Tsuji-Wilkinson decarbonylation reaction
Tsuji-Wilkinson Decarbonylation Reaction | |
---|---|
Named after | Jiro Tsuji Sir Geoffrey Wilkinson |
Reaction type | Decarbonylation |
Identifiers | |
Organic Chemistry Portal | Decarbonylation Reaction.shtm Tsuji-Wilkinson Decarbonylation Reaction |
The Tsuji-Wilkinson decarbonylation reaction is a method for the direct decarbonylation of aliphatic, aromatic, as well as α, β-unsaturated aldehydes into the corresponding C(sp3)–H or C(sp2)–H bonds.[1][2][3][4] Further, the Tsuji-Wilkinson Decarbonylation may be used to describe the decarbonylation of acyl halides.[2]

Though the reaction may be carried out by several different transition metal complexes (e.g. palladium complexes),[5] typically the most efficient complex capable of carrying out the decarbonylations is the Wilkinson's catalyst. Unfortunately, the reaction usually requires stoichiometric amounts of Wilkinson’s catalyst due to the formation of a Rh–CO intermediate; however, if the reaction is carried out above 200 °C, carbon monoxide may be liberated efficiently enough and the reaction may proceed with catalytic amount of complex. Other transition metals such as Iridium(I) may obviate this issue.[6]
The Tsuji-Wilkinson Decarbonylation has had many synthetic applications even in its stoichiometric iteration. This is also because the reaction proceeds under mild conditions and is highly stereospecific (e.g. stereocenter configuration in which a formyl moiety is bonded is retained). The Tsuji-Wilkinson Decarbonylation has been used on aliphatic, aromatic, and α, β-unsaturated aldehydes as well as acyl nitriles, and 1,2-diketones.
History
In 1965, Jiro Tsuji reported that stoichiometric amounts of Wilkinson’s Catalytst (RhCl(PPh3)3) was capable of decarbonylating cinnamaldehyde.[1] In 1968, Tsuji again showed Wilkinson’s catalyst can decarbonylate phenacetyl chloride to benzyl chloride.[2]
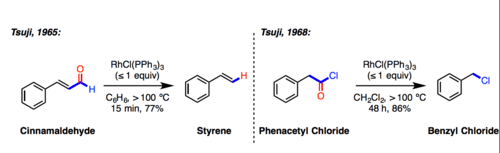
For more recent developments in reaction improvement, see below: #Efforts to render a low temperature catalytic Tsuji-Wilkinson decarbonylation.
Catalytic cycle
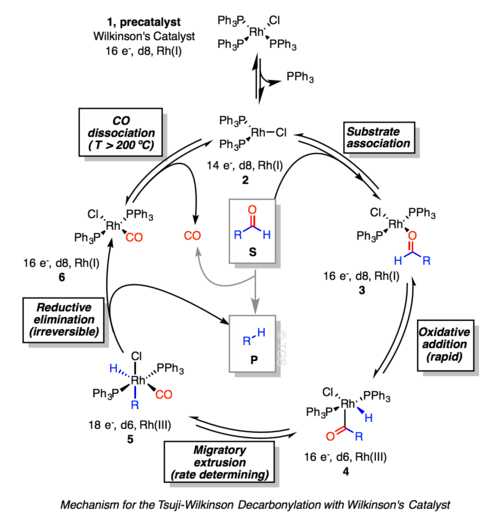
The catalytic cycle of the Tsuji-Wilkinson Decarbonylation begins with the 16 electron d8 Rh(I) Wilkinson's catalyst (RhCl(PPh3)3 (1) which acts as a precatalyst. Upon triphenylphosphine dissociation, a 14 electron d8 Rh(I) species (2) is formed which immediately complexes aldehydic carbonyls of the starting material (S), forming the 16 electron d8 Rh(I) species (3) which is primed for oxidative addition to form 4, the 16 e- d6 acyl Rh(III)-hydride adduct. Migratory extrusion of CO may then proceed, which is reversible and rate determining (akin to a pre-equilibrium) to form the 18 e- d6 Rh(III) species, 5. 5 may then undergo irreversible reductive elimination to form the 16 e- d8 Rh(I) species 6 and liberate product (P). Rh(I)-carbonyl 6 may lose CO provided the reaction is heated above 200 °C, allowing for CO dissociation thereby regenerating 1 (the catalytic variant). Otherwise, the reaction mechanism halts by formation of the thermodynamically stable 6 (the stoichiometric variant).[6]
Synthetic Applications
The Tsuji-Wilkinson Decarbonylation has been used numerous times in the total synthesis of natural products. Ziegler and colleagues have used Tsuji-Wilkinson Decarbonylation in the synthesis of the core nucleus of FR-900482.[7] Note that the ester is left unscathed.
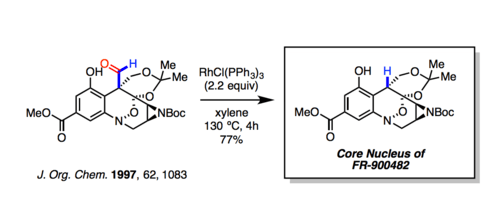
Recently, Snyder and colleagues used the Tsuji-Wilkinson decarbonylation in second-to-last step of their synthesis of (–)-presilphiperfolan-8-ol.[8] They comment “Of note in these final steps, separate reduction and oxidation steps proceeded in inferior yield in generating 38 (70% versus 93%),32 while the Rh(PPh3)3Cl operation proceeded smoothly when conducted on small scale (∼15 mg). In total, the synthesis required 13 steps from commercial [starting material], and ∼15 mg of [(–)-presilphiperfolan-8-ol] has been prepared with spectral properties and optical rotations matching that of the natural isolate.”

Efforts to render a low temperature catalytic Tsuji-Wilkinson decarbonylation
The drawback of the Tsuji-Wilkinson reaction is twofold and requires the necessity of stoichiometric Wilkinson’s catalyst or the need for high temperatures with catalytic amounts of Wilkinson’s catalyst. The ideal Tsuji-Wilkinon Decarbonylation would be typified by catalytic (e.g. 10 mol% catalyst) at mild temperatures. Relatively recently, the reaction has been carried out in flow conditions at low temperatures in which a biphasic liquid-gas flow decarbonylation was developed employing N2 as a gas carrier.[9] However, the temperature required for this reaction is 200 ºC
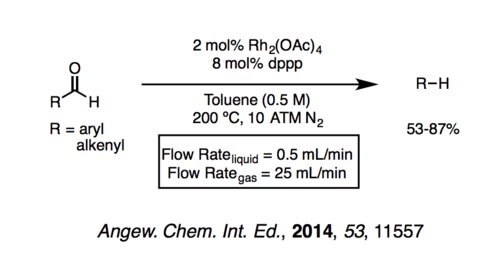
Significant improvements of the Tsuji-Wilkinson Decarbonylation have been made by using cationic rhodium complexes with chelating bisphosphines (which increase the rate of reductive elimination and lower the energetic barrier for CO extrusion).[10] These complexes have less electron density, therefore reducing backbonding into CO and therefore have significantly weaker metal-CO bonds. Improvements to this area of research are actively underway.
References
- 1 2 Tsuji, Jiro; Ohno, Kiyotaka (January 1965). "Organic syntheses by means of noble metal compounds XXI. Decarbonylation of aldehydes using rhodium complex". Tetrahedron Letters. 6 (44): 3969–3971. doi:10.1016/S0040-4039(01)89127-9.
- 1 2 3 Ohno, Kiyotaka; Tsuji, Jiro (1968). "Organic synthesis by means of noble metal compounds. XXXV. Novel decarbonylation reactions of aldehydes and acyl halides using rhodium complexes". Journal of the American Chemical Society. 90 (1): 99–107.
- ↑ Tsuji, Jiro; Ohno, Kiyotaka; Kajimoto, Tsunesuke (January 1965). "Organic syntheses by means of noble metal compounds XX. Decarbonylation of acyl chloride and aldehyde catalyzed by palladium and its relationship with the rosenmund reduction.". Tetrahedron Letters. 6 (50): 4565–4568. doi:10.1016/S0040-4039(01)89065-1.
- ↑ Synthesis, 1969, 157
- ↑ Laszlo Kurti; Barbara Czako (2005). Strategic Applications of Named Reactions in Organic Synthesis: Background and Detailed Mechanism. ISBN 0124297854.
- 1 2 Doughty, D. H.; Pignolet, L. H. (October 1978). "Catalytic decarbonylation of aldehydes". Journal of the American Chemical Society. 100 (22): 7083–7085.
- ↑ Ziegler, Frederick E.; Belema, Makonen (February 1997). "Chiral Aziridinyl Radicals: An Application to the Synthesis of the Core Nucleus of FR-900482". The Journal of Organic Chemistry. 62 (4): 1083–1094. doi:10.1021/jo961992n.
- ↑ Hu, Pengfei; Snyder, Scott A. (29 March 2017). "Enantiospecific Total Synthesis of the Highly Strained (−)-Presilphiperfolan-8-ol via a Pd-Catalyzed Tandem Cyclization". Journal of the American Chemical Society. 139 (14): 5007–5010. doi:10.1021/jacs.7b01454.
- ↑ Gutmann, Bernhard; Elsner, Petteri; Glasnov, Toma; Roberge, Dominique M.; Kappe, C. Oliver (20 October 2014). "Shifting Chemical Equilibria in Flow-Efficient Decarbonylation Driven by Annular Flow Regimes". Angewandte Chemie International Edition. 53 (43): 11557–11561. doi:10.1002/anie.201407219.
- ↑ Kreis, Michael; Palmelund, Anders; Bunch, Lennart; Madsen, Robert (October 2006). "A General and Convenient Method for the Rhodium-Catalyzed Decarbonylation of Aldehydes". Advanced Synthesis & Catalysis. 348 (15): 2148–2154. doi:10.1002/adsc.200600228.