Topologically associating domain
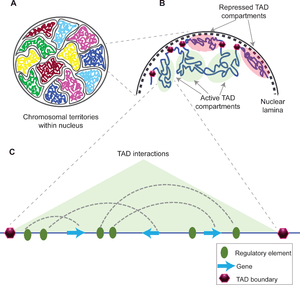
Topologically associating domains (TADs) are genomic regions ("chromosome neighborhoods") used to summarize the three-dimensional nuclear organization of mammalian genomes. A TAD represents a region of DNA within which physical interactions occur relatively frequently, whereas interactions across a TAD boundary occur relatively infrequently.[1] TADs can range in size from thousands to millions of DNA bases.
The mechanisms behind TAD formation are complex and not yet fully elucidated, though a number of proteins are known to be associated with TAD formation: for example, the proteins CTCF and cohesin.[1] Similarly, it is also unknown what components are required at TAD boundaries. However, it has been shown that these boundary regions have comparatively high levels of CTCF binding. In addition, some types of genes (such as transfer RNA genes and housekeeping genes) appear near TAD boundaries more often than would be expected by chance.[2][3]
Discovery and definition
TADs are defined as regions whose DNA sequences preferentially contact each other. They have been found using data gathered by the Hi-C technique.[4] They have been shown to be present in fruit flies (Drosophila),[5] mouse[6] and human[2] genomes, but not in the wine yeast Saccharomyces cerevisiae.[1]
TAD calling
To determine TAD locations, or "call TADs", an algorithm is applied to Hi-C data.
TADs are often called according to the method in Dixon et al. (2012), using the so-called "directionality index".[2] The directionality index is calculated for individual 40kb bins, by collecting the reads that fall in the bin, and observing whether their paired reads map upstream or downstream of the bin (read pairs are required to span no more than 2Mb). A positive value indicates that more read pairs lie downstream than upstream, and a negative value indicates the reverse. Mathematically, the directionality index is a signed chi-square statistic.
Properties of TADs
Conservation
TADs have been reported to be relatively constant between different cell types (in stem cells and blood cells, for example), and even between species in specific cases.[7]
Relationship with promoter-enhancer contacts
The majority of observed interactions between promoters and enhancers do not cross TAD boundaries. Removing a TAD boundary (for example, using CRISPR to delete the relevant region of the genome) can allow new promoter-enhancer contacts to form. This can affect gene expression nearby - such misregulation has been shown to cause limb malformations (e.g. polydactyly) in humans and mice.[7]
Relationship with other structural features of the genome
TADs have been reported to be the same as replication domains, regions of the genome that are copied (replicated) at the same time during S phase of cell division.[8] Insulated neighborhoods, DNA loops formed by CTCF/cohesin-bound regions, are proposed to functionally underlie TADs.[9]
Role in disease
Disruption of TAD boundaries can affect the expression of nearby genes, and this can cause disease.[10]
For example, genomic structural variants that disrupt TAD boundaries have been reported to cause developmental disorders such as human limb malformations.[11] Additionally, it has been claimed that disruption of TAD boundaries can provide growth advantages to certain cancers, such as T-cell acute lymphoblastic leukemia(T-ALL),[12] gliomas,[13] and colorectal cancer.[14]
Lamina-associated domains
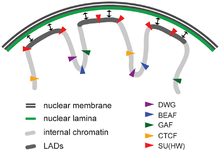
Lamina-associated domains (LADs) are parts of the chromatin that heavily interact with lamina, a network-like structure at the inner membrane of the nucleus.[15] LADs consist mostly of transcriptionally silent chromatin, being enriched with trimethylated Lys27 on histone H3, which is a common posttranslational histone modification of heterochromatin.[16] LADs have CTCF-binding sites at their periphery.[15]
See also
References
- 1 2 3 Pombo, A; Dillon, N (April 2015). "Three-dimensional genome architecture: players and mechanisms.". Nature Reviews Molecular Cell Biology. 16 (4): 245–57. PMID 25757416. doi:10.1038/nrm3965.
- 1 2 3 Dixon, J. R.; Selvaraj, S; Yue, F; Kim, A; Li, Y; Shen, Y; Hu, M; Liu, J. S.; Ren, B (2012). "Topological domains in mammalian genomes identified by analysis of chromatin interactions". Nature. 485 (7398): 376–80. PMC 3356448
. PMID 22495300. doi:10.1038/nature11082.
- ↑ Nora, EP; Lajoie, BR; Schulz, EG; Giorgetti, L; Okamoto, I; Servant, N; Piolot, T; van Berkum, NL; Meisig, J; Sedat, J; Gribnau, J; Barillot, E; Blüthgen, N; Dekker, J; Heard, Edith (2012). "Spatial partitioning of the regulatory landscape of the X-inactivation centre". Nature. 485 (7398): 381–5. PMC 3555144
. PMID 22495304. doi:10.1038/nature11049.
- ↑ de Laat, Wouter; Duboule, Denis (23 October 2013). "Topology of mammalian developmental enhancers and their regulatory landscapes". Nature. 502 (7472): 499–506. PMID 24153303. doi:10.1038/nature12753.
- ↑ Sexton, Tom; Yaffe, Eitan; Kenigsberg, Ephraim; Bantignies, Frédéric; Leblanc, Benjamin; Hoichman, Michael; Parrinello, Hugues; Tanay, Amos; Cavalli, Giacomo (February 2012). "Three-Dimensional Folding and Functional Organization Principles of the Drosophila Genome". Cell. 148 (3): 458–472. PMID 22265598. doi:10.1016/j.cell.2012.01.010.
- ↑ Nora, Elphège P.; Lajoie, Bryan R.; Schulz, Edda G.; Giorgetti, Luca; Okamoto, Ikuhiro; Servant, Nicolas; Piolot, Tristan; van Berkum, Nynke L.; Meisig, Johannes; Sedat, John; Gribnau, Joost; Barillot, Emmanuel; Blüthgen, Nils; Dekker, Job; Heard, Edith (11 April 2012). "Spatial partitioning of the regulatory landscape of the X-inactivation centre". Nature. 485 (7398): 381–385. PMC 3555144
. PMID 22495304. doi:10.1038/nature11049.
- 1 2 Jost, Daniel; Vaillant, Cédric; Meister, Peter (February 2017). "Coupling 1D modifications and 3D nuclear organization: data, models and function". Current Opinion in Cell Biology. 44: 20–27. doi:10.1016/j.ceb.2016.12.001.
- ↑ Pope, B. D.; Ryba, T; Dileep, V; Yue, F; Wu, W; Denas, O; Vera, D. L.; Wang, Y; Hansen, R. S.; Canfield, T. K.; Thurman, R. E.; Cheng, Y; Gülsoy, G; Dennis, J. H.; Snyder, M. P.; Stamatoyannopoulos, J. A.; Taylor, J; Hardison, R. C.; Kahveci, T; Ren, B; Gilbert, D. M. (2014). "Topologically associating domains are stable units of replication-timing regulation". Nature. 515 (7527): 402–5. PMC 4251741
. PMID 25409831. doi:10.1038/nature13986.
- ↑ Ji, X; Dadon, DB; Powell, BE; Fan, ZP; Borges-Rivera, D; Shachar, S; Weintraub, AS; Hnisz, D; Pegoraro, G; Lee, TI; Misteli, T; Jaenisch, R; Young, RA (4 February 2016). "3D Chromosome Regulatory Landscape of Human Pluripotent Cells.". Cell stem cell. 18 (2): 262–75. PMID 26686465. doi:10.1016/j.stem.2015.11.007.
- ↑ Lupiáñez, Darío G.; Spielmann, Malte; Mundlos, Stefan (April 2016). "Breaking TADs: How Alterations of Chromatin Domains Result in Disease". Trends in Genetics. 32 (4): 225–237. PMID 26862051. doi:10.1016/j.tig.2016.01.003.
- ↑ Lupiáñez, D. G.; Kraft, K; Heinrich, V; Krawitz, P; Brancati, F; Klopocki, E; Horn, D; Kayserili, H; Opitz, J. M.; Laxova, R; Santos-Simarro, F; Gilbert-Dussardier, B; Wittler, L; Borschiwer, M; Haas, S. A.; Osterwalder, M; Franke, M; Timmermann, B; Hecht, J; Spielmann, M; Visel, A; Mundlos, S (2015). "Disruptions of Topological Chromatin Domains Cause Pathogenic Rewiring of Gene-Enhancer Interactions". Cell. 161 (5): 1012–1025. PMC 4791538
. PMID 25959774. doi:10.1016/j.cell.2015.04.004.
- ↑ Hnisz, Denes; Weintraub, Abraham S.; Day, Daniel S.; Valton, Anne-Laure; Bak, Rasmus O.; Li, Charles H.; Goldmann, Johanna; Lajoie, Bryan R.; Fan, Zi Peng (2016-03-03). "Activation of proto-oncogenes by disruption of chromosome neighborhoods". Science. 351: aad9024. ISSN 0036-8075. PMID 26940867. doi:10.1126/science.aad9024.
- ↑ Flavahan, William A.; Drier, Yotam; Liau, Brian B.; Gillespie, Shawn M.; Venteicher, Andrew S.; Stemmer-Rachamimov, Anat O.; Suvà, Mario L.; Bernstein, Bradley E. (2016-01-07). "Insulator dysfunction and oncogene activation in IDH mutant gliomas". Nature. 529 (7584): 110–114. ISSN 0028-0836. PMC 4831574
. PMID 26700815. doi:10.1038/nature16490.
- ↑ Weischenfeldt, Joachim; Dubash, Taronish; Drainas, Alexandros P.; Mardin, Balca R.; Chen, Yuanyuan; Stütz, Adrian M.; Waszak, Sebsatian M.; Bosco, Graziella; Halvorsen, Ann R.; Raeder, Benjamin; Efthymiopoulos, Theocharis; Erkek, Serap; Siegl, Christine; Brenner, Hermann; Brustugun, Odd T.; Dieter, Sebastian M; Northcott, Paul A.; Petersen, Iver; Pfister, Stefan M.; Schneider, Martin; Solberg, Steinar K.; Thunissen, Erik; Weichert, Wilko; Zichner, Thomas; Thomas, Roman; Peifer, Martin; Helland, Aslaug; Ball, Claudia R.; Jechlinger, Martin; Sotillo, Rocio; Glimm, Hanno; Korbel, Jan O. (2016). "Pan-cancer analysis of somatic copy-number alterations implicates IRS4 and IGF2 in enhancer hijacking". Nature Genetics. 49: 65–74. PMID 27869826. doi:10.1038/ng.3722.
- 1 2 Gonzalez-Sandoval, Adriana; Gasser, Susan M. (August 2016). "On TADs and LADs: Spatial Control Over Gene Expression". Trends in genetics: TIG. 32 (8): 485–495. ISSN 0168-9525. PMID 27312344. doi:10.1016/j.tig.2016.05.004.
- ↑ Li, Mo; Liu, Guang-Hui; Izpisua Belmonte, Juan Carlos (2012-07-23). "Navigating the epigenetic landscape of pluripotent stem cells". Nature Reviews. Molecular Cell Biology. 13 (8): 524–535. ISSN 1471-0080. PMID 22820889. doi:10.1038/nrm3393.