Regeneration (biology)
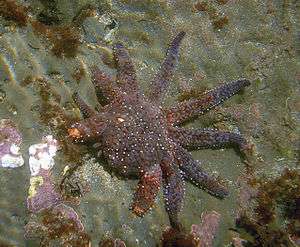

In biology, regeneration is the process of renewal, restoration, and growth that makes genomes, cells, organisms, and ecosystems resilient to natural fluctuations or events that cause disturbance or damage.[1] Every species is capable of regeneration, from bacteria to humans.[2][3] Regeneration can either be complete[4] where the new tissue is the same as the lost tissue,[4] or incomplete[5] where after the necrotic tissue comes fibrosis.[5] At its most elementary level, regeneration is mediated by the molecular processes of gene regulation.[6][7] Regeneration in biology, however, mainly refers to the morphogenic processes that characterize the phenotypic plasticity of traits allowing multi-cellular organisms to repair and maintain the integrity of their physiological and morphological states. Above the genetic level, regeneration is fundamentally regulated by asexual cellular processes.[8] Regeneration is different from reproduction. For example, hydra perform regeneration but reproduce by the method of budding.
The hydra and the planarian flatworm have long served as model organisms for their highly adaptive regenerative capabilities.[9] Once wounded, their cells become activated and start to remodel tissues and organs back to the pre-existing state.[10] The Caudata ("urodeles"; salamanders and newts), an order of tailed amphibians, is possibly the most adept vertebrate group at regeneration, given their capability of regenerating limbs, tails, jaws, eyes and a variety of internal structures.[2] The regeneration of organs is a common and widespread adaptive capability among metazoan creatures.[9] In a related context, some animals are able to reproduce asexually through fragmentation, budding, or fission.[8] A planarian parent, for example, will constrict, split in the middle, and each half generates a new end to form two clones of the original.[11] Echinoderms (such as the starfish), crayfish, many reptiles, and amphibians exhibit remarkable examples of tissue regeneration. The case of autotomy, for example, serves as a defensive function as the animal detaches a limb or tail to avoid capture. After the limb or tail has been autotomized, cells move into action and the tissues will regenerate.[12][13][14] Limited regeneration of limbs occurs in most fishes and salamanders, and tail regeneration takes place in larval frogs and toads (but not adults). The whole limb of a Salamander or a Triton will grow again and again after amputation. In reptiles, Chelonians, crocodiles and snakes are unable to regenerate lost parts, but many (not all) kinds of lizards, geckos and Iguanas possess regeneration capacity in a high degree. Usually, it involves dropping a section of their tail and regenerating it as part of a defense mechanism. While escaping a predator, if the predator catches the tail, it will disconnect.[15]
Ecosystems
Ecosystems can be regenerative. Following a disturbance, such as a fire or pest outbreak in a forest, pioneering species will occupy, compete for space, and establish themselves in the newly opened habitat. The new growth of seedlings and community assembly process is known as regeneration in ecology.[16][17]
Cellular molecular fundamentals
Pattern formation in the morphogenesis of an animal is regulated by genetic induction factors that put cells to work after damage has occurred. Neural cells, for example, express growth-associated proteins, such as GAP-43, tubulin, actin, an array of novel neuropeptides, and cytokines that induce a cellular physiological response to regenerate from the damage.[18] Many of the genes that are involved in the original development of tissues are reinitialized during the regenerative process. Cells in the primordia of zebrafish fins, for example, express four genes from the homeobox msx family during development and regeneration.[19]
Tissues
"Strategies include the rearrangement of pre-existing tissue, the use of adult somatic stem cells and the dedifferentiation and/or transdifferentiation of cells, and more than one mode can operate in different tissues of the same animal.[1] All these strategies result in the re-establishment of appropriate tissue polarity, structure and form."[20]:873 During the developmental process, genes are activated that serve to modify the properties of cell as they differentiate into different tissues. Development and regeneration involves the coordination and organization of populations cells into a blastema, which is "a mound of stem cells from which regeneration begins."[21] Dedifferentiation of cells means that they lose their tissue-specific characteristics as tissues remodel during the regeneration process. This should not be confused with the transdifferentiation of cells which is when they lose their tissue-specific characteristics during the regeneration process, and then re-differentiate to a different kind of cell.[20]
In animals
Arthropods
Arthropods are known to regenerate appendages following loss or autotomy.[22] Regeneration among arthropods is restricted by molting such that hemimetabolous insects are capable of regeneration only until their final molt whereas most crustaceans can regenerate throughout their lifetimes.[23] Molting cycles are hormonally regulated in arthropods,[24] although premature molting can be induced by autotomy.[22] Mechanisms underlying appendage regeneration in hemimetabolous insects and crustaceans is highly conserved.[25] During limb regeneration species in both taxa form a blastema[26] following autotomy with regeneration of the excised limb occurring during proecdysis.[24] Arachnids, including scorpions, are known to regenerate their venom, although the content of the regenerated venom is different than the original venom during its regeneration, as the venom volume is replaced before the active proteins are all replenished.[27]
Annelids
Many annelids are capable of regeneration.[28] For example, Chaetopterus variopedatus and Branchiomma nigromaculata can regenerate both anterior and posterior body parts after latitudinal bisection.[29] The relationship between somatic and germline stem cell regeneration has been studied at the molecular level in the annelid Capitella teleta.[30] Leeches, members of the Annelid subclass Hirudinid, are incapable of segmental regeneration.[31] Furthermore, their close relatives, the branchiobdellids, are also incapable of segmental regeneration.[31][32] However, certain individuals, like the lumbriculids, can regenerate from only a few segments.[31] Segmental regeneration in these animals is epimorphic and occurs through blastema formation.[31] Segmental regeneration has been gained and lost during annelid evolution, as seen in oligochaetes, where head regeneration has been lost three separate times.[31]
Along with epimorphosis, some polychaetes like Sabella pavonina experience morphallactic regeneration.[31][33] Morphallaxis involves the de-differentiation, transformation, and re-differentation of cells to regenerate tissues. How prominent morphallactic regeneration is in oligochaetes is currently not well understood. Although relatively under-reported, it is possible that morphallaxis is a common mode of inter-segment regeneration in annelids. Following regeneration in L. variegatus, past posterior segments sometimes become anterior in the new body orientation, consistent with morphallaxis.
Following amputation, most annelids are capable of sealing their body via rapid muscular contraction. Constriction of body muscle can lead to infection prevention. In certain species, like Limnodrilus, autolysis can be seen within hours after amputation in the ectoderm and mesoderm. Amputation is also thought to cause a large migration of cells to the injury site, and these form a wound plug.
Echinoderms
Tissue regeneration is widespread among echinoderms and has been well documented in starfish (Asteroidea), sea cucumbers (Holothuroidea), and sea urchins (Echinoidea). Appendage regeneration in echinoderms has been studied since at least the 19th century.[34] In addition to appendages, some species can regenerate internal organs and parts of their central nervous system.[35] In response to injury starfish can autotomize damaged appendages. Autotomy is the self-amputation of a body part, usually an appendage. Depending on severity, starfish will then go through a four-week process where the appendage will be regenerated.[36] Some species must retain mouth cells in order to regenerate an appendage, due to the need for energy.[37] The first organs to regenerate, in all species documented to date, are associated with the digestive tract. Thus, most of our knowledge about visceral regeneration in holothurians concerns this system.[38]
Planaria (Platyhelminthes)
Regeneration research using Planarians began in the late 1800s and was popularized by T.H. Morgan at the beginning of the 19th century.[37] Alejandro Sanchez-Alvarado and Philip Newmark transformed planarians into a model genetic organism in the beginning of the 20th century to study the molecular mechanisms underlying regeneration in these animals.[39] Planarians exhibit an extraordinary ability to regenerate lost body parts. For example, a planarian split lengthwise or crosswise will regenerate into two separate individuals. In one experiment, T.H. Morgan found that a piece corresponding to 1/279th of a planarian[37] or a fragment with as few as 10,000 cells can successfully regenerate into a new worm within one to two weeks.[40] After amputation, stump cells form a blastema formed from neoblasts, pluripotent cells found throughout the planarian body.[41] New tissue grows from neoblasts with neoblasts comprising between 20 and 30% of all planarian cells.[40] Recent work has confirmed that neoblasts are totipotent since one single noblest can regenerate an entire irradiated animal that has been rendered incapable of regeneration.[42] In order to prevent starvation a planarian will use their own cells for energy, this phenomenon is known as de-growth.[43]
Amphibians
Limb regeneration in the axolotl and newt has been extensively studied and researched. Urodele amphibians, such as salamanders and newts, display the highest regenerative ability among tetrapods.[44] As such, they can fully regenerate their limbs, tail, jaws, and retina via epimorphic regeneration leading to functional replacement of new tissue.[45] Salamander limb regeneration occurs in two main steps. First, the local cells dedifferentiate at the wound site into progenitor to form a blastema[46] Second, the blastemal cells will undergo proliferation, patterning, differentiation and growth using similar genetic mechanisms that deployed during embryonic development.[47] Ultimately, blastemal cells will generate all the cells for the new structure.[48]

After amputation, the epidermis migrates to cover the stump in 1–2 hours, forming a structure called the wound epithelium (WE).[49] Epidermal cells continue to migrate over the WE, resulting in a thickened, specialized signaling center called the apical epithelial cap (AEC).[50] Over the next several days there are changes in the underlying stump tissues that result in the formation of a blastema (a mass of dedifferentiated proliferating cells). As the blastema forms, pattern formation genes – such as HoxA and HoxD – are activated as they were when the limb was formed in the embryo.[51][52] The positional identity of the distal tip of the limb (i.e. the autopod, which is the hand or foot) is formed first in the blastema. Intermediate positional identities between the stump and the distal tip are then filled in through a process called intercalation.[51] Motor neurons, muscle, and blood vessels grow with the regenerated limb, and reestablish the connections that were present prior to amputation. The time that this entire process takes varies according to the age of the animal, ranging from about a month to around three months in the adult and then the limb becomes fully functional. Researchers at Australian Regenerative Medicine Institute at Monash University, have published that when macrophages, which eat up material debris,[53] were removed, salamanders lost their ability to regenerate and formed scarred tissue instead.[54]
In spite of the historically few researchers studying limb regeneration, remarkable progress has been made recently in establishing the neotenous amphibian the axolotl (Ambystoma mexicanum) as a model genetic organism. This progress has been facilitated by advances in genomics, bioinformatics, and somatic cell transgenesis in other fields, that have created the opportunity to investigate the mechanisms of important biological properties, such as limb regeneration, in the axolotl.[47] The Ambystoma Genetic Stock Center (AGSC) is a self-sustaining, breeding colony of the axolotl supported by the National Science Foundation as a Living Stock Collection. Located at the University of Kentucky, the AGSC is dedicated to supplying genetically well-characterized axolotl embryos, larvae, and adults to laboratories throughout the United States and abroad. An NIH-funded NCRR grant has led to the establishment of the Ambystoma EST database, the Salamander Genome Project (SGP) that has led to the creation of the first amphibian gene map and several annotated molecular data bases, and the creation of the research community web portal.[55]
Anurans can only regenerate their limbs during embryonic development.[56] Once the limb skeleton has developed regeneration does not occur (Xenopus can grow a cartilaginous spike after amputation).[56] Reactive oxygen species (ROS) appear to be required for a regeneration response in the anuran larvae.[57] ROS production is essential to activate the Wnt signaling pathway, which has been associated with regeneration in other systems.[58] Limb regeneration in salamanders occurs in two major steps. First, adult cells de-differentiate into progenitor cells which will replace the tissues they are derived from.[59][60] Second, these progenitor cells then proliferate and differentiate until they have completely replaced the missing structure.[61]
Hydra
Hydra is a genus of freshwater polyp in the phylum Cnidaria with highly proliferative stem cells that gives them the ability to regenerate their entire body.[62] Any fragment larger than a few hundred epithelial cells that is isolated from the body has the ability to regenerate into a smaller version of itself.[62] The high proportion of stem cells in the hydra supports its efficient regenerative ability.[63]
Regeneration among hydra occurs as foot regeneration arising from the basal part of the body, and head regeneration, arising from the apical region.[62] Regeneration tissues that are cut from the gastric region contain polarity, which allows them to distinguish between regenerating a head in the apical end and a foot in the basal end so that both regions are present in the newly regenerated organism.[62] Head regeneration requires complex reconstruction of the area, while foot regeneration is much simpler, similar to tissue repair.[64] In both foot and head regeneration, however, there are two distinct molecular cascades that occur once the tissue is wounded: early injury response and a subsequent, signal-driven pathway of the regenerating tissue that leads to differentiation.[63] This early-injury response includes epithelial cell stretching for wound closure, the migration of interstitial progenitors towards the wound, cell death, phagocytosis of cell debris, and reconstruction of the extracellular matrix.[63]
Regeneration in hydra has been defined as morphallaxis, the process where regeneration results from remodeling of existing material without cellular proliferation.[65][66] If a hydra is cut into two pieces, the remaining severed sections form two fully functional and independent hydra, approximately the same size as the two smaller severed sections.[62] This occurs through the exchange and rearrangement of soft tissues without the formation of new material.[63]
Aves (birds)
Owing to a limited literature on the subject, birds are believed to have very limited regenerative abilities as adults. Some studies[67] on roosters have suggested that birds can adequately regenerate some parts of the limbs and depending on the conditions in which regeneration takes place, such as age of the animal, the inter-relationship of the injured tissue with other muscles, and the type of operation, can involve complete regeneration of some musculoskeletal structure. Werber and Goldschmidt (1909) found that the goose and duck were capable of regenerating their beaks after partial amputation[67] and Sidorova (1962) observed liver regeneration via hypertrophy in roosters.[68] Birds are also capable of regenerating the hair cells in their cochlea following noise damage or ototoxic drug damage.[69] Despite this evidence, contemporary studies suggest reparative regeneration in avian species is limited to periods during embryonic development. An array of molecular biology techniques have been successful in manipulating cellular pathways known to contribute to spontaneous regeneration in chick embryos.[70] For instance, removing a portion of the elbow joint in a chick embryo via window excision or slice excision and comparing joint tissue specific markers and cartilage markers showed that window excision allowed 10 out of 20 limbs to regenerate and expressed joint genes similarly to a developing embryo. In contrast, slice excision did not allow the joint to regenerate due to the fusion of the skeletal elements seen by an expression of cartilage markers.[71]
Similar to the physiological regeneration of hair in mammals, birds can regenerate their feathers in order to repair damaged feathers or to attract mates with their plumage. Typically, seasonal changes that are associated with breeding seasons will prompt a hormonal signal for birds to begin regenerating feathers. This has been experimentally induced using thyroid hormones in the Rhode Island Red Fowls.[72]
Mammals
Mammals are capable of cellular and physiological regeneration, but have generally poor reparative regenerative ability across the group.[1][73] Examples of physiological regeneration in mammals include epithelial renewal (e.g., skin and intestinal tract), red blood cell replacement, antler regeneration and hair cycling.[74][75] Male deer lose their antlers annually during the months of January to April then through regeneration are able to regrow them as an example of physiological regeneration. A deer antler is the only appendage of a mammal that can be regrown every year.[76] While reparative regeneration is a rare phenomenon in mammals, it does occur. A well-documented example is regeneration of the digit tip distal to the nail bed.[77] Reparative regeneration has also been observed in rabbits, pikas and African spiny mice. In 2012, researchers discovered that at least two species of African Spiny Mice, Acomys kempi and Acomys percivali, are capable of completely regenerating the autotomically released or otherwise damaged tissue. These species can regrow hair follicles, skin, sweat glands, fur and cartilage.[78]
Adult mammals have limited regenerative capacity compared to most vertebrate embryos/larvae, adult salamanders and fish.[79] But the regeneration therapy approach of Robert O. Becker, using electrical stimulation, has shown promising results for rats [80] and mammals in general.[81]
The MRL mouse is a strain of mouse that exhibits remarkable regenerative abilities for a mammal. By comparing the differential gene expression of scarless healing MRL mice and a poorly-healing C57BL/6 mouse strain, 36 genes have been identified that are good candidates for studying how the healing process differs in MRL mice and other mice.[82][83] Study of the regenerative process in these animals is aimed at discovering how to duplicate them in humans, such as deactivation of the p21 gene.[84][85]
The regenerative ability of MRL mice does not, however, protect them against myocardial infarction; heart regeneration in adult mammals (neocardiogenesis) is limited, because heart muscle cells are nearly all terminally differentiated. MRL mice show the same amount of cardiac injury and scar formation as normal mice after a heart attack.[86] However, recent studies provide evidence that this may not always be the case, and that MRL mice can regenerate after heart damage.
Humans
The regrowth of lost tissues or organs in the human body is being researched. Some tissues such as skin regrow quite readily; others have been thought to have little or no capacity for regeneration, but ongoing research suggests that there is some hope for a variety of tissues and organs.[1][87] Human organs that have been regenerated include the bladder, vagina and the penis.[88]
As are all metazoans, humans are capable of physiological regeneration (i.e. the replacement of cells during homeostatic maintenance that does not necessitate injury). For example, the regeneration of red blood cells via erythropoiesis occurs through the maturation of erythrocytes from hematopoietic stem cells in the bone marrow, their subsequent circulation for around 90 days in the blood stream, and their eventual cell-death in the spleen.[89] Another example of physiological regeneration is the sloughing and rebuilding of a functional endometrium during each menstrual cycle in females in response to varying levels of circulating estrogen and progesterone.[90]
However, humans are limited in their capacity for reparative regeneration, which occurs in response to injury. One of the most studied regenerative responses in humans is the hypertrophy of the liver following liver injury.[91][92] For example, the original mass of the liver is re-established in direct proportion to the amount of liver removed following partial hepatectomy,[93] which indicates that signals from the body regulate liver mass precisely, both positively and negatively, until the desired mass is reached. This response is considered cellular regeneration (a form of compensatory hypertrophy) where the function and mass of the liver is regenerated through the proliferation of existing mature hepatic cells (mainly hepatocytes), but the exact morphology of the liver is not regained.[92] This process is driven by growth factor and cytokine regulated pathways.[91]
Adult neurogenesis is also a form of cellular regeneration. For example, hippocampal neuron renewal occurs in normal adult humans at an annual turnover rate of 1.75% of neurons.[94] Cardiac myocyte renewal has been found to occur in normal adult humans,[95] and at a higher rate in adults following acute heart injury such as infarction.[96] Even in adult myocardium following infarction, proliferation is only found in around 1% of myocytes around the area of injury, which is not enough to restore function of cardiac muscle. However, this may be an important target for regenerative medicine as it implies that regeneration of cardiomyocytes, and consequently of myocardium, can be induced.
Another example of reparative regeneration in humans is fingertip regeneration, which occurs after phalange amputation distal to the nail bed (especially in children)[97][98] and rib regeneration, which occurs following osteotomy for scoliosis treatment (though usually regeneration is only partial and may take up to 1 year).[99]
Reptiles
The ability and degree of regeneration in reptiles differs among the various species, but the most notable and well-studied occurrence is tail-regeneration in lizards.[100][101][102] In addition to lizards, regeneration has been observed in the tails and maxillary bone of crocodiles and adult neurogenesis has also been noted.[100][103][104] Tail regeneration has never been observed in snakes.[100] Lizards possess the highest regenerative capacity as a group.[100][101][102][105] Following autonomous tail loss, epimorphic regeneration of a new tail proceeds through a blastema-mediated process that results in a functionally and morphologically similar structure.[100][101] Tail regeneration in lizards presents an interesting case study for the evolution of regenerative abilities as regenerative ability among the 3,300 extant species varies greatly among them.[100][106]
Chondrichthyes
Studies have shown that some chondrichthyans can regenerate rhodopsin by cellular regeneration,[107] micro RNA organ regeneration,[108] teeth physiological teeth regeneration,[109] and reparative skin regeneration.[110] Rhodopsin regeneration has been studied in skates and rays.[107] After complete photo-bleaching, rhodopsin can completely regenerate within 2 hours in the retina.[107] White bamboo sharks can regenerate at least two-thirds of their liver and this has been linked to three micro RNAs, xtr-miR-125b, fru-miR-204, and has-miR-142-3p_R-.[108] In one study two thirds of the liver was removed and within 24 hours more than half of the liver had undergone hypertrophy.[108] Leopard sharks routinely replace their teeth every 9–12 days [109] and this is an example of physiological regeneration. This can occur because shark teeth are not attached to a bone, but instead are developed within a bony cavity.[109] It has been estimated that the average shark looses about 30,000 to 40,000 teeth in a lifetime.[109] Some sharks can regenerate scales and even skin following damage.[110] Within two weeks of skin wounding the mucus is secreted into the wound and this initiates the healing process.[110] One study showed that the majority of the wounded area was regenerated within 4 months, but the regenerated area also showed a high degree of variability.[110]
See also
Notes
- 1 2 3 4 Birbrair, Alexander; Zhang, Tan; Wang, Zhong-Min; Messi, Maria Laura; Enikolopov, Grigori N.; Mintz, Akiva; Delbono, Osvaldo (2013-03-21). "Role of Pericytes in Skeletal Muscle Regeneration and Fat Accumulation". Stem Cells and Development. 22 (16): 2298–2314. ISSN 1547-3287. PMC 3730538
. PMID 23517218. doi:10.1089/scd.2012.0647.
- 1 2 Carlson, B. M. (2007). Principles of Regenerative Biology. Elsevier Inc. p. 400. ISBN 978-0-12-369439-3.
- ↑ Gabor, M. H.; Hotchkiss, R. D. (1979). "Parameters governing bacterial regeneration and genetic recombination after fusion of Bacillus subtilis protoplasts". Journal of Bacteriology. 137 (3): 1346–1353. PMC 218319
. PMID 108246.
- 1 2 Min, Su; Wang, Song W.; Orr, William (2006). "Graphic general pathology: 2.2 complete regeneration:". Pathology. pathol.med.stu.edu.cn. Retrieved 2012-12-07.
(1) Complete regeneration: The new tissue is the same as the tissue that was lost. After the repair process has been completed, the structure and function of the injured tissue are completely normal
- 1 2 Min, Su; Wang, Song W.; Orr, William (2006). "Graphic general pathology: 2.3 Incomplete regeneration:". Pathology. pathol.med.stu.edu.cn. Retrieved 2012-12-07.
The new tissue is not the same as the tissue that was lost. After the repair process has been completed, there is a loss in the structure or function of the injured tissue. In this type of repair, it is common that granulation tissue (stromal connective tissue) proliferates to fill the defect created by the necrotic cells. The necrotic cells are then replaced by scar tissue.
- ↑ Himeno, Y.; Engelman, R. W.; Good, R. A. (1992). "Influence of calorie restriction on oncogene expression and DNA synthesis during liver regeneration". Proceedings of the National Academy of Sciences of the United States of America. 89 (12): 5497–5501. Bibcode:1992PNAS...89.5497H. PMC 49319
. PMID 1608960. doi:10.1073/pnas.89.12.5497.
- ↑ Bryant, P. J.; Fraser, S. E. (1988). "Wound healing, cell communication, and DNA synthesis during imaginal disc regeneration in Drosophila". Developmental Biology. 127 (1): 197–208. PMID 2452103. doi:10.1016/0012-1606(88)90201-1.
- 1 2 Brokes, J. P.; Kumar, A. "Comparative Aspects of Animal Regeneration". Annu. Rev. Cell Dev. Biol.. 28: 525–549. doi:10.1146/annurev.cellbio.24.110707.175336.
- 1 2 Sánchez, A. A. (2000). "Regeneration in the metazoans: why does it happen?" (PDF). BioEssays. 22 (6): 578–590. PMID 10842312. doi:10.1002/(SICI)1521-1878(200006)22:6<578::AID-BIES11>3.0.CO;2-#.
- ↑ Reddien, P. W.; Alvarado, A. S. (2004). "Fundamentals of planarian regenerations". Annual Review of Cell and Developmental Biology. 20: 725–757. PMID 15473858. doi:10.1146/annurev.cellbio.20.010403.095114.
- ↑ Campbell, N. A. Biology (4th ed.). California: The Benjamin Cummings Publishing Company, Inc. p. 1206.
- ↑ Wilkie, I. (2001). "Autotomy as a prelude to regeneration in echinoderms". Microscopy Research and Technique. 55 (6): 369–396. PMID 11782069. doi:10.1002/jemt.1185.
- ↑ Maiorana, V. C. (1977). "Tail autotomy, functional conflicts and their resolution by a salamander". Nature. 2265 (5594): 533–535. Bibcode:1977Natur.265..533M. doi:10.1038/265533a0.
- ↑ Maginnis, T. L. (2006). "The costs of autotomy and regeneration in animals: a review and framework for future research". Behavioural Ecology. 7 (5): 857–872. doi:10.1093/beheco/arl010.
- ↑ "UCSB Science Line". scienceline.ucsb.edu. Retrieved 2015-11-02.
- ↑ Dietze, M. C.; Clark, J. S. (2008). "Changing the gap dynamics paradigm: Vegetative regenerative control on forest response to disturbance" (PDF). Ecological Monographs. 78 (3): 331–347. doi:10.1890/07-0271.1.
- ↑ Bailey, J. D.; Covington, W. W. (2002). "Evaluation ponderosa pine regeneration rates following ecological restoration treatments in northern Arizona, USA" (PDF). Forest Ecology and Management. 155: 271–278. doi:10.1016/S0378-1127(01)00564-3.
- ↑ Fu, S. Y.; Gordon, T. (1997). "The cellular and molecular basis of peripheral nerve regeneration". Molecular Neurobiology. 14 (1–2): 67–116. PMID 9170101. doi:10.1007/BF02740621.
- ↑ Akimenko, M.; Johnson, S. L.; Wseterfield, M.; Ekker, M. (1996). "Differential induction of four msx homeobox genes during fin development and regeneration in zebrafish" (PDF). Development. 121 (2): 347–357. PMID 7768177.
- 1 2 Alvarado, A. S.; Tsonis, P. A. (2006). "Bridging the regeneration gap: genetic insights from diverse animal models" (PDF). Nat. Rev. Genet. 7 (11): 873–884. PMID 17047686. doi:10.1038/nrg1923.
- ↑ Kumar, A.; Godwin, J. W.; Gates, P. B.; Garza-Garcia, A. A.; Brokes, J. P. (2007). "Molecular Basis for the Nerve Dependence of Limb Regeneration in an Adult Vertebrate". Science. 318 (5851): 772–7. Bibcode:2007Sci...318..772K. PMC 2696928
. PMID 17975060. doi:10.1126/science.1147710.
- 1 2 Integument, Pigments, and Hormonal Processes: Volume 9: Integument, Pigments and Hormonal Processes. Academic Press. 2012-12-02. ISBN 9780323139229.
- ↑ Seifert, Ashley W.; Monaghan, James R.; Smith, Matthew D.; Pasch, Bret; Stier, Adrian C.; Michonneau, François; Maden, Malcolm (2012-05-01). "The influence of fundamental traits on mechanisms controlling appendage regeneration". Biological Reviews. 87 (2): 330–345. ISSN 1469-185X. PMID 21929739. doi:10.1111/j.1469-185X.2011.00199.x.
- 1 2 Travis, Dorothy F. (1955-02-01). "The Molting Cycle of the Spiny Lobster, Panulirus argus Latreille. II. Pre-Ecdysial Histological and Histochemical Changes in the Hepatopancreas and Integumental Tissues". Biological Bulletin. 108 (1): 88–112. JSTOR 1538400. doi:10.2307/1538400.
- ↑ Das, Sunetra (2015-11-01). "Morphological, Molecular, and Hormonal Basis of Limb Regeneration across Pancrustacea". Integrative and Comparative Biology. 55 (5): 869–877. ISSN 1540-7063. PMID 26296354. doi:10.1093/icb/icv101.
- ↑ Hamada, Yoshimasa; Bando, Tetsuya; Nakamura, Taro; Ishimaru, Yoshiyasu; Mito, Taro; Noji, Sumihare; Tomioka, Kenji; Ohuchi, Hideyo (2015-09-01). "Leg regeneration is epigenetically regulated by histone H3K27 methylation in the cricket Gryllus bimaculatus". Development. 142 (17): 2916–2927. ISSN 0950-1991. PMID 26253405. doi:10.1242/dev.122598.
- ↑ Nisani, Zia; Dunbar, Stephen G.; Hayes, William K. (2007-06-01). "Cost of venom regeneration in Parabuthus transvaalicus (Arachnida: Buthidae)". Comparative Biochemistry and Physiology A. 147 (2): 509–513. ISSN 1095-6433. PMID 17344080. doi:10.1016/j.cbpa.2007.01.027.
- ↑ Bely AE (August 2006). "Distribution of segment regeneration ability in the Annelida". Integr. Comp. Biol. 46 (4): 508–18. PMID 21672762. doi:10.1093/icb/icj051.
- ↑ Hill SD (December 1972). "Caudal regeneration in the absence of a brain in two species of sedentary polychaetes". J Embryol Exp Morphol. 28 (3): 667–80. PMID 4655324.
- ↑ Giani VC, Yamaguchi E, Boyle MJ, Seaver EC (2011). "Somatic and germline expression of piwi during development and regeneration in the marine polychaete annelid Capitella teleta". Evodevo. 2: 10. PMC 3113731
. PMID 21545709. doi:10.1186/2041-9139-2-10.
- 1 2 3 4 5 6 Zoran, Mark J (2001-01-01). Regeneration in Annelids. John Wiley & Sons, Ltd. ISBN 9780470015902. doi:10.1002/9780470015902.a0022103.
- ↑ Bely, Alexandra E. (2006-06-30). "Distribution of segment regeneration ability in the Annelida". Integrative and Comparative Biology. 46 (4): 508–518. ISSN 1540-7063. PMID 21672762. doi:10.1093/icb/icj051.
- ↑ Bely, Alexandra E. (2014-10-01). "Early Events in Annelid Regeneration: A Cellular Perspective". Integrative and Comparative Biology. 54 (4): 688–699. ISSN 1540-7063. PMID 25122930. doi:10.1093/icb/icu109.
- ↑ Carnevali, M. D. Candia; Bonasoro, F.; Patruno, M.; Thorndyke, M. C. (1998-10-01). "Cellular and molecular mechanisms of arm regeneration in crinoid echinoderms: the potential of arm explants". Development Genes and Evolution. 208 (8): 421–430. ISSN 0949-944X. doi:10.1007/s004270050199.
- ↑ San Miguel-Ruiz, José E.; Maldonado-Soto, Angel R.; García-Arrarás, José E. (2009-01-01). "Regeneration of the radial nerve cord in the sea cucumber Holothuria glaberrima". BMC Developmental Biology. 9: 3. ISSN 1471-213X. PMC 2640377
. PMID 19126208. doi:10.1186/1471-213X-9-3.
- ↑ Patruno, M.; Thorndyke, M. C.; Carnevali, M. D. Candia; Bonasoro, F.; Beesley, P. W. (2001-03-01). "Growth factors, heat-shock proteins and regeneration in echinoderms". Journal of Experimental Biology. 204 (5): 843–848. ISSN 0022-0949. PMID 11171408.
- 1 2 3 Morgan, T.H. (1900). "Regeneration in Planarians". Eingegangen.
- ↑ García-Arrarás, J. E.; Greenberg, M. J. (2001-12-15). "Visceral regeneration in holothurians". Microscopy Research and Technique. 55 (6): 438–451. ISSN 1059-910X. PMID 11782073. doi:10.1002/jemt.1189.
- ↑ Alvarado, Alejandro-Sánchez; Newmark, Phillip A. (1998). "The use of planarians to dissect the molecular basis of metazoan regeneration". Wound repair and regeneration. 6 (4): S413.
- 1 2 Montgomery, JR; Coward, SJ (1974). "On the minimal size of a planarian capable of regeneration". Trans Am Mic Sci. 93: 386–391.
- ↑ "The history and enduring contributions of planarians to the study of animal regeneration". Wiley Interdisciplinary Reviews: Developmental Biology. 2: 301–326. doi:10.1002/wdev.82.
- ↑ Wagner, D. E.; Wang, I. E.; Reddien, P. W. "Clonogenic Neoblasts Are Pluripotent Adult Stem Cells That Underlie Planarian Regeneration". Science. 2011: 811–16.
- ↑ Reddien, P; Sánchez Alvarado, A (2004). "Fundamentals of planarian regeneration". Annu Rev Cell Dev Biol. 20: 725–757. PMID 15473858. doi:10.1146/annurev.cellbio.20.010403.095114.
- ↑ Brockes, Kumar, Velloso, Jeremy, Anoop, Christina (2001). Regeneration as an Evolutionary Variable. Anatomical Society of Great Britain and Ireland.
- ↑ Brockes, Kumar, Jeremy, Anoop (August 2002). "Plasticity and reprogramming of differentiated cells in amphibian regeneration". Nature Reviews Molecular Cellular Biology.
- ↑ Iten, Bryant, Laurie, Susan (December 1973). "Forelimb regeneration from different levels of amputation in the newt,Notophthalmus viridescens: Length, rate, and stages". University of California Irvine.
- 1 2 Endo T, Bryant SV, Gardiner DM (June 2004). "A stepwise model system for limb regeneration". Developmental Biology. 270 (1): 135–45. PMID 15136146. doi:10.1016/j.ydbio.2004.02.016.
- ↑ Brockes, Kumar, Velloso, Jeremy, Anoop, Christina (August 2001). "Regeneration as an evolutionary variable". Journal of Anatomy.
- ↑ Satoh, A; Bryant, SV; Gardiner, DM (2012). "Nerve signaling regulates basal keratinocyte proliferation in the blastema apical epithelial cap in the axolotl (Ambystoma mexicanum).". Dev Biol. 366: 374–381. PMID 22537500. doi:10.1016/j.ydbio.2012.03.022.
- ↑ Christensen, RN; Tassava, RA (2000). "Apical epithelial cap morphology and fibronectin gene expression in regenerating axolotl limbs.". Dev Dyn. 217 (2): 216–224. PMID 10706145. doi:10.1002/(sici)1097-0177(200002)217:2<216::aid-dvdy8>3.0.co;2-8.
- 1 2 Bryant SV, Endo T, Gardiner DM (2002). "Vertebrate limb regeneration and the origin of limb stem cells". The International journal of developmental biology. 46 (7): 887–96. PMID 12455626.
- ↑ Mullen LM, Bryant SV, Torok MA, Blumberg B, Gardiner DM (November 1996). "Nerve dependency of regeneration: the role of Distal-less and FGF signaling in amphibian limb regeneration". Development (Cambridge, England). 122 (11): 3487–97. PMID 8951064.
- ↑ Souppouris, Aaron (2013-05-23). "Scientists identify cell that could hold the secret to limb regeneration". the verge.com.
Macrophages are a type of repairing cell that devour dead cells and pathogens, and trigger other immune cells to respond to pathogens.
- ↑ "Do Salamanders' Immune Systems Hold the Key to Regeneration?". ScienceDaily. Retrieved 21 May 2013.
- ↑ http://www.ambystoma.org
- 1 2 Korneluk, Liversage, Robert, Richard (February 6, 2005). "Regenerative response of amputated forelimbs of Xenopus laevis froglets to partial denervation". Journal of Morphology.
- ↑ Reya, Clevers, Tannishtha, Hans (2005-04-15). "Wnt signalling in stem cells and cancer". International Weekly Journal of Science.
- ↑ Reya, Clevers, Tannishtha, Hans (2005-04-14). "Wnt signalling in stem cells and cancer". International Weekly Journal of Science.
- ↑ Kragl, M (2009). "Cells keep a memory of their tissue origin during axolotl limb regeneration.". Nature. 460 (7251): 60–65. PMID 19571878. doi:10.1038/nature08152.
- ↑ Muneoka, K (1986). "Cellular contribution from dermis and cartilage to the regenerating limb blastema in axolotls.". Dev Biol. 116 (1): 256–260. PMID 3732605. doi:10.1016/0012-1606(86)90062-x.
- ↑ Bryant, S (2002). "Vertebrate limb regeneration and the origin of limb stem cells". Int. J. Dev. Biol. 46 (7): 887–896. PMID 12455626.
- 1 2 3 4 5 Bosch, Thomas C.G. (2007). "Why Polyps Regenerate and We Don't: Towards a Cellular and Molecular Framework for Hydra Regeneration". Developmental Biology. Elsevier. 303: 421–33. PMID 17234176. doi:10.1016/j.ydbio.2006.12.012.
- 1 2 3 4 Wenger, Yvan; Buzgariu, Wanda; Reiter, Silke; Galliot, Brigitte (2014). "Injury-Induced Immune Responses in Hydra". Seminars in Immunology. Elsevier. 26.4: 277–94. doi:10.1016/j.smim.2014.06.004.
- ↑ Buzgariu, Wanda; Crescenzi, Marco; Galliot, Brigitte (2014). Science Direct. "Robust G2 Pausing of Adult Stem Cells in Hydra". Differentiation. Elsevier. 87.1-2: 83–99.
- ↑ T.H. Morgan (1901). Regeneration.
- ↑ Agata, Kiyokazu (2007). "Unifying Principles of Regeneration I: Epimorphosis versus Morphallaxis". Department of Biophysics. Wiley Online Library. Kyoto University.
- 1 2 Vorontsova, M.A.; Liosner, L.D. (1960). Asexual Reproduction and Regeneration. London: Pergamon Press. pp. 367–371.
- ↑ Sidorova, V. F. (1962-07-01). "Liver regeneration in birds". Bulletin of Experimental Biology and Medicine. 52 (6): 1426–1429. ISSN 0007-4888. doi:10.1007/BF00785312.
- ↑ Cotanche, Douglas A.; Lee, Kenneth H.; Stone, Jennifer S.; Picard, Daniel A. (1994-01-01). "Hair cell regeneration in the bird cochlea following noise damage or ototoxic drug damage". Anatomy and Embryology. 189 (1): 1–18. ISSN 0340-2061. doi:10.1007/BF00193125.
- ↑ Coleman, Cynthia M. (2008-09-01). "Chicken embryo as a model for regenerative medicine". Birth Defects Research Part C: Embryo Today: Reviews. 84 (3): 245–256. ISSN 1542-9768. doi:10.1002/bdrc.20133.
- ↑ Özpolat, B. Duygu; Zapata, Mariana; Daniel Frugé, John; Coote, Jeffrey; Lee, Jangwoo; Muneoka, Ken; Anderson, Rosalie (2012-12-15). "Regeneration of the elbow joint in the developing chick embryo recapitulates development". Developmental Biology. 372 (2): 229–238. PMC 3501998
. PMID 23036343. doi:10.1016/j.ydbio.2012.09.020.
- ↑ Hosker, Anne (1936). "Regeneration of Feathers after Thyroid Feeding". Journal of Experimental Biology.
- ↑ Seifert, Ashley (2012). "The influence of fundamental traits on mechanisms controlling appendage regeneration.". Biological Reviews.
- ↑ Kresie, Lesley (2001). "Artificial blood: an update on current red cell and platelet substitutes". Baylor Health Care. 14: 158–61. PMC 1291332
. PMID 16369608.
- ↑ Li, Chunyi (2013). "Morphogenetic Mechanisms in the Cyclic Regeneration of Hair Follicles and Deer Antlers from Stem Cells". Biomed Resouces International. 2013: 643601. PMC 3870647
. PMID 24383056. doi:10.1155/2013/643601.
- ↑ Price, J (2004). "Exploring the Mechanisms Regulating Regeneration of Deer Antlers". The Royal Society.
- ↑ Fernando (2011). "Wound healing and blastema formation in regenerating digit tips of adult mice.". Developmental Biology.
- ↑ Seifert, A.W.; et al. (2012). "Skin shedding and tissue regeneration in African spiny mice (Acomys)". Nature. 489: 561–565. PMC 3480082
. PMID 23018966. doi:10.1038/nature11499. Retrieved 2012-01-24.
- ↑ http://www.businessinsider.com/how-regeneration-works-2013-7
- ↑ Becker RO (Jan 1972). "Stimulation of Partial Limb Regeneration in Rats" (PDF). Nature. 235 (14): 109–111. Bibcode:1972Natur.235..109B. doi:10.1038/235109a0.
- ↑ Becker RO (May 1972). "Electrical stimulation of partial limb regeneration in mammals". Bull N Y Acad Med. 48 (4): 627–41. PMC 1806700
. PMID 4503923.
- ↑ Masinde G, Li X, Baylink DJ, Nguyen B, Mohan S (April 2005). "Isolation of wound healing/regeneration genes using restrictive fragment differential display-PCR in MRL/MPJ and C57BL/6 mice". Biochemical and Biophysical Research Communications. 330 (1): 117–22. PMID 15781240. doi:10.1016/j.bbrc.2005.02.143.
- ↑ Mansuo L. Hayashi, B. S. Shankaranarayana Rao, Jin-Soo Seo, Han-Saem Choi, Bridget M. Dolan, Se-Young Choi, Sumantra Chattarji, and Susumu Tonegawa; Rao; Seo; Choi; Dolan; Choi; Chattarji; Tonegawa (July 2007). "Inhibition of p21-activated kinase rescues symptoms of fragile X syndrome in mice". Proceedings of the National Academy of Sciences. 104 (27): 11489–94. Bibcode:2007PNAS..10411489H. PMC 1899186
. PMID 17592139. doi:10.1073/pnas.0705003104.
- ↑ Bedelbaeva K, Snyder A, Gourevitch D, Clark L, Zhang X-M, Leferovich J, Cheverud JM, Lieberman P, Heber-Katz E; Snyder; Gourevitch; Clark; Zhang; Leferovich; Cheverud; Lieberman; Heber-Katz (March 2010). "Lack of p21 expression links cell cycle control and appendage regeneration in mice". Proceedings of the National Academy of Sciences. 107 (11): 5845–50. Bibcode:2010PNAS..107.5845B. PMC 2851923
. PMID 20231440. doi:10.1073/pnas.1000830107. Lay summary – PhysOrg.com.
- ↑ Humans Could Regenerate Tissue Like Newts By Switching Off a Single Gene
- ↑ Abdullah I, Lepore JJ, Epstein JA, Parmacek MS, Gruber PJ (March–April 2005). "MRL mice fail to heal the heart in response to ischemia-reperfusion injury". Wound Repair Regen. 13 (2): 205–208. PMID 15828946. doi:10.1111/j.1067-1927.2005.130212.x.
- ↑ Min, Su; Wang, Song W.; Orr, William (2006). "Graphic general pathology: 2.2 complete regeneration:". Pathology. pathol.med.stu.edu.cn. Retrieved 2013-11-10.
After the repair process has been completed, the structure and function of the injured tissue are completely normal. This type of regeneration is common in physiological situations. Examples of physiological regeneration are the continual replacement of cells of the skin and repair of the endometrium after menstruation. Complete regeneration can occur in pathological situations in tissues that have good regenerative capacity.
- ↑ Mohammadi, Dara (4 October 2014). "Bioengineered organs: The story so far…". theguardian.com. Retrieved 9 March 2015.
- ↑ Carlson, B.M. (2007). Principles of Regenerative Biology. Elsevier Inc. pp. 25–26.
- ↑ Ferenczy, A (1979). "Proliferation kinetics of human endometrium during the normal menstrual cycle.". Am J Obstet Gynecol. 133: 859–67. PMID 434029. doi:10.1016/0002-9378(79)90302-8.
- 1 2 Michalopoulos, G.K. (1997). "Liver Regeneration". Science.
- 1 2 Taub, R. (2004). "Liver Regeneration: From Myth to Mechanism". Science.
- ↑ Kawasaki, S (1992). "Liver regeneration in recipients and donors after transplantation.". Lancet. 339: 580–1. PMID 1347095. doi:10.1016/0140-6736(92)90867-3.
- ↑ Spalding, K.L. (2013). "Dynamics of Hippocampal Neurogenesis in Adult Humans". Cell. 153: 1219–27. PMC 4394608
. PMID 23746839. doi:10.1016/j.cell.2013.05.002.
- ↑ Bergmann, O (2009). "Evidence for cardiomyocyte renewal in humans". Science. 324: 98–102. PMC 2991140
. PMID 19342590. doi:10.1126/science.1164680.
- ↑ Beltrami, A.P. (2001). "Evidence that human cardiac myocytes divide after myocardial infarction.". N Engl J Med. 344: 1750–7. PMID 11396441. doi:10.1056/NEJM200106073442303.
- ↑ McKin, L.H. (1932). "Regeneration of the Distal Phalanx". The Canadian Medical Association Journal. 26: 549–50. PMC 402335
. PMID 20318716.
- ↑ Muneoka, K (2008). "Mammalian regeneration and regenerative medicine". Birth Defects Research. 84: 265–80. PMID 19067422. doi:10.1002/bdrc.20137.
- ↑ Satheesh, P.J. (2005). "Morphological study of rib regeneration following costectomy in adolescent idiopathic scoliosis". Eur Spine J. 14: 772–6. PMC 3489251
. PMID 16047208. doi:10.1007/s00586-005-0949-8.
- 1 2 3 4 5 6 Alibardi, Lorenzo (2010). "Regeneration in Reptiles and Its Position Among Vertebrates". Morphological and Cellular Aspects of Tail and Limb Regeneration in Lizards a Model System with Implications for Tissue Regeneration in Mammals. Heidelberg:Springer.
- 1 2 3 McLean, Katherine E; Vickaryous, Matthew K (2011-08-16). "A novel amniote model of epimorphic regeneration: the leopard gecko, Eublepharis macularius". BMC Developmental Biology. 11 (1): 50. doi:10.1186/1471-213x-11-50.
- 1 2 Bellairs, A.; Bryant, S. (1985). "Autonomy and Regeneration in Reptiles". In Gans, Carl; Billet, F. Biology of the Reptilia. 15. New York: John Wiley and Sons. pp. 301–410.
- ↑ Brazaitis, Peter (July 31, 1981). "Maxillary Regeneration in a Marsh Crocodile, Crocodylus palustris". Journal of Herpetology. 15 (3): 360–362. doi:10.2307/1563441.
- ↑ Font, Enrique; Desfilis, Ester; M. Mar, Pérez-Cañellas; García-Verdugo, Jose Manuel (2001). "Neurogenesis and Neuronal Regeneration in the Adult Reptilian Brain". Brain, Behavior and Evolution. 58 (5): 276–295. doi:10.1159/000057570.
- ↑ "Vickaryous Lab".
- ↑ Reichman, O.J. (1984). "Evolution of Regeneration Capabilities". The American Naturalist. 123 (6): 752–763. doi:10.1086/284237.
- 1 2 3 Sun, Yifan (1992). "Rhodopsin regeneration in the normal and in the detached/replaced retina of the skate". Experimental Eye Research.
- 1 2 3 Lu, Conger (2013). "Study of MicroRNAs Related to the Liver Regeneration of the Whitespotted Bamboo Shark, Chiloscyllium plagiosum". BioMed Research International.
- 1 2 3 4 Vorontsova, M.A.; Liosner, L.D. (1960). Asexual Propagation and Regeneration. Pergamon Press. pp. 367–371.
- 1 2 3 4 Reif, Wolf-Ernst (1978). "Wound Healing in Sharks". Zoomorphology.
Sources
- Tanaka EM (October 2003). "Cell differentiation and cell fate during urodele tail and limb regeneration". Curr. Opin. Genet. Dev. 13 (5): 497–501. PMID 14550415. doi:10.1016/j.gde.2003.08.003.
- Nye HL, Cameron JA, Chernoff EA, Stocum DL (February 2003). "Regeneration of the urodele limb: a review". Dev. Dyn. 226 (2): 280–94. PMID 12557206. doi:10.1002/dvdy.10236.
- Yu H, Mohan S, Masinde GL, Baylink DJ (December 2005). "Mapping the dominant wound healing and soft tissue regeneration QTL in MRL x CAST". Mamm. Genome. 16 (12): 918–24. PMID 16341671. doi:10.1007/s00335-005-0077-0.
- Gardiner DM, Blumberg B, Komine Y, Bryant SV (June 1995). "Regulation of HoxA expression in developing and regenerating axolotl limbs". Development. 121 (6): 1731–41. PMID 7600989.
- Torok MA, Gardiner DM, Shubin NH, Bryant SV (August 1998). "Expression of HoxD genes in developing and regenerating axolotl limbs". Dev. Biol. 200 (2): 225–33. PMID 9705229. doi:10.1006/dbio.1998.8956.
- Putta S, Smith JJ, Walker JA, et al. (August 2004). "From biomedicine to natural history research: EST resources for ambystomatid salamanders". BMC Genomics. 5 (1): 54. PMC 509418
. PMID 15310388. doi:10.1186/1471-2164-5-54.
- Andrews, Wyatt (March 23, 2008). "Medicine's Cutting Edge: Re-Growing Organs". Sunday Morning. CBS News.
External links
![]() |
Wikiquote has quotations related to: Regeneration (biology) |
![]() |
Wikisource has the text of a 1920 Encyclopedia Americana article about Regeneration. |
- Spallanzani's mouse: a model of restoration and regeneration
- Mice that regrow hearts in the news
- DARPA Grant Supports Research Toward Realizing Tissue Regeneration
- The Geniuses Of Regeneration in BusinessWeek, May 24, 2004
- UCI Limb Regeneration Lab
- A site dedicated to amputation and regeneration
- Regeneration in African spiny mice