Rings of Saturn

The rings of Saturn are the most extensive planetary ring system of any planet in the Solar System. They consist of countless small particles, ranging from μm to m in size,[1] that orbit about Saturn. The ring particles are made almost entirely of water ice, with a trace component of rocky material. There is still no consensus as to their mechanism of formation; some features of the rings suggest a relatively recent origin, but theoretical models indicate they are likely to have formed early in the Solar System's history.[2]
Although reflection from the rings increases Saturn's brightness, they are not visible from Earth with unaided vision. In 1610, the year after Galileo Galilei turned a telescope to the sky, he became the first person to observe Saturn's rings, though he could not see them well enough to discern their true nature. In 1655, Christiaan Huygens was the first person to describe them as a disk surrounding Saturn.[3] Although many people think of Saturn's rings as being made up of a series of tiny ringlets (a concept that goes back to Laplace),[3] true gaps are few. It is more correct to think of the rings as an annular disk with concentric local maxima and minima in density and brightness.[2] On the scale of the clumps within the rings there is much empty space.
The rings have numerous gaps where particle density drops sharply: two opened by known moons embedded within them, and many others at locations of known destabilizing orbital resonances with Saturn's moons. Other gaps remain unexplained. Stabilizing resonances, on the other hand, are responsible for the longevity of several rings, such as the Titan Ringlet and the G Ring.
Well beyond the main rings is the Phoebe ring, which is tilted at an angle of 27 degrees to the other rings and, like Phoebe, orbits in retrograde fashion.
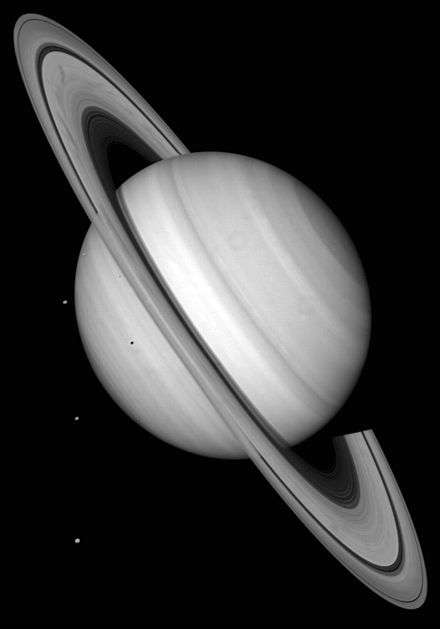
History
Galileo's work

Galileo Galilei was the first to observe the rings of Saturn in 1610 using his telescope, but was unable to identify them as such. He wrote to the Duke of Tuscany that "The planet Saturn is not alone, but is composed of three, which almost touch one another and never move nor change with respect to one another. They are arranged in a line parallel to the zodiac, and the middle one (Saturn itself) is about three times the size of the lateral ones."[4] He also described the rings as Saturn's "ears". In 1612 the Earth passed through the plane of the rings and they became invisible. Mystified, Galileo remarked "I do not know what to say in a case so surprising, so unlooked for and so novel."[3] He mused, "Has Saturn swallowed his children?" — referring to the myth of the Titan Saturn devouring his offspring to forestall the prophecy of them overthrowing him.[4] He was further confused when the rings again became visible in 1613.[3]
Early astronomers used anagrams as a form of commitment scheme to lay claim to new discoveries before their results were ready for publication. Galileo used smaismrmilmepoetaleumibunenugttauiras for Altissimum planetam tergeminum observavi ("I have observed the most distant planet to have a triple form") for discovering the rings of Saturn.[5]
Ring theory, observations and exploration
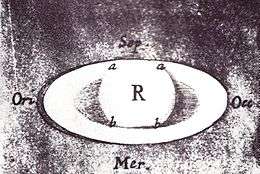
In 1655, Christiaan Huygens became the first person to suggest that Saturn was surrounded by a ring. Using a 50× power refracting telescope that he designed himself, far superior to those available to Galileo, Huygens observed Saturn and wrote that "It [Saturn] is surrounded by a thin, flat, ring, nowhere touching, inclined to the ecliptic".[3] Robert Hooke was another early observer of the rings of Saturn, and noted the casting of shadows on the rings.[6]
In 1675, Giovanni Domenico Cassini determined that Saturn's ring was composed of multiple smaller rings with gaps between them; the largest of these gaps was later named the Cassini Division. This division is a 4,800-km-wide region between the A Ring and B Ring.[7]
In 1787, Pierre-Simon Laplace proved that a uniform solid ring would be unstable and suggested that the rings were composed of a large number of solid ringlets.[3][8]
In 1859, James Clerk Maxwell demonstrated that a nonuniform solid ring, solid ringlets or a continuous fluid ring would also not be stable, indicating that the ring must be composed of numerous small particles, all independently orbiting Saturn.[8] Later, Sofia Kovalevskaya also found that Saturn's rings cannot be liquid ring-shaped bodies.[9] Spectroscopic studies of the rings carried out in 1895 by James Keeler of Allegheny Observatory and Aristarkh Belopolsky of Pulkovo Observatory showed Maxwell's analysis was correct.
Four robotic spacecraft have observed Saturn's rings from the vicinity of the planet. Pioneer 11's closest approach to Saturn occurred in September 1979 at a distance of 20,900 km.[10] Pioneer 11 was responsible for the discovery of the F ring.[10] Voyager 1's closest approach occurred in November 1980 at a distance of 64,200 km.[11] A failed photopolarimeter prevented Voyager 1 from observing Saturn's rings at the planned resolution; nevertheless, images from the spacecraft provided unprecedented detail of the ring system and revealed the existence of the G ring.[12] Voyager 2's closest approach occurred in August 1981 at a distance of 41,000 km.[11] Voyager 2's working photopolarimeter allowed it to observe the ring system at higher resolution than Voyager 1, and to thereby discover many previously unseen ringlets.[13] Cassini entered into orbit around Saturn in July 2004.[14] Cassini's images of the rings are the most detailed to-date, and are responsible for the discovery of yet more ringlets.[15]
The rings are named alphabetically in the order they were discovered.[16] The main rings are, working outward from the planet, C, B and A, with the Cassini Division, the largest gap, separating Rings B and A. Several fainter rings were discovered more recently. The D Ring is exceedingly faint and closest to the planet. The narrow F Ring is just outside the A Ring. Beyond that are two far fainter rings named G and E. The rings show a tremendous amount of structure on all scales, some related to perturbations by Saturn's moons, but much unexplained.[16]
Physical characteristics


The dense main rings extend from 7,000 km (4,300 mi) to 80,000 km (50,000 mi) away from Saturn's equator, whose radius is 60,300 km (37,500 mi) (see Major subdivisions). With an estimated local thickness of as little as 10 m[17] and as much as 1 km,[18] they are composed of 99.9% pure water ice with a smattering of impurities that may include tholins or silicates.[19] The main rings are primarily composed of particles ranging in size from 1 cm to 10 m.[20]
Based on Voyager observations, the total mass of the rings was estimated to be about 3 x 1019 kg. This is a small fraction of the total mass of Saturn (about 50 ppb) and is just a little less than the moon Mimas.[21] More recent observations and computer modeling based on Cassini observations show that this may be an underestimate due to clumping in the rings and the mass may be three times this figure.[22] Although the largest gaps in the rings, such as the Cassini Division and Encke Gap, can be seen from Earth, both Voyager spacecraft discovered that the rings have an intricate structure of thousands of thin gaps and ringlets. This structure is thought to arise, in several different ways, from the gravitational pull of Saturn's many moons. Some gaps are cleared out by the passage of tiny moonlets such as Pan,[23] many more of which may yet be discovered, and some ringlets seem to be maintained by the gravitational effects of small shepherd satellites (similar to Prometheus and Pandora's maintenance of the F ring). Other gaps arise from resonances between the orbital period of particles in the gap and that of a more massive moon further out; Mimas maintains the Cassini Division in this manner.[24] Still more structure in the rings consists of spiral waves raised by the inner moons' periodic gravitational perturbations at less disruptive resonances. Data from the Cassini space probe indicate that the rings of Saturn possess their own atmosphere, independent of that of the planet itself. The atmosphere is composed of molecular oxygen gas (O2) produced when ultraviolet light from the Sun interacts with water ice in the rings. Chemical reactions between water molecule fragments and further ultraviolet stimulation create and eject, among other things, O2. According to models of this atmosphere, H2 is also present. The O2 and H2 atmospheres are so sparse that if the entire atmosphere were somehow condensed onto the rings, it would be about one atom thick.[25] The rings also have a similarly sparse OH (hydroxide) atmosphere. Like the O2, this atmosphere is produced by the disintegration of water molecules, though in this case the disintegration is done by energetic ions that bombard water molecules ejected by Saturn's moon Enceladus. This atmosphere, despite being extremely sparse, was detected from Earth by the Hubble Space Telescope.[26] Saturn shows complex patterns in its brightness.[27] Most of the variability is due to the changing aspect of the rings,[28][29] and this goes through two cycles every orbit. However, superimposed on this is variability due to the eccentricity of the planet's orbit that causes the planet to display brighter oppositions in the northern hemisphere than it does in the southern.[30]
In 1980, Voyager 1 made a fly-by of Saturn that showed the F-ring to be composed of three narrow rings that appeared to be braided in a complex structure; it is now known that the outer two rings consist of knobs, kinks and lumps that give the illusion of braiding, with the less bright third ring lying inside them.
New images of the rings taken around the 11 August 2009 equinox of Saturn by NASA's Cassini spacecraft have shown that the rings extend significantly out of the nominal ring plane in a few places. This displacement reaches as much as 4 km (2.5 mi) at the border of the Keeler Gap, due to the out-of-plane orbit of Daphnis, the moon that creates the gap.[31]
Formation of main rings
Saturn's rings may be very old, dating to the formation of Saturn itself. There are two main theories regarding the origin of Saturn's inner rings. One theory, originally proposed by Édouard Roche in the 19th century, is that the rings were once a moon of Saturn (named Veritas, a Roman goddess who hid in a well) whose orbit decayed until it came close enough to be ripped apart by tidal forces (see Roche limit).[32] A variation on this theory is that this moon disintegrated after being struck by a large comet or asteroid.[33] The second theory is that the rings were never part of a moon, but are instead left over from the original nebular material from which Saturn formed.
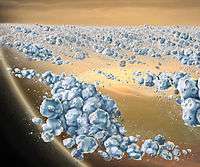
A more traditional version of the disrupted-moon theory is that the rings are composed of debris from a moon 400 to 600 km in diameter, slightly larger than Mimas. The last time there were collisions large enough to be likely to disrupt a moon that large was during the Late Heavy Bombardment some four billion years ago.[34]
A more recent variant of this type of theory by R. M. Canup is that the rings could represent part of the remains of the icy mantle of a much larger, Titan-sized, differentiated moon that was stripped of its outer layer as it spiraled into the planet during the formative period when Saturn was still surrounded by a gaseous nebula.[35][36] This would explain the scarcity of rocky material within the rings. The rings would initially have been much more massive (~1,000 times) and broader than at present; material in the outer portions of the rings would have coalesced into the moons of Saturn out to Tethys, also explaining the lack of rocky material in the composition of most of these moons.[36] Subsequent collisional or cryovolcanic evolution of Enceladus might then have caused selective loss of ice from this moon, raising its density to its current value of 1.61 g/cm3, compared to values of 1.15 for Mimas and 0.97 for Tethys.[36]
The idea of massive early rings was subsequently extended to explain the formation of Saturn's moons out to Rhea.[37] If the initial massive rings contained chunks of rocky material (>100 km across) as well as ice, these silicate bodies would have accreted more ice and been expelled from the rings, due to gravitational interactions with the rings and tidal interaction with Saturn, into progressively wider orbits. Within the Roche limit, bodies of rocky material are dense enough to accrete additional material, whereas less-dense bodies of ice are not. Once outside the rings, the newly formed moons could have continued to evolve through random mergers. This process may explain the variation in silicate content of Saturn's moons out to Rhea, as well as the trend towards less silicate content closer to Saturn. Rhea would then be the oldest of the moons formed from the primordial rings, with moons closer to Saturn being progressively younger.[37]
The brightness and purity of the water ice in Saturn's rings has been cited as evidence that the rings are much younger than Saturn, perhaps just 100 million years old, as the infall of meteoric dust would have led to darkening of the rings. However, new research indicates that the B Ring may be massive enough to have diluted infalling material and thus avoided substantial darkening over the age of the Solar System. Ring material may be recycled as clumps form within the rings and are then disrupted by impacts. This would explain the apparent youth of some of the material within the rings.[38] Further evidence supporting a young ring theory has been gathered by researchers analyzing data from the Cassini Titan Radar Mapper, which focused on analyzing the proportion of rocky silicates contained within the C ring.[39]
The Cassini UVIS team, led by Larry Esposito, used stellar occultation to discover 13 objects, ranging from 27 m to 10 km across, within the F ring. They are translucent, suggesting they are temporary aggregates of ice boulders a few m across. Esposito believes this to be the basic structure of the Saturnian rings, particles clumping together, then being blasted apart.[40]
Subdivisions and structures within the rings
The densest parts of the Saturnian ring system are the A and B Rings, which are separated by the Cassini Division (discovered in 1675 by Giovanni Domenico Cassini). Along with the C Ring, which was discovered in 1850 and is similar in character to the Cassini Division, these regions constitute the main rings. The main rings are denser and contain larger particles than the tenuous dusty rings. The latter include the D Ring, extending inward to Saturn's cloud tops, the G and E Rings and others beyond the main ring system. These diffuse rings are characterised as "dusty" because of the small size of their particles (often about a μm); their chemical composition is, like the main rings, almost entirely of water ice. The narrow F Ring, just off the outer edge of the A Ring, is more difficult to categorize; parts of it are very dense, but it also contains a great deal of dust-size particles.
Physical parameters of the rings
Notes:
(1) Names as designated by the International Astronomical Union, unless otherwise noted. Broader separations between named rings are termed divisions, while narrower separations within named rings are called gaps.
(2) Data mostly from the Gazetteer of Planetary Nomenclature, a NASA factsheet and several papers.[41][42][43]
(3) distance is to centre of gaps, rings and ringlets that are narrower than 1,000 km
(4) unofficial name
Major subdivisions
Name(1) | Distance from Saturn's center (km)(2) | Width (km)(2) | Named after |
---|---|---|---|
D Ring | 66,900 – 74,510 | 7,500 | |
C Ring | 74,658 – 92,000 | 17,500 | |
B Ring | 92,000 – 117,580 | 25,500 | |
Cassini Division | 117,580 – 122,170 | 4,700 | Giovanni Cassini |
A Ring | 122,170 – 136,775 | 14,600 | |
Roche Division | 136,775 – 139,380 | 2,600 | Édouard Roche |
F Ring | 140,180 (3) | 30 – 500 | |
Janus/Epimetheus Ring(4) | 149,000 – 154,000 | 5,000 | Janus and Epimetheus |
G Ring | 166,000 – 175,000 | 9,000 | |
Methone Ring Arc(4) | 194,230 | ? | Methone |
Anthe Ring Arc(4) | 197,665 | ? | Anthe |
Pallene Ring(4) | 211,000 – 213,500 | 2,500 | Pallene |
E Ring | 180,000 – 480,000 | 300,000 | |
Phoebe Ring | ~4,000,000 – >13,000,000 | Phoebe |
C Ring structures
Name(1) | Distance from Saturn's center (km)(2) | Width (km)(2) | Named after |
---|---|---|---|
Colombo Gap | 77,870 (3) | 150 | Giuseppe "Bepi" Colombo |
Titan Ringlet | 77,870 (3) | 25 | Titan, moon of Saturn |
Maxwell Gap | 87,491 (3) | 270 | James Clerk Maxwell |
Maxwell Ringlet | 87,491 (3) | 64 | James Clerk Maxwell |
Bond Gap | 88,700 (3) | 30 | William Cranch Bond and George Phillips Bond |
1.470RS Ringlet | 88,716 (3) | 16 | its radius |
1.495RS Ringlet | 90,171 (3) | 62 | its radius |
Dawes Gap | 90,210 (3) | 20 | William Rutter Dawes |
Cassini Division structures
- Source:[44]
Name(1) | Distance from Saturn's center (km)(2) | Width (km)(2) | Named after |
---|---|---|---|
Huygens Gap | 117,680 (3) | 285–400 | Christiaan Huygens |
Huygens Ringlet | 117,848 (3) | ~17 | Christiaan Huygens |
Herschel Gap | 118,234 (3) | 102 | William Herschel |
Russell Gap | 118,614 (3) | 33 | Henry Norris Russell |
Jeffreys Gap | 118,950 (3) | 38 | Harold Jeffreys |
Kuiper Gap | 119,405 (3) | 3 | Gerard Kuiper |
Laplace Gap | 119,967 (3) | 238 | Pierre-Simon Laplace |
Bessel Gap | 120,241 (3) | 10 | Friedrich Bessel |
Barnard Gap | 120,312 (3) | 13 | Edward Emerson Barnard |
A Ring structures
Name(1) | Distance from Saturn's center (km)(2) | Width (km)(2) | Named after |
---|---|---|---|
Encke Gap | 133,589 (3) | 325 | Johann Encke |
Keeler Gap | 136,505 (3) | 35 | James Keeler |
D Ring

The D Ring is the innermost ring, and is very faint. In 1980, Voyager 1 detected within this ring three ringlets designated D73, D72 and D68, with D68 being the discrete ringlet nearest to Saturn. Some 25 years later, Cassini images showed that D72 had become significantly broader and more diffuse, and had moved planetward by 200 km.[45]
Present in the D Ring is a finescale structure with waves 30 km apart. First seen in the gap between the C Ring and D73,[45] the structure was found during Saturn's 2009 equinox to extend a radial distance of 19,000 km from the D Ring to the inner edge of the B Ring.[46][47] The waves are interpreted as a spiral pattern of vertical corrugations of 2 to 20 m amplitude;[48] the fact that the period of the waves is decreasing over time (from 60 km in 1995 to 30 km by 2006) allows a deduction that the pattern may have originated in late 1983 with the impact of a cloud of debris (with a mass of ~1012 kg) from a disrupted comet that tilted the rings out of the equatorial plane.[45][46][49] A similar spiral pattern in Jupiter's main ring has been attributed to a perturbation caused by impact of material from Comet Shoemaker-Levy 9 in 1994.[46][50][51]
C Ring

The C Ring is a wide but faint ring located inward of the B Ring. It was discovered in 1850 by William and George Bond, though William R. Dawes and Johann Galle also saw it independently. William Lassell termed it the "Crepe Ring" because it seemed to be composed of darker material than the brighter A and B Rings.[52]
Its vertical thickness is estimated at 5 m its mass at around 1.1 × 1018 kg, and its optical depth varies from 0.05 to 0.12. That is, between 5 and 12 percent of light shining perpendicularly through the ring is blocked, so that when seen from above, the ring is close to transparent. The 30-km wavelength spiral corrugations first seen in the D Ring were observed during Saturn's equinox of 2009 to extend throughout the C Ring (see above).
Colombo Gap and Titan Ringlet
The Colombo Gap lies in the inner C Ring. Within the gap lies the bright but narrow Colombo Ringlet, centered at 77,883 km from Saturn's center, which is slightly elliptical rather than circular. This ringlet is also called the Titan Ringlet as it is governed by an orbital resonance with the moon Titan.[53] At this location within the rings, the length of a ring particle's apsidal precession is equal to the length of Titan's orbital motion, so that the outer end of this eccentric ringlet always points towards Titan.[53]
Maxwell Gap and Ringlet
The Maxwell Gap lies within the outer part of the C Ring. It also contains a dense non-circular ringlet, the Maxwell Ringlet. In many respects this ringlet is similar to the ε ring of Uranus. There are wave-like structures in the middle of both rings. While the wave in the ε ring is thought to be caused by Uranian moon Cordelia, no moon has been discovered in the Maxwell gap as of July 2008.[54]
B Ring

The B Ring is the largest, brightest, and most massive of the rings. Its thickness is estimated as 5 to 15 m and its optical depth varies from 0.4 to greater than 5,[55] meaning that >99% of the light passing through some parts of the B Ring is blocked. The B Ring contains a great deal of variation in its density and brightness, nearly all of it unexplained. These are concentric, appearing as narrow ringlets, though the B Ring does not contain any gaps.. In places, the outer edge of the B Ring contains vertical structures deviating up to 2.5 km from the main ring plane.
A 2016 study of spiral density waves using stellar occultations indicated that the B Ring's surface density is in the range of 40 to 140 g/cm2, lower than previously believed, and that the ring's optical depth has little correlation with its mass density (a finding previously reported for the A and C rings).[55][56] The total mass of the B Ring was estimated to be somewhere in the range of 7 to ×1018 kg. This compares to a mass for 24Mimas of ×1018 kg. 37.5[55]
Spokes
Until 1980, the structure of the rings of Saturn was explained as being caused exclusively by the action of gravitational forces. Then images from the Voyager spacecraft showed radial features in the B ring, known as spokes,[57][58] which could not be explained in this manner, as their persistence and rotation around the rings was not consistent with gravitational orbital mechanics.[59] The spokes appear dark in backscattered light, and bright in forward-scattered light (see images in gallery); the transition occurs at a phase angle near 60°. The leading theory regarding the spokes' composition is that they consist of microscopic dust particles suspended away from the main ring by electrostatic repulsion, as they rotate almost synchronously with the magnetosphere of Saturn. The precise mechanism generating the spokes is still unknown, although it has been suggested that the electrical disturbances might be caused by either lightning bolts in Saturn's atmosphere or micrometeoroid impacts on the rings.[59]
The spokes were not observed again until some twenty-five years later, this time by the Cassini space probe. The spokes were not visible when Cassini arrived at Saturn in early 2004. Some scientists speculated that the spokes would not be visible again until 2007, based on models attempting to describe their formation. Nevertheless, the Cassini imaging team kept looking for spokes in images of the rings, and they were next seen in images taken on 5 September 2005.[60]
The spokes appear to be a seasonal phenomenon, disappearing in the Saturnian midwinter and midsummer and reappearing as Saturn comes closer to equinox. Suggestions that the spokes may be a seasonal effect, varying with Saturn's 29.7-year orbit, were supported by their gradual reappearance in the later years of the Cassini mission.[61]
Moonlet
In 2009, during equinox, a moonlet embedded in the B ring was discovered from the shadow it cast. It is estimated to be 400 m (1,300 ft) in diameter.[62] The moonlet was given the provisional designation S/2009 S 1.
Cassini Division
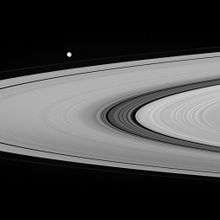
The Cassini Division is a region 4,800 km (3,000 mi) in width between Saturn's A Ring and B Ring. It was discovered in 1675 by Giovanni Cassini at the Paris Observatory using a refracting telescope that had a 2.5-inch objective lens with a 20-foot-long focal length and a 90x magnification.[63][64] From Earth it appears as a thin black gap in the rings. However, Voyager discovered that the gap is itself populated by ring material bearing much similarity to the C Ring.[54] The division may appear bright in views of the unlit side of the rings, since the relatively low density of material allows more light to be transmitted through the thickness of the rings (see second image in gallery).
The inner edge of the Cassini Division is governed by a strong orbital resonance. Ring particles at this location orbit twice for every orbit of the moon Mimas.[65] The resonance causes Mimas' pulls on these ring particles to accumulate, destabilizing their orbits and leading to a sharp cutoff in ring density. Many of the other gaps between ringlets within the Cassini Division, however, are unexplained.
Huygens Gap
The Huygens Gap is located at the inner edge of the Cassini Division. It contains the dense, eccentric Huygens Ringlet in the middle. This ringlet exhibits irregular azimuthal variations of geometrical width and optical depth, which may be caused by the nearby 2:1 resonance with Mimas and the influence of the eccentric outer edge of the B-ring. There is an additional narrow ringlet just outside the Huygens Ringlet.[54]
A Ring

The A Ring is the outermost of the large, bright rings. Its inner boundary is the Cassini Division and its sharp outer boundary is close to the orbit of the small moon Atlas. The A Ring is interrupted at a location 22% of the ring width from its outer edge by the Encke Gap. A narrower gap 2% of the ring width from the outer edge is called the Keeler Gap.
The thickness of the A Ring is estimated to be 10 to 30 m, its surface density from 35 to 40 g/cm2 and its total mass as 4 to ×1018 kg 5[55] (just under the mass of Hyperion). Its optical depth varies from 0.4 to 0.9.[55]
Similarly to the B Ring, the A Ring's outer edge is maintained by an orbital resonance, in this case the 7:6 resonance with Janus and Epimetheus. Other orbital resonances also excite many spiral density waves in the A Ring (and, to a lesser extent, other rings as well), which account for most of its structure. These waves are described by the same physics that describes the spiral arms of galaxies. Spiral bending waves, also present in the A Ring and also described by the same theory, are vertical corrugations in the ring rather than compression waves.
In April 2014, NASA scientists reported observing the possible formative stage of a new moon near the outer edge of the A Ring.[66][67]
Encke Gap
The Encke Gap is a 325-km-wide gap within the A Ring, centered at a distance of 133,590 km from Saturn's center.[68] It is caused by the presence of the small moon Pan,[69] which orbits within it. Images from the Cassini probe have shown that there are at least three thin, knotted ringlets within the gap.[54] Spiral density waves visible on both sides of it are induced by resonances with nearby moons exterior to the rings, while Pan induces an additional set of spiraling wakes (see image in gallery).[54]
Johann Encke himself did not observe this gap; it was named in honour of his ring observations. The gap itself was discovered by James Edward Keeler in 1888.[52] The second major gap in the A Ring, discovered by Voyager, was named the Keeler Gap in his honor.[70]
The Encke Gap is a gap because it is entirely within the A Ring. There was some ambiguity between the terms gap and division until the IAU clarified the definitions in 2008; before that, the separation was sometimes called the "Encke Division".[71]
Keeler Gap

The Keeler Gap is a 42-km-wide gap in the A Ring, approximately 250 km from the ring's outer edge. The small moon Daphnis, discovered 1 May 2005, orbits within it, keeping it clear.[72] The moon's passage induces waves in the edges of the gap (this is also influenced by its slight orbital eccentricity).[54] Because the orbit of Daphnis is slightly inclined to the ring plane, the waves have a component that is perpendicular to the ring plane, reaching a distance of 1500 m "above" the plane.[73][74]
The Keeler gap was discovered by Voyager, and named in honor of the astronomer James Edward Keeler. Keeler had in turn discovered and named the Encke Gap in honor of Johann Encke.[52]
Moonlets
.jpg)
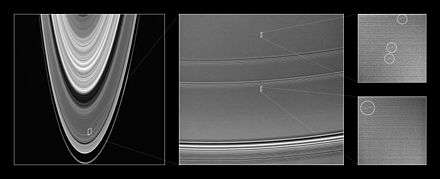
In 2006, four tiny "moonlets" were found in Cassini images of the A Ring.[75] The moonlets themselves are only about a hundred m in diameter, too small to be seen directly; what Cassini sees are the "propeller"-shaped disturbances the moonlets create, which are several km across. It is estimated that the A Ring contains thousands of such objects. In 2007, the discovery of eight more moonlets revealed that they are largely confined to a 3,000 km belt, about 130,000 km from Saturn's center,[76] and by 2008 over 150 propeller moonlets had been detected.[77] One that has been tracked for several years has been nicknamed Bleriot.[78]
Roche Division

The separation between the A Ring and the F Ring has been named the Roche Division in honor of the French physicist Édouard Roche.[79] The Roche Division should not be confused with the Roche limit which is the distance at which a large object is so close to a planet (such as Saturn) that the planet's tidal forces will pull it apart.[80] Lying at the outer edge of the main ring system, the Roche Division is in fact close to Saturn's Roche limit, which is why the rings have been unable to accrete into a moon.[81]
Like the Cassini Division, the Roche Division is not empty but contains a sheet of material. The character of this material is similar to the tenuous and dusty D, E, and G Rings. Two locations in the Roche Division have a higher concentration of dust than the rest of the region. These were discovered by the Cassini probe imaging team and were given temporary designations: R/2004 S 1, which lies along the orbit of the moon Atlas; and R/2004 S 2, centered at 138,900 km from Saturn's center, inward of the orbit of Prometheus.[82][83]
F Ring
The F Ring is the outermost discrete ring of Saturn and perhaps the most active ring in the Solar System, with features changing on a timescale of hours.[84] It is located 3,000 km beyond the outer edge of the A Ring.[85] The ring was discovered in 1979 by the Pioneer 11 imaging team.[86] It is very thin, just a few hundred km in radial extent. While the traditional view has been that it is held together by two shepherd moons, Prometheus and Pandora, which orbit inside and outside it,[69] recent studies indicate that only Prometheus contributes to the confinement.[87][88] Numerical simulations suggest the ring was formed when Prometheus and Pandora collided with each other and were partially disrupted.[89]
Recent closeup images from the Cassini probe show that the F Ring consists of one core ring and a spiral strand around it.[90] They also show that when Prometheus encounters the ring at its apoapsis, its gravitational attraction creates kinks and knots in the F Ring as the moon 'steals' material from it, leaving a dark channel in the inner part of the ring (see video link and additional F Ring images in gallery). Since Prometheus orbits Saturn more rapidly than the material in the F ring, each new channel is carved about 3.2 degrees in front of the previous one.[84]
In 2008, further dynamism was detected, suggesting that small unseen moons orbiting within the F Ring are continually passing through its narrow core because of perturbations from Prometheus. One of the small moons was tentatively identified as S/2004 S 6.[84]
Outer rings

Janus/Epimetheus Ring
A faint dust ring is present around the region occupied by the orbits of Janus and Epimetheus, as revealed by images taken in forward-scattered light by the Cassini spacecraft in 2006. The ring has a radial extent of about 5,000 km.[91] Its source is particles blasted off the moons' surfaces by meteoroid impacts, which then form a diffuse ring around their orbital paths.[92]
G Ring
The G Ring (see last image in gallery) is a very thin, faint ring about halfway between the F Ring and the beginning of the E Ring, with its inner edge about 15,000 km inside the orbit of Mimas. It contains a single distinctly brighter arc near its inner edge (similar to the arcs in the rings of Neptune) that extends about one sixth of its circumference, centered on the half-km diameter moonlet Aegaeon, which is held in place by a 7:6 orbital resonance with Mimas.[93][94] The arc is believed to be composed of icy particles up to a few m in diameter, with the rest of the G Ring consisting of dust released from within the arc. The radial width of the arc is about 250 km, compared to a width of 9,000 km for the G Ring as a whole.[93] The arc is thought to contain matter equivalent to a small icy moonlet about a hundred m in diameter.[93] Dust released from Aegaeon and other source bodies within the arc by micrometeoroid impacts drifts outward from the arc because of interaction with Saturn's magnetosphere (whose plasma corotates with Saturn's magnetic field, which rotates much more rapidly than the orbital motion of the G Ring). These tiny particles are steadily eroded away by further impacts and dispersed by plasma drag. Over the course of thousands of years the ring gradually loses mass,[95] which is replenished by further impacts on Aegaeon.
Methone Ring Arc
A faint ring arc, first detected in September 2006, covering a longitudinal extent of about 10 degrees is associated with the moon Methone. The material in the arc is believed to represent dust ejected from Methone by micrometeoroid impacts. The confinement of the dust within the arc is attributable to a 14:15 resonance with Mimas (similar to the mechanism of confinement of the arc within the G ring).[96][97] Under the influence of the same resonance, Methone librates back and forth in its orbit with an amplitude of 5° of longitude.
Anthe Ring Arc

A faint ring arc, first detected in June 2007, covering a longitudinal extent of about 20 degrees is associated with the moon Anthe. The material in the arc is believed to represent dust knocked off Anthe by micrometeoroid impacts. The confinement of the dust within the arc is attributable to a 10:11 resonance with Mimas. Under the influence of the same resonance, Anthe drifts back and forth in its orbit over 14° of longitude.[96][97]
Pallene Ring
A faint dust ring shares Pallene's orbit, as revealed by images taken in forward-scattered light by the Cassini spacecraft in 2006.[91] The ring has a radial extent of about 2,500 km. Its source is particles blasted off Pallene's surface by meteoroid impacts, which then form a diffuse ring around its orbital path.[92][97]
E Ring
The E Ring is the second outermost ring and is extremely wide; it consists of many tiny (micron and sub-micron) particles of water ice with silicates, carbon dioxide and ammonia.[98] The E Ring is distributed between the orbits of Mimas and Titan.[99] Unlike the other rings, it is composed of microscopic particles rather than macroscopic ice chunks. In 2005, the source of the E Ring's material was determined to be cryovolcanic plumes[100][101] emanating from the "tiger stripes" of the south polar region of the moon Enceladus.[102] Unlike the main rings, the E Ring is more than 2,000 km thick and increases with its distance from Enceladus.[99] Tendril-like structures observed within the E Ring can be related to the emissions of the most active south polar jets of Enceladus.[103]
Particles of the E Ring tend to accumulate on moons that orbit within it. The equator of the leading hemisphere of Tethys is tinted slightly blue due to infalling material.[104] The trojan moons Telesto, Calypso, Helene and Polydeuces are particularly affected as their orbits move up and down the ring plane. This results in their surfaces being coated with bright material that smooths out features.[105]

The moon's south polar jets erupt brightly below it.

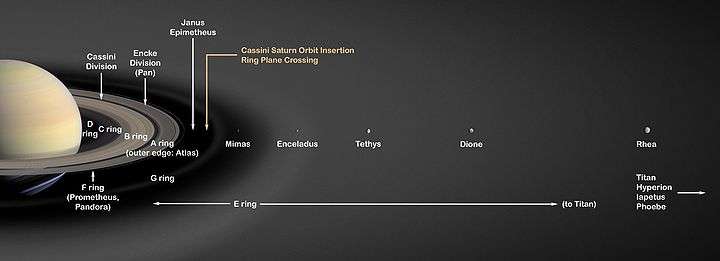

Phoebe ring
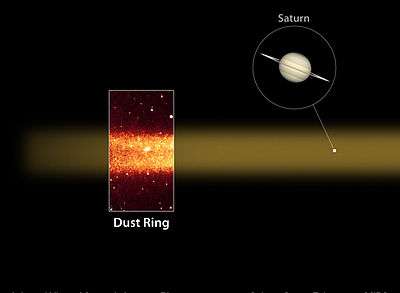
In October 2009, the discovery of a tenuous disk of material just interior to the orbit of Phoebe was reported. The disk was aligned edge-on to Earth at the time of discovery. This disk can be loosely described as another ring. Although very large (the apparent size of two full moons as seen from Earth), the ring is virtually invisible. It was discovered using NASA's infrared Spitzer Space Telescope,[106] and was seen over the entire range of the observations, which extended from 128 to 207 times the radius of Saturn,[107] with calculations indicating that it may extend outward up to 300 Saturn radii and inward to the orbit of Iapetus at 59 Saturn radii.[108] The ring was subsequently studied using the WISE, Herschel and Cassini spacecraft;[109] WISE observations show that it extends from at least between 50 and 100 to 270 Saturn radii (the inner edge is lost in the planet's glare).[110] Data obtained with WISE indicate the ring particles are small; those with radii of greater than 10 cm comprise 10% or less of the cross-sectional area.[110]
Phoebe orbits the planet at a distance ranging from 180 to 250 radii. The ring has a thickness of about 40 radii.[111] Because the ring's particles are presumed to have originated from impacts (micrometeoroid and larger) on Phoebe, they should share its retrograde orbit,[108] which is opposite to the orbital motion of the next inner moon, Iapetus. This ring lies in the plane of Saturn's orbit, or roughly the ecliptic, and thus is tilted 27 degrees from Saturn's equatorial plane and the other rings. Phoebe is inclined by 5° with respect to Saturn's orbit plane (often written as 175°, due to Phoebe's retrograde orbital motion), and its resulting vertical excursions above and below the ring plane agree closely with the ring's observed thickness of 40 Saturn radii.
The existence of the ring was proposed in the 1970s by Steven Soter.[108] The discovery was made by Anne J. Verbiscer and Michael F. Skrutskie (of the University of Virginia) and Douglas P. Hamilton (of the University of Maryland, College Park).[107][112] The three had studied together at Cornell University as graduate students.[113]
Ring material migrates inward due to reemission of solar radiation,[107] with a speed inversely proportional to particle size; a 3 cm particle would migrate from the vicinity of Phoebe to that of Iapetus over the age of the Solar System.[110] The material would thus strike the leading hemisphere of Iapetus. Infall of this material causes a slight darkening and reddening of the leading hemisphere of Iapetus (similar to what is seen on the Uranian moons Oberon and Titania) but does not directly create the dramatic two-tone coloration of that moon.[114] Rather, the infalling material initiates a positive feedback thermal self-segregation process of ice sublimation from warmer regions, followed by vapor condensation onto cooler regions. This leaves a dark residue of "lag" material covering most of the equatorial region of Iapetus's leading hemisphere, which contrasts with the bright ice deposits covering the polar regions and most of the trailing hemisphere.[115][116][117]
Possible ring system around Rhea
Saturn's second largest moon Rhea has been hypothesized to have a tenuous ring system of its own consisting of three narrow bands embedded in a disk of solid particles.[118][119] These putative rings have not been imaged, but their existence has been inferred from Cassini observations in November 2005 of a depletion of energetic electrons in Saturn's magnetosphere near Rhea. The Magnetospheric Imaging Instrument (MIMI) observed a gentle gradient punctuated by three sharp drops in plasma flow on each side of the moon in a nearly symmetric pattern. This could be explained if they were absorbed by solid material in the form of an equatorial disk containing denser rings or arcs, with particles perhaps several dm to approximately a m in diameter. A more recent piece of evidence consistent with the presence of Rhean rings is a set of small ultraviolet-bright spots distributed in a line that extends three quarters of the way around the moon's circumference, within 2 degrees of the equator. The spots have been interpreted as the impact points of deorbiting ring material.[120] However, targeted observations by Cassini of the putative ring plane from several angles have turned up nothing, suggesting that another explanation for these enigmatic features is needed.[121]
Gallery
![]() Image of part of the D Ring from within, taken by Cassini during its close approach to the inner edge of the ring on 28 May 2017. |
![]() Cassini image mosaic of the unlit side of the inner B Ring (top) and outer C Ring (bottom) near Saturn's equinox, showing multiple views of the shadow of Mimas. The shadow is attenuated by the denser B ring. The Maxwell Gap is below center. |
![]() Dark B Ring spokes in a low-phase-angle Cassini image of the rings' unlit side. Left of center, two dark gaps (the larger being the Huygens Gap) and the bright (from this viewing geometry) ringlets to their left comprise the Cassini Division. |
![]() Cassini image of the sun-lit side of the rings taken in 2009 at a phase angle of 144°, with bright B Ring spokes. |
![]() Pan's motion through the A Ring's Encke Gap induces edge waves and (non-self-propagating) spiraling wakes ahead of and inward of it. The other more tightly wound bands are spiral density waves. |
![]() |
![]() F ring dynamism, probably due to perturbing effects of small moonlets orbiting close to or through the ring's core. |
![]() Prometheus (at center) and Pandora orbit just inside and outside the F Ring, but only Prometheus acts as a ring shepherd. |
See also
- Galileo Galilei – the first person to observe Saturn's rings, in 1610
- Christiaan Huygens – the first person to propose that there was a ring surrounding Saturn, in 1655
- Giovanni Cassini – discovered the separation between the A and B rings (the Cassini Division), in 1675
- Édouard Roche – French astronomer who described how a satellite that comes within the Roche limit of Saturn could break up and form the rings
References
- ↑ Porco, Carolyn. "Questions about Saturn's rings". CICLOPS web site. Retrieved 2012-10-05.
- 1 2 Tiscareno, M. S. (2012-07-04). "Planetary Rings". In Kalas, P.; French, L. Planets, Stars and Stellar Systems. Springer. pp. 61–63. ISBN 978-94-007-5605-2. doi:10.1007/978-94-007-5606-9_7. Retrieved 2012-10-05.
- 1 2 3 4 5 6 Baalke, Ron. "Historical Background of Saturn's Rings". Saturn Ring Plane Crossings of 1995–1996. Jet Propulsion Laboratory. Archived from the original on 2009-03-21. Retrieved 2007-05-23.
- 1 2 Whitehouse, David (2009). Renaissance Genius: Galileo Galilei and His Legacy to Modern Science. Sterling Publishing Company, Inc. p. 100. ISBN 978-1-4027-6977-1. OCLC 434563173.
- ↑ Miner, Ellis D.; et al. (2007). "The scientific significance of planetary ring systems". Planetary Ring Systems. Springer Praxis Books in Space Exploration. Praxis. pp. 1–16. ISBN 978-0-387-34177-4. doi:10.1007/978-0-387-73981-6_1.
- ↑ Alexander, A. F. O'D. (1962). The Planet Saturn. London: Faber and Faber Limited. pp. 108–109. ISBN 0-486-23927-6. doi:10.1002/qj.49708837730.
- ↑ "Saturn's Cassini Division". StarChild. Retrieved 2007-07-06.
- 1 2 "James Clerk Maxwell on the nature of Saturn's rings". JOC/EFR. March 2006. Retrieved 2007-07-08.
- ↑ "Kovalevsky, Sonya (or Kovalevskaya, Sofya Vasilyevna). Entry from Complete Dictionary of Scientific Biography". 2013.
- 1 2 Dunford, Bill. "Pioneer 11 - In Depth". NASA web site. Retrieved 2015-12-03.
- 1 2 Angrum, Andrea. "Voyager - The Interstellar Mission". JPL/NASA web site. Retrieved 2015-12-03.
- ↑ Dunford, Bill. "Voyager 1 - In Depth". NASA web site. Retrieved 2015-12-03.
- ↑ Dunford, Bill. "Voyager 2 - In Depth". NASA web site. Retrieved 2015-12-03.
- ↑ Dunford, Bill. "Cassini - Key Dates". NASA web site. Retrieved 2015-12-03.
- ↑ Piazza, Enrico. "Cassini Solstice Mission: About Saturn & Its Moons". JPL/NASA web site. Retrieved 2015-12-03.
- 1 2 "Solar System Exploration: Planets: Saturn: Rings". Solar System Exploration.
- ↑ Cornell University News Service (2005-11-10). "Researchers Find Gravitational Wakes In Saturn's Rings". ScienceDaily. Retrieved 2008-12-24.
- ↑ "Saturn: Rings". NASA.
- ↑ Nicholson, P.D.; et al. (2008). "A close look at Saturn's rings with Cassini VIMS". Icarus. 193 (1): 182–212. Bibcode:2008Icar..193..182N. doi:10.1016/j.icarus.2007.08.036.
- ↑ Zebker, H.A.; et al. (1985). "Saturn's rings – Particle size distributions for thin layer model". Icarus. 64 (3): 531–548. Bibcode:1985Icar...64..531Z. doi:10.1016/0019-1035(85)90074-0.
- ↑ Jerome Brainerd, "Saturn's Rings", The Astrophysics Spectator, Issue 1.8, 24 November 2004, retrieved 27 May 2009.
- ↑ Stewart, Glen R.; et al. (October 2007). "Evidence for a Primordial Origin of Saturn's Rings". Bulletin of the American Astronomical Society. American Astronomical Society, DPS meeting #39. American Astronomical Society. 39: 420. Bibcode:2007DPS....39.0706S. Retrieved 2009-05-27.
- ↑ Burns, J.A.; et al. (2001). "Dusty Rings and Circumplanetary Dust: Observations and Simple Physics" (PDF). In Grun, E.; Gustafson, B. A. S.; Dermott, S. T.; Fechtig H. Interplanetary Dust. Berlin: Springer. pp. 641–725. Bibcode:2001indu.book..641B. ISBN 3-540-42067-3.
- ↑ Goldreich, Peter; et al. (1978). "The formation of the Cassini division in Saturn's rings". Icarus. 34 (2): 240–253. Bibcode:1978Icar...34..240G. doi:10.1016/0019-1035(78)90165-3.
- ↑ Rincon, Paul (2005-07-01). "Saturn rings have own atmosphere". British Broadcasting Corporation. Retrieved 2007-07-06.
- ↑ Johnson, R. E.; et al. (2006). "The Enceladus and OH Tori at Saturn". The Astrophysical Journal. The American Astronomical Society. 644 (2): L137. Bibcode:2006ApJ...644L.137J. doi:10.1086/505750.
- ↑ Schmude, Richard W Junior (2001). "Wideband photoelectric magnitude measurements of Saturn in 2000" (PDF). Georgia Journal of Science. Retrieved 2007-10-14.
- ↑ Schmude, Richard, Jr. (2006-09-22). "Wideband photometric magnitude measurements of Saturn made during the 2005–06 Apparition" (PDF). Georgia Journal of Science. Retrieved 2007-10-14.
- ↑ Schmude, Richard W Jr (2003). "Saturn in 2002–03". Georgia Journal of Science. Retrieved 2007-10-14.
- ↑ "The Journal of the British Astronomical Association". British Astronomical Association. February 2003. Retrieved 2007-07-07.
- ↑ "Surprising, Huge Peaks Discovered in Saturn's Rings". SPACE.com Staff. space.com. 2009-09-21. Retrieved 2009-09-26.
- ↑ Baalke, Ron. "Historical Background of Saturn's Rings". 1849 Roche Proposes Tidal Break-up. Jet Propulsion Laboratory. Retrieved 2008-09-13.
- ↑ "The Real Lord of the Rings". nasa.gov.
- ↑ Kerr, Richard A (2008). "Saturn's Rings Look Ancient Again". Science. 319 (5859): 21. PMID 18174403. doi:10.1126/science.319.5859.21a.
- ↑ Choi, C. Q. (2010-12-13). "Saturn's Rings Made by Giant "Lost" Moon, Study Hints". National Geographic. Retrieved 2012-11-05.
- 1 2 3 Canup, R. M. (2010-12-12). "Origin of Saturn’s rings and inner moons by mass removal from a lost Titan-sized satellite". Nature. 468 (7326): 943–6. Bibcode:2010Natur.468..943C. PMID 21151108. doi:10.1038/nature09661. Retrieved 2012-11-04.
- 1 2 Charnoz, S.; et al. (December 2011). "Accretion of Saturn’s mid-sized moons during the viscous spreading of young massive rings: Solving the paradox of silicate-poor rings versus silicate-rich moons". Icarus. 216 (2): 535–550. Bibcode:2011Icar..216..535C. arXiv:1109.3360
. doi:10.1016/j.icarus.2011.09.017. Retrieved 2012-10-05.
- ↑ "Saturn's Rings May Be Old Timers". NASA/JPL and University of Colorado. 2007-12-12. Retrieved 2008-01-24.
- ↑ Romero, James. "Saturn’s Rings Could Have Formed when Dinosaurs Walked the Earth". sci-news.com. sci-news.com. Retrieved 16 December 2016.
- ↑ Esposito, L.W.; et al. (January 2012). "A predator–prey model for moon-triggered clumping in Saturn’s rings". Icarus. 217 (1): 103–114. Bibcode:2012Icar..217..103E. doi:10.1016/j.icarus.2011.09.029.
- ↑ Porco, C.; et al. (October 1984). "The Eccentric Saturnian Ringlets at 1.29RS and 1.45RS". Icarus. Elsevier Science. 60 (1): 1–16. Bibcode:1984Icar...60....1P. doi:10.1016/0019-1035(84)90134-9.
- ↑ Porco, C. C.; et al. (November 1987). "Eccentric features in Saturn's outer C ring". Icarus. Elsevier Science. 72 (2): 437–467. Bibcode:1987Icar...72..437P. doi:10.1016/0019-1035(87)90185-0.
- ↑ Flynn, B. C.; et al. (November 1989). "Regular Structure in the Inner Cassini Division of Saturn's Rings". Icarus. Elsevier Science. 82 (1): 180–199. Bibcode:1989Icar...82..180F. doi:10.1016/0019-1035(89)90030-4.
- ↑ Lakdawalla, E. (2009-02-09). "New names for gaps in the Cassini Division within Saturn's rings". New names for gaps in Saturn's rings – The Planetary Society Blog. Planetary Society. Retrieved 2009-01-11.
- 1 2 3 Hedman, Matthew M.; et al. (2007). "Saturn's dynamic D ring" (PDF). Icarus. 188 (1): 89–107. Bibcode:2007Icar..188...89H. doi:10.1016/j.icarus.2006.11.017.
- 1 2 3 Mason, J.; et al. (2011-03-31). "Forensic sleuthing ties ring ripples to impacts". CICLOPS press release. Cassini Imaging Central Laboratory for Operations. Retrieved 2011-04-04.
- ↑ "Extensive spiral corrugations". PIA 11664 caption. NASA / Jet Propulsion Laboratory / Space Science Institute. 2011-03-31. Retrieved 2011-04-04.
- ↑ "Tilting Saturn's rings". PIA 12820 caption. NASA / Jet Propulsion Laboratory / Space Science Institute. 2011-03-31. Retrieved 2011-04-04.
- ↑ Hedman, M. M.; et al. (2011-03-31). "Saturn's curiously corrugated C Ring". Science. AAAS. 332 (6030): 708. Bibcode:2011Sci...332..708H. PMID 21454753. doi:10.1126/science.1202238. Retrieved 2011-04-05.
- ↑ "Subtle Ripples in Jupiter's Ring". PIA 13893 caption. NASA / Jet Propulsion Laboratory-Caltech / SETI. 2011-03-31. Retrieved 2011-04-04.
- ↑ Showalter, M. R.; et al. (2011-03-31). "The impact of comet Shoemaker-Levy 9 sends ripples through the rings of Jupiter". Science. AAAS. 332 (6030): 711. Bibcode:2011Sci...332..711S. PMID 21454755. doi:10.1126/science.1202241. Retrieved 2011-04-05.
- 1 2 3 Harland, David M., Mission to Saturn: Cassini and the Huygens Probe, Chichester: Praxis Publishing, 2002.
- 1 2 Porco, C.; et al. (October 1984). "The eccentric Saturnian ringlets at 1.29Rs and 1.45Rs". Icarus. 60 (1): 1–16. Bibcode:1984Icar...60....1P. doi:10.1016/0019-1035(84)90134-9. Retrieved 2014-01-09.
- 1 2 3 4 5 6 Porco, C.C.; et al. (2005). "Cassini Imaging Science: Initial Results on Saturn'sRings and Small Satellites" (PDF). Science. 307 (5713): 1226–1236. Bibcode:2005Sci...307.1226P. PMID 15731439. doi:10.1126/science.1108056.
- 1 2 3 4 5 Hedman, M.M.; Nicholson, P.D. (2016-01-22). "The B-ring’s surface mass density from hidden density waves: Less than meets the eye?". Icarus. doi:10.1016/j.icarus.2016.01.007.
- ↑ Dyches, Preston (2 February 2016). "Saturn's Rings: Less than Meets the Eye?". NASA. Retrieved 3 February 2016.
- ↑ Smith, B. A.; Soderblom, L.; Batson, R.; Bridges, P.; Inge, J.; Masursky, H.; Shoemaker, E.; Beebe, R.; Boyce, J.; Briggs, G.; Bunker, A.; Collins, S. A.; Hansen, C. J.; Johnson, T. V.; Mitchell, J. L.; Terrile, R. J.; Cook Af, A. F.; Cuzzi, J.; Pollack, J. B.; Danielson, G. E.; Ingersoll, A. P.; Davies, M. E.; Hunt, G. E.; Morrison, D.; Owen, T.; Sagan, C.; Veverka, J.; Strom, R.; Suomi, V. E. (1982). "A New Look at the Saturn System: The Voyager 2 Images". Science. 215 (4532): 504–537. PMID 17771273. doi:10.1126/science.215.4532.504.
- ↑ "The Alphabet Soup of Saturn's Rings". The Planetary Society. 2007. Archived from the original on 2010-12-13. Retrieved 2007-07-24.
- 1 2 Hamilton, Calvin (2004). "Saturn's Magnificent Rings". Retrieved 2007-07-25.
- ↑ Malik, Tarig (2005-09-15). "Cassini Probe Spies Spokes in Saturn's Rings". Imaginova Corp. Retrieved 2007-07-06.
- ↑ Mitchell, C.J.; et al. (2006). "Saturn's Spokes: Lost and Found" (PDF). Science. 311 (5767): 1587–9. Bibcode:2006Sci...311.1587M. PMID 16543455. doi:10.1126/science.1123783.
- ↑ "Cassini Solstice Mission: A Small Find Near Equinox". Cassini Solstice Mission.
- ↑ "Celestial Objects for Common Telescopes". google.com.
- ↑ Archie Frederick Collins, The greatest eye in the world: astronomical telescopes and their stories, page 8
- ↑ "Lecture 41: Planetary Rings". ohio-state.edu.
- ↑ Platt, Jane; et al. (14 April 2014). "NASA Cassini Images May Reveal Birth of a Saturn Moon". NASA.
- ↑ Murray, C. D.; Cooper, N. J.; Williams, G. A.; Attree, N. O.; Boyer, J. S. (2014-03-28). "The discovery and dynamical evolution of an object at the outer edge of Saturn's a ring". Icarus. 236: 165–168. Bibcode:2014Icar..236..165M. doi:10.1016/j.icarus.2014.03.024.
- ↑ Williams, David R. "Saturnian Rings Fact Sheet". NASA. Retrieved 2008-07-22.
- 1 2 Esposito, L. W. (2002). "Planetary rings" (PDF). Reports on Progress in Physics. 65 (12): 1741–1783. Bibcode:2002RPPh...65.1741E. doi:10.1088/0034-4885/65/12/201.
- ↑ Osterbrock, D. E.; Cruikshank, D. P. (1983). "J.E. Keeler's discovery of a gap in the outer part of the a ring". Icarus. 53 (2): 165. Bibcode:1983Icar...53..165O. doi:10.1016/0019-1035(83)90139-2.
- ↑ Blue, J. (2008-02-06). "Encke Division Changed to Encke Gap". USGS Astrogeology Science Center. USGS. Retrieved 2010-09-02.
- ↑ Porco, C.C.; et al. (2007). "Saturn's Small Inner Satellites: Clues to Their Origins" (PDF). Science. 318 (5856): 1602–1607. Bibcode:2007Sci...318.1602P. PMID 18063794. doi:10.1126/science.1143977.
- ↑ Mason, Joe (11 June 2009). "Saturn's Approach To Equinox Reveals Never-before-seen Vertical Structures In Planet's Rings". CICLOPS web site. Retrieved 2009-06-13.
- ↑ Weiss, J. W.; et al. (11 June 2009). "Ring Edge Waves and the Masses of Nearby Satellites". The Astronomical Journal. American Astronomical Society. 138 (1): 272–286. Bibcode:2009AJ....138..272W. doi:10.1088/0004-6256/138/1/272. Retrieved 2009-06-15.
- ↑ Tiscareno, Matthew S.; et al. (2006). "100-m-diameter moonlets in Saturn's A ring from observations of 'propeller' structures". Nature. 440 (7084): 648–650. Bibcode:2006Natur.440..648T. PMID 16572165. doi:10.1038/nature04581.
- ↑ Sremčević, Miodrag; et al. (2007). "A belt of moonlets in Saturn's A ring". Nature. 449 (7165): 1019–1021. Bibcode:2007Natur.449.1019S. PMID 17960236. doi:10.1038/nature06224.
- ↑ Tiscareno, Matthew S.; et al. (2008). "The population of propellers in Saturn's A Ring". Astronomical Journal. 135 (3): 1083–1091. Bibcode:2008AJ....135.1083T. arXiv:0710.4547
. doi:10.1088/0004-6256/135/3/1083.
- ↑ Porco, C. (2013-02-25). "Bleriot Recaptured". CICLOPS web site. NASA/JPL-Caltech/Space Science Institute. Retrieved 2013-02-27.
- ↑ "Planetary Names: Ring and Ring Gap Nomenclature". usgs.gov.
- ↑ Weisstein, Eric W. (2007). "Eric Weisstein's World of Physics – Roche Limit". scienceworld.wolfram.com. Retrieved 2007-09-05.
- ↑ NASA. "What is the Roche limit?". NASA–JPL. Retrieved 2007-09-05.
- ↑ http://www.cbat.eps.harvard.edu/iauc/08400/08401.html
- ↑ http://www.cbat.eps.harvard.edu/iauc/08400/08432.html
- 1 2 3 Murray, C. D.; et al. (June 5, 2008). "The determination of the structure of Saturn's F ring by nearby moonlets". Nature. Nature Publishing Group. 453 (7196): 739–744. Bibcode:2008Natur.453..739M. PMID 18528389. doi:10.1038/nature06999.
- ↑ Karttunen, H.; et al. (2007). Fundamental Astronomy. Springer-Verlag Berlin Heidelberg. ISBN 978-3-540-34144-4. OCLC 804078150. Retrieved 2013-05-25.
- ↑ Gehrels, T.; Baker, L. R.; Beshore, E.; Blenman, C.; Burke, J. J.; Castillo, N. D.; Dacosta, B.; Degewij, J.; Doose, L. R.; Fountain, J. W.; Gotobed, J.; Kenknight, C. E.; Kingston, R.; McLaughlin, G.; McMillan, R.; Murphy, R.; Smith, P. H.; Stoll, C. P.; Strickland, R. N.; Tomasko, M. G.; Wijesinghe, M. P.; Coffeen, D. L.; Esposito, L. (1980). "Imaging Photopolarimeter on Pioneer Saturn". Science. 207 (4429): 434–439. Bibcode:1980Sci...207..434G. PMID 17833555. doi:10.1126/science.207.4429.434.
- ↑ Lakdawalla, E. (2014-07-05). "On the masses and motions of mini-moons: Pandora's not a "shepherd," but Prometheus still is". Planetary Society. Retrieved 2015-04-17.
- ↑ Cuzzi, J. N.; Whizin, A. D.; Hogan, R. C.; Dobrovolskis, A. R.; Dones, L.; Showalter, M. R.; Colwell, J. E.; Scargle, J. D. (April 2014). "Saturn’s F Ring core: Calm in the midst of chaos". Icarus. 232: 157–175. Bibcode:2014Icar..232..157C. ISSN 0019-1035. doi:10.1016/j.icarus.2013.12.027.
- ↑ Hyodo, R.; Ohtsuki, K. (2015-08-17). "Saturn’s F ring and shepherd satellites a natural outcome of satellite system formation". Nature Geoscience. 8: 686–689. doi:10.1038/ngeo2508.
- ↑ Charnoz, S.; et al. (2005). "Cassini Discovers a Kinematic Spiral Ring Around Saturn" (PDF). Science. 310 (5752): 1300–1304. Bibcode:2005Sci...310.1300C. PMID 16311328. doi:10.1126/science.1119387.
- 1 2 NASA Planetary Photojournal PIA08328: Moon-Made Rings
- 1 2 Cassini–Huygens press release NASA Finds Saturn's Moons May Be Creating New Rings, 11 October 2006.
- 1 2 3 Hedman, M. M.; et al. (2007). "The Source of Saturn's G Ring" (PDF). Science. 317 (5838): 653–656. Bibcode:2007Sci...317..653H. PMID 17673659. doi:10.1126/science.1143964.
- ↑ "S/2008 S 1. (NASA Cassini Saturn Mission Images)". ciclops.org.
- ↑ Davison, Anna (2 August 2007). "Saturn ring created by remains of long-dead moon". NewScientist.com news service.
- 1 2 Porco C. C., ; et al. (2008-09-05). "More Ring Arcs for Saturn". Cassini Imaging Central Laboratory for Operations web site. Retrieved 2008-09-05.
- 1 2 3 Hedman, M. M.; et al. (2008-11-25). "Three tenuous rings/arcs for three tiny moons". Icarus. 199 (2): 378–386. Bibcode:2009Icar..199..378H. doi:10.1016/j.icarus.2008.11.001.
- ↑ Hillier, JK; et al. (June 2007). "The composition of Saturn's E Ring". Monthly Notices of the Royal Astronomical Society. 377 (4): 1588–1596. Bibcode:2007MNRAS.377.1588H. doi:10.1111/j.1365-2966.2007.11710.x.
- 1 2 Hedman, M. M.; et al. (2012). "The three-dimensional structure of Saturn's E Ring". Icarus. 217: 322–338. Bibcode:2012Icar..217..322H. arXiv:1111.2568
. doi:10.1016/j.icarus.2011.11.006.
- ↑ Spahn, F.; et al. (2006-03-10). "Cassini Dust Measurements at Enceladus and Implications for the Origin of the E Ring". Science. AAAS. 311 (5766): 1416–8. Bibcode:2006Sci...311.1416S. PMID 16527969. doi:10.1126/science.1121375. Retrieved 2008-09-13.
- ↑ Porco, C. C.; Helfenstein, P.; Thomas, P. C.; Ingersoll, A. P.; Wisdom, J.; West, R.; Neukum, G.; Denk, T.; Wagner, R. (10 March 2006). "Cassini Observes the Active South Pole of Enceladus". Science. 311 (5766): 1393–1401. Bibcode:2006Sci...311.1393P. PMID 16527964. doi:10.1126/science.1123013.
- ↑ "Icy Tendrils Reaching into Saturn Ring Traced to Their Source". NASA News. 14 April 2015. Retrieved 2015-04-15.
- ↑ Mitchell, C. J.; Porco, C. C.; Weiss, J. W. (2015-04-15). "Tracking the geysers of Enceladus into Saturn’s E ring". The Astronomical Journal. 149 (5): 156. Bibcode:2015AJ....149..156M. ISSN 1538-3881. doi:10.1088/0004-6256/149/5/156.
- ↑ Schenk Hamilton et al. 2011, pp. 751–53.
- ↑ Mason 2010.
- ↑ NASA Space Telescope Discovers Largest Ring Around Saturn
- 1 2 3 Verbiscer, Anne; et al. (2009-10-07). "Saturn's largest ring". Nature. 461 (7267): 1098–100. Bibcode:2009Natur.461.1098V. PMID 19812546. doi:10.1038/nature08515.
- 1 2 3 Cowen, Rob (2009-10-06). "Largest known planetary ring discovered". Science News.
- ↑ Tamayo, D.; et al. (2014-01-23). "First observations of the Phoebe ring in optical light". Icarus. 233: 1. Bibcode:2014Icar..233....1T. arXiv:1401.6166
. doi:10.1016/j.icarus.2014.01.021.
- 1 2 3 Hamilton, Douglas P.; Skrutskie, Michael F.; Verbiscer, Anne J.; Masci, Frank J. (2015-06-10). "Small particles dominate Saturn's Phoebe ring to surprisingly large distances". Nature. 522 (7555): 185–187. PMID 26062508. doi:10.1038/nature14476.
- ↑ "The King of Rings". NASA, Spitzer Space Telescope center. 2009-10-07. Retrieved 2009-10-07.
- ↑ Grayson, Michelle (2009-10-07). "Huge 'ghost' ring discovered around Saturn". Nature News. doi:10.1038/news.2009.979.
- ↑ Weil, Martin (Oct 25, 2009). "U-Va., U-Md. astronomers find another Saturn ring". Washington Post. p. 4C. Retrieved 2012-09-02.
- ↑ Denk, T.; et al. (2009-12-10). "Iapetus: Unique Surface Properties and a Global Color Dichotomy from Cassini Imaging". Science. AAAS. 327 (5964): 435–9. Bibcode:2010Sci...327..435D. PMID 20007863. doi:10.1126/science.1177088. Retrieved 2009-12-19.
- ↑ "Cassini Is on the Trail of a Runaway Mystery". NASA Mission News. NASA. 8 October 2007. Retrieved 2009-10-08.
- ↑ Mason, J.; et al. (2009-12-10). "Cassini Closes In On The Centuries-old Mystery Of Saturn's Moon Iapetus". CICLOPS website newsroom. Space Science Institute. Retrieved 2009-12-22.
- ↑ Spencer, J. R.; et al. (2009-12-10). "Formation of Iapetus' Extreme Albedo Dichotomy by Exogenically Triggered Thermal Ice Migration". Science. AAAS. 327 (5964): 432–5. Bibcode:2010Sci...327..432S. PMID 20007862. doi:10.1126/science.1177132. Retrieved 2009-12-19.
- ↑ Jones, Geraint H.; et al. (2008-03-07). "The Dust Halo of Saturn's Largest Icy Moon, Rhea". Science. AAAS. 319 (5868): 1380–1384. Bibcode:2008Sci...319.1380J. PMID 18323452. doi:10.1126/science.1151524.
- ↑ Lakdawalla, E. (2008-03-06). "A Ringed Moon of Saturn? Cassini Discovers Possible Rings at Rhea". The Planetary Society web site. Planetary Society. Archived from the original on March 10, 2008. Retrieved 2008-03-09.
- ↑ Lakdawalla, E. (5 October 2009). "Another possible piece of evidence for a Rhea ring". The Planetary Society Blog. Planetary Society. Retrieved 2009-10-06.
- ↑ Kerr, Richard A. (2010-06-25). "The Moon Rings That Never Were". ScienceNow. Archived from the original on 2010-07-01. Retrieved 2010-08-05.
- ↑ "Soft Collision (NASA Cassini Saturn Mission Images)". ciclops.org.
- ↑ Prometheus collision. YouTube. 18 November 2007.
- ↑ Saturn's G Ring. YouTube. 6 August 2007.
- ↑ "Rounding the Corner (NASA Cassini Saturn Mission Images)". ciclops.org.
External links
![]() |
Wikimedia Commons has media related to Rings of Saturn. |
![]() |
Wikimedia Commons has media related to Saturn (Rings). |
- Planetary Rings Node: Saturn's Ring System
- Saturn's Rings by NASA's Solar System Exploration
- Rings of Saturn nomenclature from the USGS planetary nomenclature page
- NASA finds giant ring around Saturn – Yahoo 7 news (retrieved 2009-10-07)
- Everything a Curious Mind Should Know About Planetary Ring Systems with Dr Mark Showalter (Waseem Akhtar podcast with Mark Showalter)
- High-resolution animation by Seán Doran of the backlit rings