forces of the stars they orbit, which would destabilize any satellite's orbit, the latter process being stronger for larger moons. This means that for most hot Jupiters, stable satellites would be small asteroid-sized bodies.[42] In spite of this, observations of WASP-12b suggest that it is orbited by at least 1 large exomoon.[43]
Hot Jupiters around red giants
It has been proposed that, even though no planet of this type has been found until now, gas giants orbiting red giants at distances similar to that of Jupiter could be hot Jupiters due to the intense irradiation they would receive from their stars. It is very likely that in the Solar System Jupiter will become a hot Jupiter after the transformation of the Sun into a red giant.[44]
Hot Jupiters orbiting red giants would differ from those orbiting main-sequence stars in a number of ways, most notably the possibility of accreting material from the stellar winds of their stars and, assuming a fast rotation (not tidally locked to their stars), a much more evenly distributed heat with many narrow-banded jets. Their detection using the transit method would be much more difficult due to their tiny size compared to the stars they orbit, as well as the long time needed (months or even years) for one to transit their star as well as to be occulted by it.[44]
See also
References
- ↑ Sharp, A. G.; Moses, J. I.; Friedson, A. J.; Fegley, B.; Marley, M. S.; Lodders, K. (2004), "Predicting the Atmospheric Composition of Extrasolar Giant Planets" (PDF), 35th Lunar and Planetary Science Conference, Lunar and Planetary Science Conference, 35: 1152, Bibcode:2004LPI....35.1152S
- ↑ Darling, David, epistellar jovians, The Internet Encyclopedia of Science
- ↑ Odenwald, Sten, What is an "Epistellar Jovian Exoplanet"?, The Astronomy Cafe
- ↑ Interiors of extrasolar planets: A first step (PDF), Astronomy & Astrophysics, 2006-05-30
- ↑ Than, Ker (2006-06-05), Inside Exoplanets: Motley Crew of Worlds Share Common Thread, Space.com
- ↑ ON THE OCCURRENCE RATE OF HOT JUPITERS IN DIFFERENT STELLAR ENVIRONMENTS
- ↑ "What worlds are out there?". Canadian Broadcasting Corporation. 25 August 2016. Retrieved 5 June 2017.
- ↑ "Hot Jupiter with hidden Water". spacetelescope.org. ESA/Hubble. Retrieved 13 June 2016.
- 1 2 Winn, Joshua N.; Fabrycky, Daniel; Albrecht, Simon; Johnson, John Asher (2010-01-01). "Hot Stars with Hot Jupiters Have High Obliquities". The Astrophysical Journal Letters. 718 (2): L145. ISSN 2041-8205. doi:10.1088/2041-8205/718/2/L145.
- ↑ "Astronomy & Astrophysics (A&A)". www.aanda.org. Retrieved 2016-04-15.
- ↑ Fabrycky, D.; Tremaine, S. (2007-11-10). "Shrinking Binary and Planetary Orbits by Kozai Cycles with Tidal Friction". Astrophysical Journal. 669: 1298–1315. Bibcode:2007ApJ...669.1298F. doi:10.1086/521702.
- 1 2 Mandushev, Georgi; O'Donovan, Francis T.; Charbonneau, David; Torres, Guillermo; Latham, David W.; Bakos, Gáspár Á.; Dunham, Edward W.; Sozzetti, Alessandro; Fernández, José M. (2007-10-01). "TrES-4: A Transiting Hot Jupiter of Very Low Density". The Astrophysical Journal. 667 (2): L195–L198. Bibcode:2007ApJ...667L.195M. doi:10.1086/522115.
- ↑ Burrows, A.; Hubeny, I.; Budaj, J.; Hubbard, W. B. (2007-01-01). "Possible Solutions to the Radius Anomalies of Transiting Giant Planets". The Astrophysical Journal. 661 (1): 502–514. Bibcode:2007ApJ...661..502B. ISSN 0004-637X. doi:10.1086/514326.
- ↑ Cooper, Curtis S.; Showman, Adam P. (2005-01-01). "Dynamic Meteorology at the Photosphere of HD 209458b". The Astrophysical Journal Letters. 629 (1): L45. Bibcode:2005ApJ...629L..45C. ISSN 1538-4357. doi:10.1086/444354.
- 1 2 Rauscher, Emily; Menou, Kristen (2010-01-01). "Three-dimensional Modeling of Hot Jupiter Atmospheric Flows". The Astrophysical Journal. 714 (2): 1334–1342. Bibcode:2010ApJ...714.1334R. ISSN 0004-637X. doi:10.1088/0004-637X/714/2/1334.
- ↑ Characterizing the Cool KOIs II. The M Dwarf KOI-254 and its Hot Jupiter: John Asher Johnson, J. Zachary Gazak, Kevin Apps, Philip S. Muirhead, Justin R. Crepp, Ian J. M. Crossfield, Tabetha Boyajian, Kaspar von Braun, Barbara Rojas-Ayala, Andrew W. Howard, Kevin R. Covey, Everett Schlawin, Katherine Hamren, Timothy D. Morton, James P. Lloyd
- ↑ Chambers, John (2007-07-01). Planet Formation with Type I and Type II Migration. AAS/Division of Dynamical Astronomy Meeting. 38. Bibcode:2007DDA....38.0604C.
- ↑ D'Angelo, Gennaro; Durisen, Richard H.; Lissauer, Jack J. (December 2010). "Giant Planet Formation". In Seager, Sara. Exoplanets. University of Arizona Press. pp. 319–346. Bibcode:2010exop.book..319D. ISBN 978-0-8165-2945-2. arXiv:1006.5486
.
- ↑ D'Angelo, G.; Lubow, S. H. (2008). "Evolution of Migrating Planets Undergoing Gas Accretion". The Astrophysical Journal. 685 (1): 560–583. Bibcode:2008ApJ...685..560D. arXiv:0806.1771
. doi:10.1086/590904.
- ↑ Lubow, S. H.; Ida, S. (2011). "Planet Migration". In S. Seager. Exoplanets. University of Arizona Press, Tucson, AZ. pp. 347–371. Bibcode:2011exop.book..347L. arXiv:1004.4137
.
- ↑ Knutson, Heather A.; Fulton, Benjamin J.; Montet, Benjamin T.; Kao, Melodie; Ngo, Henry; Howard, Andrew W.; Crepp, Justin R.; Hinkley, Sasha; Bakos, Gaspar Á (2014-01-01). "Friends of Hot Jupiters. I. A Radial Velocity Search for Massive, Long-period Companions to Close-in Gas Giant Planets". The Astrophysical Journal. 785 (2): 126. Bibcode:2014ApJ...785..126K. ISSN 0004-637X. doi:10.1088/0004-637X/785/2/126.
- ↑ Batygin, Konstantin; Bodenheimer, Peter H.; Laughlin, Gregory P. (2016). "In Situ Formation and Dynamical Evolution of Hot Jupiter Systems". The Astrophysical Journal. 829 (2): 114. arXiv:1511.09157
. doi:10.3847/0004-637X/829/2/114.
- ↑ Rafikov, Roman R. (2006-01-01). "Atmospheres of Protoplanetary Cores: Critical Mass for Nucleated Instability". The Astrophysical Journal. 648 (1): 666–682. Bibcode:2006ApJ...648..666R. ISSN 0004-637X. doi:10.1086/505695.
- ↑ Hayashi, Chushiro (1981-01-01). "Structure of the Solar Nebula, Growth and Decay of Magnetic Fields and Effects of Magnetic and Turbulent Viscosities on the Nebula". Progress of Theoretical Physics Supplement. 70: 35–53. ISSN 0375-9687. doi:10.1143/PTPS.70.35.
- ↑ D'Angelo, G.; Bodenheimer, P. (2016). "In Situ and Ex Situ Formation Models of Kepler 11 Planets". The Astrophysical Journal. 828: in press. Bibcode:2016ApJ...828...33D. arXiv:1606.08088
. doi:10.3847/0004-637X/828/1/33.
- ↑ Mayor, M.; Marmier, M.; Lovis, C.; Udry, S.; Ségransan, D.; Pepe, F.; Benz, W.; Bertaux, J.-L.; Bouchy, F. (2011-09-12). "The HARPS search for southern extra-solar planets XXXIV. Occurrence, mass distribution and orbital properties of super-Earths and Neptune-mass planets". arXiv:1109.2497
[astro-ph].
- ↑ Batalha, Natalie M.; Rowe, Jason F.; Bryson, Stephen T.; Barclay, Thomas; Burke, Christopher J.; Caldwell, Douglas A.; Christiansen, Jessie L.; Mullally, Fergal; Thompson, Susan E. (2013-01-01). "Planetary Candidates Observed by Kepler. III. Analysis of the First 16 Months of Data". The Astrophysical Journal Supplement Series. 204 (2): 24. ISSN 0067-0049. doi:10.1088/0067-0049/204/2/24.
- ↑ "Exoplanets Exposed to the Core". 2009-04-25. Retrieved 2009-04-25.
- 1 2 Fogg, Martyn J.; Nelson, Richard P. (2007), "On the formation of terrestrial planets in hot-Jupiter systems", Astronomy and Astrophysics, 461 (3): 1195–1208, Bibcode:2007A&A...461.1195F, arXiv:astro-ph/0610314
, doi:10.1051/0004-6361:20066171.
- ↑ Becker, Juliette C.; et al. (10 August 2015). "WASP-47: A Hot Jupiter System with Two Additional Planets Discovered by K2". The Astrophysical Journal Letters (Letter). IOP Publishing (published October 2015). 812 (2): L18. Bibcode:2015ApJ...812L..18B. arXiv:1508.02411
. doi:10.1088/2041-8205/812/2/L18.
The mass of WASP-47d is 15.2±7 M⊕. Only an upper limit can be placed on WASP-47e of <22M⊕.
- ↑ [NULL]. "Kepler: A far-off solar system". kepler.nasa.gov. Retrieved 2016-08-02.
- ↑ "Turning planetary theory upside down", ESO Press Release, Royal Astronomical Society: 16, 2010-04-13, Bibcode:2010eso..pres...16.
- ↑ Tilting stars may explain backwards planets, New Scientist, 01 September 2010, Magazine issue 2776.
- ↑ Hebrard, G.; Desert, J.-M.; Diaz, R. F.; Boisse, I.; Bouchy, F.; Etangs, A. Lecavelier des; Moutou, C.; Ehrenreich, D.; Arnold, L. "Observation of the full 12-hour-long transit of the exoplanet HD80606b. Warm-Spitzer photometry and SOPHIE spectroscopy". Astronomy and Astrophysics. 516: A95. Bibcode:2010A&A...516A..95H. ISSN 0004-6361. arXiv:1004.0790
. doi:10.1051/0004-6361/201014327.
- ↑ Triaud, A. H. M. J.; Queloz, D.; Bouchy, F.; Moutou, C.; Collier Cameron, A.; Claret, A.; Barge, P.; Benz, W.; Deleuil, M. (2009-10-01). "The Rossiter-McLaughlin effect of CoRoT-3b and HD 189733b". Astronomy and Astrophysics. 506: 377–384. Bibcode:2009A&A...506..377T. ISSN 0004-6361. doi:10.1051/0004-6361/200911897.
- 1 2 Sahu; et al. (2006). "Transiting extrasolar planetary candidates in the Galactic bulge" (abstract). Nature. 443 (7111): 534–540. Bibcode:2006Natur.443..534S. PMID 17024085. arXiv:astro-ph/0610098
. doi:10.1038/nature05158.
- ↑ WASP Planets
- ↑ Chang, Kenneth (2010-11-11). "Puzzling Puffy Planet, Less Dense Than Cork, Is Discovered". The New York Times.
- ↑ Ker Than (2006-09-14). "Puffy 'Cork' Planet Would Float on Water". Space.com. Retrieved 2007-08-08.
- ↑ "Puffy planet poses pretty puzzle". BBC News. 2006-09-15. Retrieved 2010-03-17.
- ↑ http://arxiv.org/pdf/1101.3800v4.pdf
- ↑ "Stability of Satellites around Close-in Extrasolar Giant Planets
- ↑ Российские астрономы впервые открыли луну возле экзопланеты (in Russian) – "Studying of a curve of change of shine of WASP-12b has brought to the Russian astronomers unusual result: regular splashes were found out.<...> Though stains on a star surface also can cause similar changes of shine, observable splashes are very similar on duration, a profile and amplitude that testifies for benefit of exomoon existence."
- 1 2 Spiegel, David S.; Madhusudhan, Nikku (2012-09-01). "Jupiter will Become a Hot Jupiter: Consequences of Post-main-sequence Stellar Evolution on Gas Giant Planets". The Astrophysical Journal. 756: 132. Bibcode:2012ApJ...756..132S. ISSN 0004-637X. doi:10.1088/0004-637X/756/2/132.
External links
- Inside Exoplanets: Motley Crew of Worlds Share Common Thread
- NASA: Global temperature map of an exoplanet* First known theoretical prediction about existence of Hot Jupiters – by Otto Struve in 1952.
- "Proposal for a Project of High-Precision Stellar Radial Velocity Work". Otto Struve proposal, July 24, 1952.
- Audio: Cain/Gay Hot Jupiters and Pulsar Planets – Sept 2006.