Elastography
Elastography | |
---|---|
Medical diagnostics | |
![]() Conventional ultrasonography (lower image) and elastography (supersonic shear imaging; upper image) of papillary thyroid carcinoma, a malignant cancer. The cancer (red) is much stiffer than the healthy tissue. | |
MeSH | D054459 |
Elastography is a medical imaging modality that maps the elastic properties and stiffness of soft tissue.[1][2] The main idea is that whether the tissue is hard or soft will give diagnostic information about the presence or status of disease. For example, cancerous tumours will often be harder than the surrounding tissue, and diseased livers are stiffer than healthy ones.[1][2][3][4]
The most prominent techniques use ultrasound or magnetic resonance imaging (MRI) to make both the stiffness map and an anatomical image for comparison. Tactile imaging composed from acquired stress-strain data reveals elasticity and anatomical features.
Applications
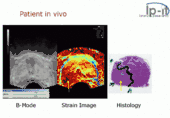
Elastography is used for the investigation of many disease conditions in many organs. It can be used for additional diagnostic information compared to a mere anatomical image, and it can be used to guide biopsies or, increasingly, replace them entirely. Biopsies are invasive and painful, presenting a risk of infection, whereas elastography is completely noninvasive.
Elastography is used to investigate disease in the liver. Liver stiffness is usually indicative of fibrosis or steatosis, which are in turn indicative of numerous disease conditions, including cirrhosis and hepatitis. Elastography is particularly advantageous in this case because when fibrosis is diffuse, a biopsy can easily miss sampling the diseased tissue, which results in a false negative misdiagnosis.
Naturally, elastography sees use for organs and diseases where manual palpation was already widespread. Elastography is used for detection and diagnosis of breast, thyroid and prostate cancers. Certain types of elastography are also suitable for musculoskeletal imaging, and they can determine the mechanical properties and state of muscles and tendons.
Because elastography does not have the same limitations as manual palpation, it is being investigated in some areas for which there is no history of diagnosis with manual palpation. For example, magnetic resonance elastography is capable of assessing the stiffness of the brain, and there is a growing body of scientific literature on elastography in healthy and diseased brains.
Historical Background
_(3).jpg)
Palpation is the practice of feeling the stiffness of a patient's tissues with the practitioner's hands. Manual palpation dates back at least to 1500 BC, with the Egyptian Ebers Papyrus and Edwin Smith Papyrus both giving instructions on diagnosis with palpation. In Ancient Greece, Hippocrates gave instructions on many forms of diagnosis using palpation, including palpation of the breasts, wounds, bowels, ulcers, uterus, skin and tumours. In the modern Western world, palpation became considered a respectable method of diagnosis in the 1930s.[1] Since then, the practice of palpation has become widespread, and it is considered an effective method of detecting tumours and other pathologies.
Manual palpation, however, suffers from several important limitations: it is limited to tissues accessible to the physician's hand, it is distorted by any intervening tissue, and it is qualitative but not quanitative. Elastography, the measurement of tissue stiffness, seeks to address these challenges. Tactile imaging, also called "Mechanical imaging", "Stress imaging" or "Computerized palpation", is a medical imaging modality that translates the sense of touch into a digital image.
How it works
There are numerous elastographic techniques, in development stages from early research to extensive clinical application. Each of these techniques works in a different way. What all methods have in common is that they create a distortion in the tissue,† observe and process the tissue response to infer the mechanical properties of the tissue, and then display the results to the operator, usually as an image. Each elastographic method is characterized by the way it does each of these things.
Inducing a distortion
To image the mechanical properties of tissue, we need to see how it behaves when deformed. There are three main ways of inducing a distortion to observe. These are:
- Pushing/deforming or vibrating the surface of the body (skin) or organ (prostate) with a probe or a tool,
- Using radiation force of focused ultrasound to remotely create a 'push' inside the tissue, and
- Using distortions created by normal physiological processes, e.g. pulse or heartbeat.
Observing the response
The primary way elastographic techniques are categorized is by what imaging modality (type) they use to observe the response. Elastographic techniques use ultrasound, magnetic resonance imaging (MRI) and pressure/stress sensors in tactile imaging (TI). There are a handful of other methods that exist as well.
The observation of the tissue response can take many forms. In terms of the image obtained, it can be 1-D (i.e. a line), 2-D (a plane) or 3-D (a volume), or just a single value, and it can either be a video or a single image. In most cases, the result is displayed to the operator along with a conventional image of the tissue, which shows where in the tissue the different stiffness values occur.
Processing and presentation
Once the response has been observed, the stiffness can be calculated from it. Most elastography techniques find the stiffness of tissue based on one of two main principles:
- For a given applied force (stress), stiffer tissue deforms (strains) less than does softer tissue.
- Mechanical waves (specifically shear waves) travel faster through stiffer tissue than through softer tissue.
Some techniques will simply display the distortion and/or response, or the wave speed to the operator, while others will compute the stiffness (specifically the Young's modulus or similar shear modulus) and display that instead. Some techniques present results quantitatively, while others only present qualitative (relative) results.
Ultrasound Elastography
There are great many ultrasound elastographic techniques. The most prominent are highlighted below.
Quasistatic Elastography / Strain Imaging

Quasistatic elastography (sometimes called simply 'elastography' for historical reasons) is a pioneering elastography technique. In this technique, an external compression is applied to tissue, and the ultrasound images before and after the compression are compared. The areas of the image that are least deformed are the ones that are the stiffest, while the most deformed areas are the least stiff.[3] Generally, what is displayed to the operator is an image of the relative distortions (strains), which is often of clinical utility.[1]
From the relative distortion image, however, making a quantitative stiffness map is often desired. To do this requires that assumptions be made about the nature of the soft tissue being imaged and about tissue outside of the image. Additionally, under compression, objects can move into or out of the image or around in the image, causing problems with interpretation. Another limit of this technique is that like manual palpation, it has difficulty with organs or tissues that are not close to the surface or easily compressed.[4]
Acoustic Radiation Force Impulse imaging (ARFI)

Acoustic Radiation Force Impulse Imaging (ARFI)[5] uses ultrasound to create a qualitative 2-D map of tissue stiffness. It does so by creating a 'push' inside the tissue using the acoustic radiation force from a focused ultrasound beam. The amount the tissue along the axis of the beam is pushed down is reflective of tissue stiffness; softer tissue is more easily pushed than stiffer tissue. ARFI shows a qualitative stiffness value along the axis of the pushing beam. By pushing in many different places, a map of the tissue stiffness is built up.
Shear Wave Elasticity Imaging (SWEI)
In Shear Wave Elasticity Imaging (SWEI),[6] similar to ARFI, a 'push' is induced deep in the tissue by acoustic radiation force. The disturbance created by this push travels sideways through the tissue as a shear wave. By using an image modality like ultrasound or MRI to see how fast the wave gets to different lateral positions, the stiffness of the intervening tissue is inferred. Since the terms “Elasticity Imaging” and "Elastography" are synonyms, the original term SWEI denoting the technology for elasticity mapping using shear waves is often replaced by SWE. Principal difference between SWEI and ARFI is that SWEI is based on the use of shear waves propagating laterally from the beam axis and creating elasticity map by measuring shear wave propagation parameters whereas ARFI gets elasticity information from the axis of the pushing beam and uses multiple pushes to create a 2-D stiffness map. No shear waves are involved in ARFI and no axial elasticity assessment is involved in SWEI. SWEI is implemented in the Supersonic Shear Imaging (SSI), one of the most advanced modalities of ultrasound elastography.
Supersonic Shear Imaging (SSI)
_supersonic_shear_imaging_of_hand_muscle_stiffness.tif.jpg)
Supersonic Shear Imaging (SSI)[7][8] gives a quantitative, real-time two-dimensional map of tissue stiffness. SSI is based on SWEI: it uses acoustic radiation force to induce a 'push' inside the tissue of interest generating shear waves and the tissue's stiffness is computed from how fast the resulting shear wave travels through the tissue. Local tissue velocity maps are obtained with a conventional speckle tracking technique and provide a full movie of the shear wave propagation through the tissue. There are two principal innovations implemented in SSI. First, by using many near-simultaneous pushes, SSI creates a source of shear waves which is moved through the medium at a supersonic speed. Second, the generated shear wave is visualized by using ultrafast imaging technique. Using inversion algorithms, the shear elasticity of medium is mapped quantitatively from the wave propagation movie. SSI is the first ultrasonic imaging technology able to reach more than 10,000 frames per second of deep seated organs. SSI provides a set of quantitative and in vivo parameters describing the tissue mechanical properties: Young’s modulus, viscosity, anisotropy.
This approach demonstrated clinical benefit in breast, thyroid, liver, prostate and musculoskeletal imaging. SSI is used for breast examination with a number of high-resolution linear transducers.[9] A large multi-center breast imaging study has demonstrated both reproducibility [10] and significant improvement in the classification[11] of breast lesions when shear wave elastography images are added to the interpretation of standard B-mode and Color mode ultrasound images.
Transient Elastography
Transient elastography gives a quantitative one-dimensional (i.e. a line) image of tissue stiffness. It functions by vibrating the skin with a motor to create a passing distortion in the tissue (a shear wave), and imaging the motion of that distortion as it passes deeper into the body using a 1D ultrasound beam. It then displays a quantitative line of tissue stiffness data (the Young's modulus).[12][13] This technique is used mainly by the FibroScan system, which is used for liver assessment,[14] for example, to diagnose cirrhosis.
Magnetic Resonance Elastography (MRE)

Magnetic resonance elastography (MRE) [15] was introduced in the mid-1990s, and multiple clinical applications have been investigated. In MRE, a mechanical vibrator is used on the surface of the patient's body; this creates shear waves that travel into the patient's deeper tissues. An imaging acquisition sequence that measures the velocity of the waves is used, and this is used to infer the tissue's stiffness (the shear modulus).[16][17] The result of an MRE scan is a quantitative 3-D map of the tissue stiffness, as well as a conventional 3-D MRI image.
One strength of MRE is the resulting 3D elasticity map, which can cover an entire organ.[2] Because MRI is not limited by air or bone, it can access some tissues ultrasound cannot, notably the brain. It also has the advantage of being more uniform across operators and less dependent on operator skill than most methods of ultrasound elastography.
MR elastography has made significant advances over the past few years with acquisition times down to a minute or less and has been used in a variety of medical applications including cardiology research on living human hearts. MR elastography's short acquisition time also make it competitive with other elastography techniques.
Tactile Imaging (TI)

Tactile Imaging is a medical imaging modality that translates the sense of touch into a digital image. The tactile image is a function of P(x,y,z), where P is the pressure on soft tissue surface under applied deformation and x,y,z are coordinates where pressure P was measured. Tactile imaging closely mimics manual palpation, since the probe of the device with a pressure sensor array mounted on its face acts similar to human fingers during clinical examination, deforming soft tissue by the probe and detecting resulting changes in the pressure pattern. Clinical applications include imaging of the prostate,[18][19] breast,[20][21] elasticity assessment of vagina and pelvic floor support structures,[22] muscle functional imaging of the female pelvic floor [23] and myofascial trigger points in muscle.[24]
Mechanical imaging, as a modality of medical diagnostics using mechanical sensors, was introduced in mid 1990s.[25][26] Many physical principles have been explored for the realization of tactile sensors: resistive, inductive, capacitive, optoelectric, magnetic, piezoelectric and electroacoustic principles, in a variety of configurations.[27][28][29]
Other Techniques
These include elastography with optical coherence tomography [30] (i.e. light).
![]() |
Wikimedia Commons has media related to Elastography. |
Notes
- †^ In the case of endogenous motion imaging, instead of inducing a disturbance, disturbances naturally created by physiological processes are observed.
References
- 1 2 3 4 Wells, P. N. T. (June 2011). "Medical ultrasound: imaging of soft tissue strain and elasticity". Journal of the Royal Society, Interface. 8 (64): 1521–1549. doi:10.1098/rsif.2011.0054.
- 1 2 3 Sarvazyan A, Hall TJ, Urban MW, Fatemi M, Aglyamov SR, Garra BS. Overview of elastography–an emerging branch of medical imaging. Current Medical Imaging Reviews, 2011, 7(4):255-282.
- 1 2 Ophir, J.; Céspides, I.; Ponnekanti, H.; Li, X. (April 1991). "Elastography: A quantitative method for imaging the elasticity of biological tissues". Ultrasonic Imaging. 13 (2): 111–134. PMID 1858217. doi:10.1016/0161-7346(91)90079-W.
- 1 2 Parker, K J; Doyley, M M; Rubens, D J (February 2011). "Imaging the elastic properties of tissue: the 20 year perspective". Physics in Medicine and Biology. 56 (2): 513. doi:10.1088/0031-9155/57/16/5359.
- ↑ Nightingale KR, Palmeri ML, Nightingale RW, and Trahey GE, On the feasibility of remote palpation using acoustic radiation force. J. Acoust. Soc. Am. 2001; 110: 625-34
- ↑ Sarvazyan AP, Rudenko OV, Swanson SD, Fowlkes JB, Emelianov SY. Shear wave elasticity imaging: a new ultrasonic technology of medical diagnostics. Ultrasound Med Biol. 1998; 24(9): 1419-35.
- ↑ Supersonic Shear Imaging: A New Technique for Soft Tissue Elasticity Mapping. Bercoff J. et al., IEEE Transactions on Ultrasonics, Ferroelectrics, and Frequency Control, Vol. 51, No. 4, April 2004.
- ↑ Acoustoelasticity in soft solids: Assessment of the nonlinear shear modulus with the acoustic radiation force, J.-L. Gennisson,a M. Rénier, S. Catheline, C. Barrière, J. Bercoff, M. Tanter, and M. Fink, J. Acoust. Soc. Am. 122 [1]6, December 2007
- ↑ Mendelson EB, Chen J, Karstaedt P. Assessing tissue stiffness may boost breast imaging specificity. Diagnostic Imaging. 2009;31(12):15-17.
- ↑ Shear wave elastography for breast masses is highly reproducible. Cosgrove DO, Berg WA, Doré CJ, Skyba DM, Henry JP, Gay J, Cohen-Bacrie C; the BE1 Study Group. Eur Radiol. 2011 Dec 31.
- ↑ Shear-wave Elastography Improves the Specificity of Breast US: The BE1 Multinational Study of 939 Masses. Berg WA, Cosgrove DO, Doré CJ, Schäfer FKW, Svensson WE, Hooley RJ, Ohlinger R, Mendelson EB, Balu-Maestro C, Locatelli M, Tourasse C, Cavanaugh BC, Juhan V, Stavros AT, Tardivon A, Gay J, Henry JP, Cohen-Bacrie C, and the BE1 Investigators. Radiology 2012;262:435-449
- ↑ Catheline, Stefan; Wu, Francois; Fink, Mathias (1999). "A solution to diffraction biases in sonoelasticity: The acoustic impulse technique.". Journal of the Acoustical Society of America. 105 (5): 2941–2950. doi:10.1109/58.996561.
- ↑ Sandrin, Laurent; Tanter, Mickaël; Gennisson, Jean-Luc; Catheline, Stefan; Fink, Mathias (April 2002). "Shear elasticity probe for soft tissues with 1-D transient elastography.". IEEE transactions on ultrasonics, ferroelectrics, and frequency control. 49 (4): 436–446. doi:10.1109/58.996561.
- ↑ Ganne-Carrié N; Ziol M; de Ledinghen V; et al. (2006). "Accuracy of liver stiffness measurement for the diagnosis of cirrhosis in patients with chronic liver diseases". Hepatology. 44 (6): 1511–7. PMID 17133503. doi:10.1002/hep.21420.
- ↑ Sarvazyan AP, Skovoroda AR, Emelianov SY, Fowlkes JB, Pipe JG, Adler RS, Buxton RB, Carson PL. Biophysical bases of elasticity imaging. In: Acoustical Imaging. Ed. Jones JP, Plenum Press, New York and London, 1995; 21: 223-240.
- ↑ Muthupillai R, Lomas DJ, Rossman PJ, et al. Magnetic resonance elastography by direct visualization of propagating acoustic strain waves. Science 1995; 269: 1854-7.[49, 219, 220].
- ↑ Manduca A, Oliphant TE, Dresner MA, et al. Magnetic resonance elastography: Non-invasive mapping of tissue elasticity. Med Image Anal 2001; 5: 237-54.
- ↑ Egorov V, Ayrapetyan S, Sarvazyan AP. Prostate Mechanical Imaging: 3-D image composition and feature calculations. IEEE Trans Med Imaging 2006; 25(10): 1329-40.
- ↑ Weiss RE, Egorov V, Ayrapetyan S, Sarvazyan N, Sarvazyan A. Prostate mechanical imaging: a new method for prostate assessment. Urology 2008; 71(3):425-429.
- ↑ Egorov V, Sarvazyan AP. Mechanical Imaging of the Breast. IEEE Transactions on Medical Imaging 2008; 27(9):1275-87.
- ↑ Egorov V, Kearney T, Pollak SB, Rohatgi C, Sarvazyan N, Airapetian S, Browning S, Sarvazyan A. Differentiation of benign and malignant breast lesions by mechanical imaging. Breast Cancer Research and Treatment 2009; 118(1): 67-80.
- ↑ Egorov V, van Raalte H, Sarvazyan A. Vaginal Tactile Imaging. IEEE Transactions on Biomedical Engineering 2010; 57(7):1736-44.
- ↑ van Raalte H, Egorov V. Characterizing female pelvic floor conditions by tactile imaging. International Urogynecology Journal 2015; 26(4): 6097-7, with Video Supplement.
- ↑ Turo D, Otto P, Egorov V, Sarvazyan A, Gerber LH, Sikdar S. Elastography and tactile imaging for mechanical characterization of superficial muscles. J Acoust Soc Am 2012; 132(3):1983.
- ↑ Sarvazyan A (April 1998). "Mechanical imaging: a new technology for medical diagnostics". International Journal of Medical Informatics. 49 (2): 195–216. PMID 9741894. doi:10.1016/S1386-5056(98)00040-9.
- ↑ Sarvazyan AP, Skovoroda AR. June 1996. Method and apparatus for elasticity imaging. U.S. Patent 5,524,636; 1996.
- ↑ Regtien, PPL (1992). "Tactile imaging". Sensors and Actuators A: Physical. 31 (1–3): 83–9. doi:10.1016/0924-4247(92)80084-G.
- ↑ Galea, AM (2004). Mapping tactile imaging information: parameter estimation and deformable registration (PhD thesis). Cambridge, MA: Harvard University's Division of Engineering and Applied Sciences. pp. 1–235. OCLC 70682744.
- ↑ Tegin, J; Wikander, J (2005). "Tactile sensing in intelligent robotic manipulation – a review". Industrial Robot. 32 (1): 64–70. doi:10.1108/01439910510573318.
- ↑ Kennedy BF, Kennedy KM, Sampson DD. A review of optical coherence elastography: fundamentals, techniques and prospects. IEEE Journal of Selected Topics in Quantum Electronics 2014; 20(2):7101217.