RNA world
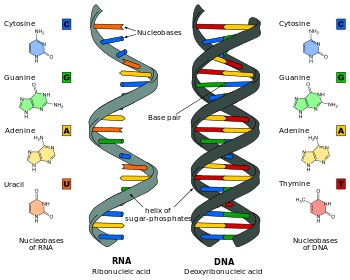
The RNA world is a hypothetical phase of the evolutionary history of life on Earth, in which self-replicating RNA molecules proliferated before the evolution of DNA and proteins.
The concept of the RNA world was first proposed in 1962 by Alexander Rich,[1] and the term was coined by Walter Gilbert in 1986.[2] Alternative chemical paths to life have been proposed,[3] and RNA-based life may not have been the first life to exist.[2][4] Even so, the evidence for an RNA world is strong enough that the hypothesis has gained wide acceptance.[1][5][6]
Like DNA, RNA can store and replicate genetic information; like enzymes, it can catalyze (start or accelerate) chemical reactions that are critical for life (then called Ribozyme).[7] One of the most critical components of the cell, ribosome, is composed primarily of RNA. Ribonucleotide moieties in many coenzymes, such as Acetyl-CoA, NADH, FADH and F420, have long been thought to be surviving remnants of covalently bound coenzymes in an RNA world.[8]
Although RNA is fragile, some ancient RNAs may have evolved the ability to methylate other RNAs to protect them.[9]
If the RNA world existed, it was probably followed by an age characterized by the evolution of ribonucleoproteins,[2] which in turn ushered in the era of DNA and longer proteins. The reason why DNA became the predominant storage molecule may be because it is more stable and durable than RNA.[10] Protein enzymes may have come to replace RNA-based ribozymes as biocatalysts because their greater abundance and diversity of monomers makes them more versatile. As some co-factors contain both nucleotide and amino acid characteristic, it may be that amino acids, peptides and finally proteins initially were co-factors for ribozymes.[8]
History
One of the challenges in studying abiogenesis is that the system of reproduction and metabolism utilized by all extant life involves three distinct types of interdependent macromolecules (DNA, RNA, and protein). This suggests that life could not have arisen in its current form, and mechanisms have then been sought whereby the current system might have arisen from a simpler precursor system. The concept of RNA as a primordial molecule[2] can be found in papers by Francis Crick[11] and Leslie Orgel,[12] as well as in Carl Woese's 1967 book The Genetic Code.[13] In 1962, the molecular biologist Alexander Rich, of the Massachusetts Institute of Technology, had posited much the same idea in an article he contributed to a volume issued in honor of Nobel-laureate physiologist Albert Szent-Györgyi.[14] Hans Kuhn in 1972 laid out a possible process by which the modern genetic system might have arisen from a nucleotide-based precursor, and this led Harold White in 1976 to observe that many of the cofactors essential for enzymatic function are either nucleotides or could have been derived from nucleotides. He proposed that these nucleotide cofactors represent "fossils of nucleic acid enzymes".[15] The phrase "RNA World" was first used by Nobel laureate Walter Gilbert in 1986, in a commentary on how recent observations of the catalytic properties of various forms of RNA fit with this hypothesis.[16]
Properties of RNA
The properties of RNA make the idea of the RNA world hypothesis conceptually plausible, though its general acceptance as an explanation for the origin of life requires further evidence.[14] RNA is known to form efficient catalysts and its similarity to DNA makes clear its ability to store information. Opinions differ, however, as to whether RNA constituted the first autonomous self-replicating system or was a derivative of a still-earlier system.[2] One version of the hypothesis is that a different type of nucleic acid, termed pre-RNA, was the first one to emerge as a self-reproducing molecule, to be replaced by RNA only later. On the other hand, the recent finding that activated pyrimidine ribonucleotides can be synthesized under plausible prebiotic conditions[17] means that it is premature to dismiss the RNA-first scenarios.[2] Suggestions for 'simple' pre-RNA nucleic acids have included peptide nucleic acid (PNA), threose nucleic acid (TNA) or glycol nucleic acid (GNA).[18][19] Despite their structural simplicity and possession of properties comparable with RNA, the chemically plausible generation of "simpler" nucleic acids under prebiotic conditions has yet to be demonstrated.[20]
RNA as an enzyme
RNA enzymes, or ribozymes, are found in today's DNA-based life and could be examples of living fossils. Ribozymes play vital roles, such as those in the ribosome, which is vital for protein synthesis. Many other ribozyme functions exist; for example, the hammerhead ribozyme performs self-cleavage[21] and an RNA polymerase ribozyme can synthesize a short RNA strand from a primed RNA template.[22]
Among the enzymatic properties important for the beginning of life are:
- Self-replication
- The ability to self-replicate, or synthesize other RNA molecules; relatively short RNA molecules that can synthesize others have been artificially produced in the lab. The shortest was 165-bases long, though it has been estimated that only part of the molecule was crucial for this function. One version, 189-bases long, had an error rate of just 1.1% per nucleotide when synthesizing an 11 nucleotide long RNA strand from primed template strands.[23] This 189 base pair ribozyme could polymerize a template of at most 14 nucleotides in length, which is too short for self replication, but a potential lead for further investigation. The longest primer extension performed by a ribozyme polymerase was 20 bases.[24] In 2016, researchers reported the use of in vitro evolution to improve dramatically the activity and generality of an RNA polymerase ribozyme by selecting variants that can synthesize functional RNA molecules from an RNA template. Each RNA polymerase ribozyme was engineered to remain linked to its new, synthesized RNA strand, this allowed the team to isolate successful polymerases. The isolated RNA polymerases were again used for another round of evolution. After several rounds of evolution, they obtained one RNA polymerase ribozyme called 24-3 that was able to copy almost any other RNA, from small catalysts to long RNA based enzymes. Particular RNAs were amplified up to 10,000 times, a first RNA version of the polymerase chain reaction (PCR). The RNA polymerase is not yet able to make copies of itself.[25]
- Catalysis
- The ability to catalyze simple chemical reactions—which would enhance creation of molecules that are building blocks of RNA molecules (i.e., a strand of RNA that would make creating more strands of RNA easier). Relatively short RNA molecules with such abilities have been artificially formed in the lab.[26][27] A recent study showed that almost any nucleic acid can evolve into a catalytic sequence under appropriate selection. For instance, an arbitrarily chosen 50-nucleotide DNA fragment encoding for the Bos taurus (cattle) albumin mRNA was subjected to test-tube evolution to derive a catalytic DNA (Deoxyribozyme, also called DNAzyme) with RNA-cleavage activity. After only a few weeks, a DNAzyme with significant catalytic activity had evolved.[28] In general, DNA is much more chemically inert than RNA and hence much more resistant to obtaining catalytic properties. If in vitro evolution works for DNA it will happen much more easily with RNA.
- Amino acid-RNA ligation
- The ability to conjugate an amino acid to the 3'-end of an RNA in order to use its chemical groups or provide a long-branched aliphatic side-chain.[29]
- Peptide bond formation
- The ability to catalyse the formation of peptide bonds between amino acids to produce short peptides or longer proteins. This is done in modern cells by ribosomes, a complex of several RNA molecules known as rRNA together with many proteins. The rRNA molecules are thought responsible for its enzymatic activity, as no amino acid molecules lie within 18Å of the enzyme's active site,[14] and, when the majority of the amino acids in the ribosome were stringently removed, the resulting ribosome retained its full peptidyl transferase activity, fully able to catalyze the formation of peptide bonds between amino acids.[30] A much shorter RNA molecule has been synthesized in the laboratory with the ability to form peptide bonds, and it has been suggested that rRNA has evolved from a similar molecule.[31] It has also been suggested that amino acids may have initially been involved with RNA molecules as cofactors enhancing or diversifying their enzymatic capabilities, before evolving to more complex peptides. Similarly, tRNA is suggested to have evolved from RNA molecules that began to catalyze amino acid transfer.[32]
RNA in information storage
RNA is a very similar molecule to DNA, and only has two chemical differences. The overall structure of RNA and DNA are immensely similar—one strand of DNA and one of RNA can bind to form a double helical structure. This makes the storage of information in RNA possible in a very similar way to the storage of information in DNA. However, RNA is less stable.
Comparison of DNA and RNA structure
The major difference between RNA and DNA is the presence of a hydroxyl group at the 2'-position of the ribose sugar in RNA (illustration, right).[14] This group makes the molecule less stable because, when not constrained in a double helix, the 2' hydroxyl can chemically attack the adjacent phosphodiester bond to cleave the phosphodiester backbone. The hydroxyl group also forces the ribose into the C3'-endo sugar conformation unlike the C2'-endo conformation of the deoxyribose sugar in DNA. This forces an RNA double helix to change from a B-DNA structure to one more closely resembling A-DNA.
RNA also uses a different set of bases than DNA—adenine, guanine, cytosine and uracil, instead of adenine, guanine, cytosine and thymine. Chemically, uracil is similar to thymine, differing only by a methyl group, and its production requires less energy.[33] In terms of base pairing, this has no effect. Adenine readily binds uracil or thymine. Uracil is, however, one product of damage to cytosine that makes RNA particularly susceptible to mutations that can replace a GC base pair with a GU (wobble) or AU base pair.
RNA is thought to have preceded DNA, because of their ordering in the biosynthetic pathways. The deoxyribonucleotides used to make DNA are made from ribonucleotides, the building blocks of RNA, by removing the 2'-hydroxyl group. As a consequence a cell must have the ability to make RNA before it can make DNA.
Limitations of information storage in RNA
The chemical properties of RNA make large RNA molecules inherently fragile, and they can easily be broken down into their constituent nucleotides through hydrolysis.[34][35] These limitations do not make use of RNA as an information storage system impossible, simply energy intensive (to repair or replace damaged RNA molecules) and prone to mutation. While this makes it unsuitable for current 'DNA optimised' life, it may have been acceptable for more primitive life.
RNA as a regulator
Riboswitches have been found to act as regulators of gene expression, particularly in bacteria, but also in plants and archaea. Riboswitches alter their secondary structure in response to the binding of a metabolite. This change in structure can result in the formation or disruption of a terminator, truncating or permitting transcription respectively.[36] Alternatively, riboswitches may bind or occlude the Shine-Dalgarno sequence, affecting translation.[37] It has been suggested that these originated in an RNA-based world.[38] In addition, RNA thermometers regulate gene expression in response to temperature changes.[39]
Support and difficulties
The RNA world hypothesis is supported by RNA's ability to store, transmit, and duplicate genetic information, as DNA does. RNA can act as a ribozyme, a special type of enzyme. Because it can perform the tasks of both DNA and enzymes, RNA is believed to have once been capable of supporting independent life forms.[14] Some viruses use RNA as their genetic material, rather than DNA.[40] Further, while nucleotides were not found in experiments based on Miller-Urey experiment, their formation in prebiotically plausible conditions has now been reported, as noted above;[17] the purine base known as adenine is merely a pentamer of hydrogen cyanide. Experiments with basic ribozymes, like Bacteriophage Qβ RNA, have shown that simple self-replicating RNA structures can withstand even strong selective pressures (e.g., opposite-chirality chain terminators).[41]
Since there were no known chemical pathways for the abiogenic synthesis of nucleotides from pyrimidine nucleobases cytosine and uracil under prebiotic conditions, it is thought by some that nucleic acids did not contain these nucleobases seen in life's nucleic acids.[42] The nucleoside cytosine has a half-life in isolation of 19 days at 100 °C (212 °F) and 17,000 years in freezing water, which some argue is too short on the geologic time scale for accumulation.[43] Others have questioned whether ribose and other backbone sugars could be stable enough to find in the original genetic material,[44] and have raised the issue that all ribose molecules would have had to be the same enantiomer, as any nucleotide of the wrong chirality acts as a chain terminator.[45]
Pyrimidine ribonucleosides and their respective nucleotides have been prebiotically synthesised by a sequence of reactions that by-pass free sugars and assemble in a stepwise fashion by including nitrogenous and oxygenous chemistries. In a series of publications, John Sutherland and his team at the School of Chemistry, University of Manchester, have demonstrated high yielding routes to cytidine and uridine ribonucleotides built from small 2 and 3 carbon fragments such as glycolaldehyde, glyceraldehyde or glyceraldehyde-3-phosphate, cyanamide and cyanoacetylene. One of the steps in this sequence allows the isolation of enantiopure ribose aminooxazoline if the enantiomeric excess of glyceraldehyde is 60% or greater, of possible interest towards biological homochirality.[46] This can be viewed as a prebiotic purification step, where the said compound spontaneously crystallised out from a mixture of the other pentose aminooxazolines. Aminooxazolines can react with cyanoacetylene in a mild and highly efficient manner, controlled by inorganic phosphate, to give the cytidine ribonucleotides. Photoanomerization with UV light allows for inversion about the 1' anomeric centre to give the correct beta stereochemistry; one problem with this chemistry is the selective phosphorylation of alpha-cytidine at the 2' position.[47] However, in 2009, they showed that the same simple building blocks allow access, via phosphate controlled nucleobase elaboration, to 2',3'-cyclic pyrimidine nucleotides directly, which are known to be able to polymerise into RNA.[17] Organic chemist Donna Blackmond described this finding as "strong evidence" in favour of the RNA world.[48] However, John Sutherland said that while his team's work suggests that nucleic acids played an early and central role in the origin of life, it did not necessarily support the RNA world hypothesis in the strict sense, which he described as a "restrictive, hypothetical arrangement".[49]
The Sutherland group's 2009 paper also highlighted the possibility for the photo-sanitization of the pyrimidine-2',3'-cyclic phosphates.[17] A potential weakness of these routes is the generation of enantioenriched glyceraldehyde, or its 3-phosphate derivative (glyceraldehyde prefers to exist as its keto tautomer dihydroxyacetone).
On August 8, 2011, a report, based on NASA studies with meteorites found on Earth, was published suggesting building blocks of RNA (adenine, guanine and related organic molecules) may have been formed extraterrestrially in outer space.[50][51][52] On August 29, 2012, astronomers at Copenhagen University reported the detection of a specific sugar molecule, glycolaldehyde, in a distant star system. The molecule was found around the protostellar binary IRAS 16293-2422, which is located 400 light years from Earth.[53][54] Because glycolaldehyde is needed to form RNA, this finding suggests that complex organic molecules may form in stellar systems prior to the formation of planets, eventually arriving on young planets early in their formation.[55]
"Molecular biologist's dream"
"Molecular biologist's dream" is a phrase coined by Gerald Joyce and Leslie Orgel to refer to the problem of emergence of self-replicating RNA molecules, as any movement towards an RNA world on a properly modeled prebiotic early Earth would have been continuously suppressed by destructive reactions.[56] It was noted that many of the steps needed for the nucleotides formation do not proceed efficiently in prebiotic conditions.[57] Joyce and Orgel specifically referred the molecular biologist's dream to "a magic catalyst" that could "convert the activated nucleotides to a random ensemble of polynucleotide sequences, a subset of which had the ability to replicate".[56]
Joyce and Orgel further argued that nucleotides cannot link unless there is some activation of the phosphate group, whereas the only effective activating groups for this are "totally implausible in any prebiotic scenario", particularly adenosine triphosphate.[56] According to Joyce and Orgel, in case of the phosphate group activation, the basic polymer product would have 5',5'-pyrophosphate linkages, while the 3',5'-phosphodiester linkages, which are present in all known RNA, would be much less abundant.[56] The associated molecules would have been also prone to addition of incorrect nucleotides or to reactions with numerous other substances likely to have been present.[56] The RNA molecules would have been also continuously degraded by such destructive process as spontaneous hydrolysis, present on the early Earth.[56] Joyce and Orgel proposed to reject "the myth of a self-replicating RNA molecule that arose de novo from a soup of random polynucleotides"[56] and hypothesised a scenario where the prebiotic processes furnish pools of enantiopure beta-D-ribonucleosides.[58] In a later paper, Joyce describes "The myth of a small RNA molecule that arises de novo and can replicate efficiently and with high fidelity under plausible prebiotic conditions" as a strawman.[59]
Prebiotic RNA synthesis
Nucleotides are the fundamental molecules that combine in series to form RNA. They consist of a nitrogenous base attached to a sugar-phosphate backbone. RNA is made of long stretches of specific nucleotides arranged so that their sequence of bases carries information. The RNA world hypothesis holds that in the primordial soup (or sandwich), there existed free-floating nucleotides. These nucleotides regularly formed bonds with one another, which often broke because the change in energy was so low. However, certain sequences of base pairs have catalytic properties that lower the energy of their chain being created, enabling them to stay together for longer periods of time. As each chain grew longer, it attracted more matching nucleotides faster, causing chains to now form faster than they were breaking down.
These chains have been proposed by some as the first, primitive forms of life.[60] In an RNA world, different sets of RNA strands would have had different replication outputs, which would have increased or decreased their frequency in the population, i.e. natural selection. As the fittest sets of RNA molecules expanded their numbers, novel catalytic properties added by mutation, which benefitted their persistence and expansion, could accumulate in the population. Such an autocatalytic set of ribozymes, capable of self replication in about an hour, has been identified. It was produced by molecular competition (in vitro evolution) of candidate enzyme mixtures.[61]
Competition between RNA may have favored the emergence of cooperation between different RNA chains, opening the way for the formation of the first protocell. Eventually, RNA chains developed with catalytic properties that help amino acids bind together (a process called peptide-bonding). These amino acids could then assist with RNA synthesis, giving those RNA chains that could serve as ribozymes the selective advantage. The ability to catalyze one step in protein synthesis, aminoacylation of RNA, has been demonstrated in a short (five-nucleotide) segment of RNA.[62]
One of the problems with the RNA world hypothesis is to discover the pathway by which RNA became upgraded to the DNA system. Geoffrey Diemer and Ken Stedman, at Portland State University in Oregon, may have found a solution. While conducting a survey of viruses in a hot acidic lake in Lassen Volcanic National Park, California, they uncovered evidence that a simple DNA virus had acquired a gene from a completely unrelated RNA-based virus. Virologist Luis Villareal of the University of California Irvine also suggests that viruses capable of converting an RNA-based gene into DNA and then incorporating it into a more complex DNA-based genome might have been common in the Virus world during the RNA to DNA transition some 4 billion years ago.[63][64] This finding bolsters the argument for the transfer of information from the RNA world to the emerging DNA world before the emergence of the Last Universal Common Ancestor. From the research, the diversity of this virus world is still with us.
In March 2015, NASA scientists reported that, for the first time, complex DNA and RNA organic compounds of life, including uracil, cytosine and thymine, have been formed in the laboratory under conditions found only in outer space, using starting chemicals, like pyrimidine, found in meteorites. Pyrimidine, like polycyclic aromatic hydrocarbons (PAHs), the most carbon-rich chemical found in the Universe, may have been formed in giant red stars or in interstellar dust and gas clouds, according to the scientists.[65]
Viroids
Additional evidence supporting the concept of an RNA world has resulted from research on viroids, the first representatives of a novel domain of "subviral pathogens".[66][67] Viroids are mostly plant pathogens, which consist of short stretches (a few hundred nucleobases) of highly complementary, circular, single-stranded, and non-coding RNA without a protein coat. Compared with other infectious plant pathogens, viroids are extremely small in size, ranging from 246 to 467 nucleobases. In comparison, the genome of the smallest known viruses capable of causing an infection are about 2,000 nucleobases long.[68]
In 1989, Diener proposed that, based on their characteristic properties, viroids are more plausible "living relics" of the RNA world than are introns or other RNAs then so considered.[69] If so, viroids have attained potential significance beyond plant pathology to evolutionary biology, by representing the most plausible macromolecules known capable of explaining crucial intermediate steps in the evolution of life from inanimate matter (see: abiogenesis).
Apparently, Diener's hypothesis lay dormant until 2014, when Flores et al. published a review paper, in which Diener's evidence supporting his hypothesis was summarized.[70] In the same year, a New York Times science writer published a popularized version of Diener's proposal, in which, however, he mistakenly credited Flores et al. with the hypothesis' original conception.[71]
Pertinent viroid properties listed in 1989 are:
- their small size, imposed by error-prone replication;
- their high guanine and cytosine content, which increases stability and replication fidelity;
- their circular structure, which assures complete replication without genomic tags;
- existence of structural periodicity, which permits modular assembly into enlarged genomes;
- their lack of protein-coding ability, consistent with a ribosome-free habitat; and
- replication mediated in some by ribozymes—the fingerprint of the RNA world.[70]
The existence, in extant cells, of RNAs with molecular properties predicted for RNAs of the RNA World constitutes an additional argument supporting the RNA World hypothesis.
Origin of sex
Eigen et al.[72] and Woese[73] proposed that the genomes of early protocells were composed of single-stranded RNA, and that individual genes corresponded to separate RNA segments, rather than being linked end-to-end as in present-day DNA genomes. A protocell that was haploid (one copy of each RNA gene) would be vulnerable to damage, since a single lesion in any RNA segment would be potentially lethal to the protocell (e.g. by blocking replication or inhibiting the function of an essential gene).
Vulnerability to damage could be reduced by maintaining two or more copies of each RNA segment in each protocell, i.e. by maintaining diploidy or polyploidy. Genome redundancy would allow a damaged RNA segment to be replaced by an additional replication of its homolog. However, for such a simple organism, the proportion of available resources tied up in the genetic material would be a large fraction of the total resource budget. Under limited resource conditions, the protocell reproductive rate would likely be inversely related to ploidy number. The protocell's fitness would be reduced by the costs of redundancy. Consequently, coping with damaged RNA genes while minimizing the costs of redundancy would likely have been a fundamental problem for early protocells.
A cost-benefit analysis was carried out in which the costs of maintaining redundancy were balanced against the costs of genome damage.[74] This analysis led to the conclusion that, under a wide range of circumstances, the selected strategy would be for each protocell to be haploid, but to periodically fuse with another haploid protocell to form a transient diploid. The retention of the haploid state maximizes the growth rate. The periodic fusions permit mutual reactivation of otherwise lethally damaged protocells. If at least one damage-free copy of each RNA gene is present in the transient diploid, viable progeny can be formed. For two, rather than one, viable daughter cells to be produced would require an extra replication of the intact RNA gene homologous to any RNA gene that had been damaged prior to the division of the fused protocell. The cycle of haploid reproduction, with occasional fusion to a transient diploid state, followed by splitting to the haploid state, can be considered to be the sexual cycle in its most primitive form.[74][75] In the absence of this sexual cycle, haploid protocells with damage in an essential RNA gene would simply die.
This model for the early sexual cycle is hypothetical, but it is very similar to the known sexual behavior of the segmented RNA viruses, which are among the simplest organisms known. Influenza virus, whose genome consists of 8 physically separated single-stranded RNA segments,[76] is an example of this type of virus. In segmented RNA viruses, “mating” can occur when a host cell is infected by at least two virus particles. If these viruses each contain an RNA segment with a lethal damage, multiple infection can lead to reactivation providing that at least one undamaged copy of each virus gene is present in the infected cell. This phenomenon is known as “multiplicity reactivation”. Multiplicity reactivation has been reported to occur in influenza virus infections after induction of RNA damage by UV-irradiation,[77] and ionizing radiation.[78]
Further developments
Patrick Forterre has been working on a novel hypothesis, called "three viruses, three domains":[79] that viruses were instrumental in the transition from RNA to DNA and the evolution of Bacteria, Archaea, and Eukaryota. He believes the last common ancestor (specifically, the "last universal cellular ancestor")[79] was RNA-based and evolved RNA viruses. Some of the viruses evolved into DNA viruses to protect their genes from attack. Through the process of viral infection into hosts the three domains of life evolved.[79][80] Another interesting proposal is the idea that RNA synthesis might have been driven by temperature gradients, in the process of thermosynthesis.[81] Single nucleotides have been shown to catalyze organic reactions.[82]
Steven Benner has argued that chemical conditions on the planet Mars, such as the presence of boron, molybdenum and oxygen, may have been better for initially producing RNA molecules than those on Earth. If so, life-suitable molecules, originating on Mars, may have later migrated to Earth via panspermia or similar process.[83][84]
Alternative hypotheses
The hypothesized existence of an RNA world does not exclude a "Pre-RNA world", where a metabolic system based on a different nucleic acid is proposed to pre-date RNA. A candidate nucleic acid is peptide nucleic acid (PNA), which uses simple peptide bonds to link nucleobases.[85] PNA is more stable than RNA, but its ability to be generated under prebiological conditions has yet to be demonstrated experimentally.
Threose nucleic acid (TNA) has also been proposed as a starting point, as has glycol nucleic acid (GNA), and like PNA, also lack experimental evidence for their respective abiogenesis.
An alternative — or complementary — theory of RNA origin is proposed in the PAH world hypothesis, whereby polycyclic aromatic hydrocarbons (PAHs) mediate the synthesis of RNA molecules.[86] PAHs are the most common and abundant of the known polyatomic molecules in the visible Universe, and are a likely constituent of the primordial sea.[87] PAHs, along with fullerenes (also implicated in the origin of life),[88] have been recently detected in nebulae.[89]
The iron-sulfur world theory proposes that simple metabolic processes developed before genetic materials did, and these energy-producing cycles catalyzed the production of genes.
Some of the difficulties of producing the precursors on earth are bypassed by another alternative or complementary theory for their origin, panspermia. It discusses the possibility that the earliest life on this planet was carried here from somewhere else in the galaxy, possibly on meteorites similar to the Murchison meteorite.[90] This does not invalidate the concept of an RNA world, but posits that this world or its precursors originated not on Earth but rather another, probably older, planet.
There are hypotheses that are in direct conflict to the RNA world hypothesis. The relative chemical complexity of the nucleotide and the unlikelihood of it spontaneously arising, along with the limited number of combinations possible among four base forms, as well as the need for RNA polymers of some length before seeing enzymatic activity, have led some to reject the RNA world hypothesis in favor of a metabolism-first hypothesis, where the chemistry underlying cellular function arose first, along with the ability to replicate and facilitate this metabolism.
RNA-peptide coevolution
Another proposal is that the dual-molecule system we see today, where a nucleotide-based molecule is needed to synthesize protein, and a protein-based molecule is needed to make nucleic acid polymers, represents the original form of life.[91] This theory is called RNA-peptide coevolution,[92] or the Peptide-RNA world, and offers a possible explanation for the rapid evolution of high-quality replication in RNA (since proteins are catalysts), with the disadvantage of having to postulate the coincident formation of two complex molecules, an enzyme (from peptides) and a RNA (from nucleotides). In this Peptide-RNA World scenario, RNA would have contained the instructions for life, while peptides (simple protein enzymes) would have accelerated key chemical reactions to carry out those instructions.[93] The study leaves open the question of exactly how those primitive systems managed to replicate themselves — something neither the RNA World hypothesis nor the Peptide-RNA World theory can yet explain, unless polymerases (enzymes that rapidly assemble the RNA molecule) played a role.[93]
A research project completed in March 2015 by the Sutherland group found that a network of reactions beginning with hydrogen cyanide and hydrogen sulfide, in streams of water irradiated by UV light, could produce the chemical components of proteins and lipids, alongside those of RNA.[94][95] The researchers used the term "cyanosulfidic" to describe this network of reactions.[94]
Implications of the RNA world
The RNA world hypothesis, if true, has important implications for the definition of life. For most of the time that followed Watson and Crick's elucidation of DNA structure in 1953, life was largely defined in terms of DNA and proteins: DNA and proteins seemed the dominant macromolecules in the living cell, with RNA only aiding in creating proteins from the DNA blueprint.
The RNA world hypothesis places RNA at center-stage when life originated. The RNA world hypothesis is supported by the observations that ribosomes are ribozymes: the catalytic site is composed of RNA, and proteins hold no major structural role and are of peripheral functional importance. This was confirmed with the deciphering of the 3-dimensional structure of the ribosome in 2001. Specifically, peptide bond formation, the reaction that binds amino acids together into proteins, is now known to be catalyzed by an adenine residue in the rRNA.
RNAs are known to play roles in other cellular catalytic processes, specifically in the targeting of enzymes to specific RNA sequences. In eukaryotes, the processing of pre-mRNA and RNA editing take place at sites determined by the base pairing between the target RNA and RNA constituents of small nuclear ribonucleoproteins (snRNPs). Such enzyme targeting is also responsible for gene down regulation though RNA interference (RNAi), where an enzyme-associated guide RNA targets specific mRNA for selective destruction. Likewise, in eukaryotes the maintenance of telomeres involves copying of an RNA template that is a constituent part of the telomerase ribonucleoprotein enzyme.[96] Another cellular organelle, the vault, includes a ribonucleoprotein component, although the function of this organelle remains to be elucidated.
See also
References
- 1 2 Neveu M, Kim HJ, Benner SA (Apr 2013). "The "strong" RNA world hypothesis: fifty years old". Astrobiology. 13 (4): 391–403. Bibcode:2013AsBio..13..391N. PMID 23551238. doi:10.1089/ast.2012.0868.
[The RNA world's existence] has broad support within the community today.
- 1 2 3 4 5 6 Cech TR (Jul 2012). "The RNA worlds in context". Cold Spring Harbor Perspectives in Biology. 4 (7): a006742. PMC 3385955
. PMID 21441585. doi:10.1101/cshperspect.a006742.
- ↑ Patel BH, Percivalle C, Ritson DJ, Duffy CD, Sutherland JD (Apr 2015). "Common origins of RNA, protein and lipid precursors in a cyanosulfidic protometabolism". Nature Chemistry. 7 (4): 301–7. Bibcode:2015NatCh...7..301P. PMC 4568310
. PMID 25803468. doi:10.1038/nchem.2202.
- ↑ Robertson MP, Joyce GF (May 2012). "The origins of the RNA world". Cold Spring Harbor Perspectives in Biology. 4 (5): a003608. PMC 3331698
. PMID 20739415. doi:10.1101/cshperspect.a003608.
- ↑ Wade, Nicholas (May 4, 2015). "Making Sense of the Chemistry That Led to Life on Earth". New York Times. Retrieved May 10, 2015.
- ↑ Copley SD, Smith E, Morowitz HJ (Dec 2007). "The origin of the RNA world: co-evolution of genes and metabolism". Bioorganic Chemistry. 35 (6): 430–43. PMID 17897696. doi:10.1016/j.bioorg.2007.08.001.
The proposal that life on Earth arose from an RNA World is widely accepted.
- ↑ Zimmer, Carl (September 25, 2014). "A Tiny Emissary from the Ancient Past". New York Times. Retrieved September 26, 2014.
- 1 2 Shen, Liang.; Hong-Fang, Ji (18 July 2011). "Small Cofactors May Assist Protein Emergence from RNA World: Clues from RNA-Protein Complexes". Biochimie. 6. PMC 3138788
. PMID 27375676. doi:10.1371/journal.pone.0022494. free online
- ↑ Rana, Ajay K.; Ankri, Serge (6 June 2016). "Reviving the RNA World: An Insight into the Appearance of RNA Methyltransferases". Frontiers in Genetics. 7. PMC 4893491
. PMID 21789260. doi:10.3389/fgene.2016.00099.
- ↑ Garwood RJ (2012). "Patterns In Palaeontology: The first 3 billion years of evolution". Palaeontology Online. 2 (11): 1–14. Retrieved June 25, 2015.
- ↑ Crick FH (Dec 1968). "The origin of the genetic code". Journal of Molecular Biology. 38 (3): 367–79. PMID 4887876. doi:10.1016/0022-2836(68)90392-6.
- ↑ Orgel LE (Dec 1968). "Evolution of the genetic apparatus". Journal of Molecular Biology. 38 (3): 381–93. PMID 5718557. doi:10.1016/0022-2836(68)90393-8.
- ↑ Woese C.R. (1967). The genetic code: The molecular basis for genetic expression. p. 186. Harper & Row
- 1 2 3 4 5 Atkins JF, Gesteland RF, Cech T (2006). The RNA world: the nature of modern RNA suggests a prebiotic RNA world. Plainview, N.Y: Cold Spring Harbor Laboratory Press. ISBN 0-87969-739-3.
- ↑ White HB (Mar 1976). "Coenzymes as fossils of an earlier metabolic state". Journal of Molecular Evolution. 7 (2): 101–4. PMID 1263263. doi:10.1007/BF01732468.
- ↑ Gilbert, Walter (February 1986). "The RNA World". Nature. 319 (6055): 618. Bibcode:1986Natur.319..618G. doi:10.1038/319618a0.
- 1 2 3 4 Powner MW, Gerland B, Sutherland JD (May 2009). "Synthesis of activated pyrimidine ribonucleotides in prebiotically plausible conditions". Nature. 459 (7244): 239–42. Bibcode:2009Natur.459..239P. PMID 19444213. doi:10.1038/nature08013.
- ↑ Orgel L (Nov 2000). "Origin of life. A simpler nucleic acid". Science. 290 (5495): 1306–7. PMID 11185405. doi:10.1126/science.290.5495.1306.
- ↑ Nelson KE, Levy M, Miller SL (Apr 2000). "Peptide nucleic acids rather than RNA may have been the first genetic molecule". Proceedings of the National Academy of Sciences of the United States of America. 97 (8): 3868–71. Bibcode:2000PNAS...97.3868N. PMC 18108
. PMID 10760258. doi:10.1073/pnas.97.8.3868.
- ↑ Anastasi C, Buchet FF, Crowe MA, Parkes AL, Powner MW, Smith JM, Sutherland JD (Apr 2007). "RNA: prebiotic product, or biotic invention?". Chemistry & Biodiversity. 4 (4): 721–39. PMID 17443885. doi:10.1002/cbdv.200790060.
- ↑ Forster AC, Symons RH (Apr 1987). "Self-cleavage of plus and minus RNAs of a virusoid and a structural model for the active sites". Cell. 49 (2): 211–20. PMID 2436805. doi:10.1016/0092-8674(87)90562-9.
- ↑ Johnston WK, Unrau PJ, Lawrence MS, Glasner ME, Bartel DP (May 2001). "RNA-catalyzed RNA polymerization: accurate and general RNA-templated primer extension" (PDF). Science. 292 (5520): 1319–25. Bibcode:2001Sci...292.1319J. PMID 11358999. doi:10.1126/science.1060786.
- ↑ Johnston WK, Unrau PJ, Lawrence MS, Glasner ME, Bartel DP (May 2001). "RNA-catalyzed RNA polymerization: accurate and general RNA-templated primer extension". Science. 292 (5520): 1319–25. Bibcode:2001Sci...292.1319J. PMID 11358999. doi:10.1126/science.1060786.
- ↑ Hani S. Zaher and Peter J. Unrau, Selection of an improved RNA polymerase ribozyme with superior extension and fidelity. RNA (2007), 13:1017-1026
- ↑ Horning, David P.; Joyce, Gerald F. (2016-08-15). "Amplification of RNA by an RNA polymerase ribozyme". Proceedings of the National Academy of Sciences. 113 (35): 9786–91. ISSN 0027-8424. PMC 5024611
. PMID 27528667. doi:10.1073/pnas.1610103113.
- ↑ Huang F, Yang Z, Yarus M (1998). "RNA enzymes with two small-molecule substrates". Chem. Biol. 5 (11): 669–78. PMID 9831528. doi:10.1016/s1074-5521(98)90294-0.
- ↑ Unrau PJ, Bartel DP (Sep 1998). "RNA-catalysed nucleotide synthesis". Nature. 395 (6699): 260–3. Bibcode:1998Natur.395..260U. PMID 9751052. doi:10.1038/26193.
- ↑ Gysbers R, Tram K, Gu J, Li Y (2015). "Evolution of an Enzyme from a Noncatalytic Nucleic Acid Sequence". Scientific Reports. 5: 11405. PMC 4473686
. PMID 26091540. doi:10.1038/srep11405.
- ↑ Erives A (Aug 2011). "A model of proto-anti-codon RNA enzymes requiring L-amino acid homochirality". Journal of Molecular Evolution. 73 (1–2): 10–22. PMC 3223571
. PMID 21779963. doi:10.1007/s00239-011-9453-4.
- ↑ Noller HF, Hoffarth V, Zimniak L (Jun 1992). "Unusual resistance of peptidyl transferase to protein extraction procedures". Science. 256 (5062): 1416–9. Bibcode:1992Sci...256.1416N. PMID 1604315. doi:10.1126/science.1604315.
- ↑ Zhang B, Cech TR (Nov 1997). "Peptide bond formation by in vitro selected ribozymes". Nature. 390 (6655): 96–100. Bibcode:1997Natur.390...96Z. PMID 9363898. doi:10.1038/36375.
- ↑ Szathmáry E (Jun 1999). "The origin of the genetic code: amino acids as cofactors in an RNA world". Trends in Genetics. 15 (6): 223–9. PMID 10354582. doi:10.1016/S0168-9525(99)01730-8.
- ↑ http://www.humpath.com/uracil
- ↑ Lindahl T (Apr 1993). "Instability and decay of the primary structure of DNA". Nature. 362 (6422): 709–15. Bibcode:1993Natur.362..709L. PMID 8469282. doi:10.1038/362709a0.
- ↑ Pääbo, S (November 1993). "Ancient DNA". Scientific American. 269 (5): 60–66. doi:10.1038/scientificamerican1193-86.
- ↑ Nudler E, Mironov AS (Jan 2004). "The riboswitch control of bacterial metabolism". Trends in Biochemical Sciences. 29 (1): 11–7. PMID 14729327. doi:10.1016/j.tibs.2003.11.004.
- ↑ Tucker BJ, Breaker RR (Jun 2005). "Riboswitches as versatile gene control elements". Current Opinion in Structural Biology. 15 (3): 342–8. PMID 15919195. doi:10.1016/j.sbi.2005.05.003.
- ↑ Bocobza SE, Aharoni A (2008). "Switching the light on plant riboswitches". Trends Plant Sci. 13 (10): 526–33. PMID 18778966. doi:10.1016/j.tplants.2008.07.004.
- ↑ Narberhaus F, Waldminghaus T, Chowdhury S (Jan 2006). "RNA thermometers". FEMS Microbiology Reviews. 30 (1): 3–16. PMID 16438677. doi:10.1111/j.1574-6976.2005.004.x.
- ↑ Patton, John T. Editor (2008). Segmented Double-stranded RNA Viruses: Structure and Molecular Biology. Caister Academic Press. Editor's affiliation: Laboratory of Infectious Diseases, NIAID, NIH, Bethesda, MD 20892-8026. ISBN 978-1-904455-21-9
- ↑ Bell, Graham: The Basics of Selection. Springer, 1997.
- ↑ Orgel LE (Oct 1994). "The origin of life on the earth". Scientific American. 271 (4): 76–83. PMID 7524147. doi:10.1038/scientificamerican1094-76.
- ↑ Levy M, Miller SL (Jul 1998). "The stability of the RNA bases: implications for the origin of life". Proceedings of the National Academy of Sciences of the United States of America. 95 (14): 7933–8. Bibcode:1998PNAS...95.7933L. PMC 20907
. PMID 9653118. doi:10.1073/pnas.95.14.7933.
- ↑ Larralde R, Robertson MP, Miller SL (Aug 1995). "Rates of decomposition of ribose and other sugars: implications for chemical evolution". Proceedings of the National Academy of Sciences of the United States of America. 92 (18): 8158–60. Bibcode:1995PNAS...92.8158L. PMC 41115
. PMID 7667262. doi:10.1073/pnas.92.18.8158.
- ↑ Joyce GF, Visser GM, van Boeckel CA, van Boom JH, Orgel LE, van Westrenen J (1984). "Chiral selection in poly(C)-directed synthesis of oligo(G)". Nature. 310 (5978): 602–4. Bibcode:1984Natur.310..602J. PMID 6462250. doi:10.1038/310602a0.
- ↑ Carole Anastasi, Michael A. Crowe, Matthew W. Powner, John D. Sutherland "Direct Assembly of Nucleoside Precursors from Two- and Three-Carbon Units Angewandte Chemie International Edition 45(37):6176–79, 2006.
- ↑ Powner MW, Sutherland JD (2008). "Potentially prebiotic synthesis of pyrimidine beta-D-ribonucleotides by photoanomerization/hydrolysis of alpha-D-cytidine-2'-phosphate". Chembiochem. 9 (15): 2386–7. PMID 18798212. doi:10.1002/cbic.200800391.
- ↑ Van Noorden R (2009). "RNA world easier to make". Nature. doi:10.1038/news.2009.471.
- ↑ Urquhart J (13 May 2009), "Insight into RNA origins", Chemistry World, Royal Society of Chemistry
- ↑ Callahan MP, Smith KE, Cleaves HJ, Ruzicka J, Stern JC, Glavin DP, House CH, Dworkin JP (Aug 2011). "Carbonaceous meteorites contain a wide range of extraterrestrial nucleobases". Proceedings of the National Academy of Sciences of the United States of America. PNAS. 108 (34): 13995–8. Bibcode:2011PNAS..10813995C. PMC 3161613
. PMID 21836052. doi:10.1073/pnas.1106493108.
- ↑ Steigerwald J (8 August 2011). "NASA Researchers: DNA Building Blocks Can Be Made in Space". NASA. Retrieved 2011-08-10.
- ↑ ScienceDaily Staff (9 August 2011). "DNA Building Blocks Can Be Made in Space, NASA Evidence Suggests". ScienceDaily. Retrieved 2011-08-09.
- ↑ Than, Ker (August 29, 2012). "Sugar Found In Space". National Geographic. Retrieved August 31, 2012.
- ↑ Staff (August 29, 2012). "Sweet! Astronomers spot sugar molecule near star". AP News. Retrieved August 31, 2012.
- ↑ Jørgensen JK, Favre C, Bisschop S, Bourke T, Dishoeck E, Schmalzl M (2012). "Detection of the simplest sugar, glycolaldehyde, in a solar-type protostar with ALMA" (PDF). The Astrophysical Journal Letters. eprint. 757: L4. Bibcode:2012ApJ...757L...4J. doi:10.1088/2041-8205/757/1/L4.
- 1 2 3 4 5 6 7 Mills GC, Kenyon D. "The RNA World: A Critique". Access Research Network. Retrieved 10 Sep 2011.
- ↑ Schopf JW (2002). Life's origin: the beginnings of biological evolution. University of California Press. p. 150. ISBN 0-520-23390-5.
- ↑ "Prebiotic RNA chemistry: realising the molecular biologist's dream". Engineering and Physical Sciences Research Council. Retrieved 10 Sep 2011.
- ↑ http://www.ncbi.nlm.nih.gov/pmc/articles/PMC3331698/
- ↑ Villarreal LP, Witzany G (2013). "The DNA Habitat and its RNA Inhabitants: At the Dawn of RNA". Sociology. 6: 1–12. doi:10.4137/GEI.S11490.
- ↑ Lincoln TA, Joyce GF (Feb 2009). "Self-sustained replication of an RNA enzyme". Science. American Association for the Advancement of Science. 323 (5918): 1229–32. Bibcode:2009Sci...323.1229L. PMC 2652413
. PMID 19131595. doi:10.1126/science.1167856. Lay summary – Medical News Today (January 12, 2009).
- ↑ Turk RM, Chumachenko NV, Yarus M (Mar 2010). "Multiple translational products from a five-nucleotide ribozyme". Proceedings of the National Academy of Sciences of the United States of America. 107 (10): 4585–9. Bibcode:2010PNAS..107.4585T. PMC 2826339
. PMID 20176971. doi:10.1073/pnas.0912895107. Lay summary – ScienceDaily (February 24, 2010).
- ↑ Holmes, Bob (2012) "First Glimpse at the birth of DNA" (New Scientist April 12, 2012)
- ↑ Diemer GS, Stedman KM (19 April 2012). "A novel virus genome discovered in an extreme environment suggests recombination between unrelated groups of RNA and DNA viruses". Biology Direct. BioMed Central. 7 (1): 13. PMC 3372434
. PMID 22515485. doi:10.1186/1745-6150-7-13. Retrieved 7 February 2015.
- ↑ Marlaire R (3 March 2015). "NASA Ames Reproduces the Building Blocks of Life in Laboratory". NASA. Retrieved 5 March 2015.
- ↑ Diener TO (Aug 1971). "Potato spindle tuber "virus". IV. A replicating, low molecular weight RNA". Virology. 45 (2): 411–28. PMID 5095900. doi:10.1016/0042-6822(71)90342-4.
- ↑ "ARS Research Timeline – Tracking the Elusive Viroid". 2006-03-02. Retrieved 2007-07-18.
- ↑ Sanger HL, Klotz G, Riesner D, Gross HJ, Kleinschmidt AK (Nov 1976). "Viroids are single-stranded covalently closed circular RNA molecules existing as highly base-paired rod-like structures". Proceedings of the National Academy of Sciences of the United States of America. 73 (11): 3852–6. PMC 431239
. PMID 1069269. doi:10.1073/pnas.73.11.3852.
- ↑ Diener TO (1989). "Circular RNAs: Relics of precellular evolution?". Proc. Natl. Acad. Sci. USA. 86 (23): 9370–9374. PMC 298497
. PMID 2480600. doi:10.1073/pnas.86.23.9370.
- 1 2 Flores R, Gago-Zachert S, Serra P, Sanjuan R, Elena SF (2014). "Viroids: Survivors from the RNA World?". Annu. Rev. Microbiol. 68: 395–41. PMID 25002087. doi:10.1146/annurev-micro-091313-103416.
- ↑ Zimmer, Carl (September 25, 2014). "A Tiny Emissary From the Ancient Past". New York Times. Retrieved November 22, 2014.
- ↑ Eigen M, Gardiner W, Schuster P, Winkler-Oswatitsch R (Apr 1981). "The origin of genetic information". Scientific American. 244 (4): 88–92, 96, et passim. Bibcode:1981SciAm.244...88H. PMID 6164094. doi:10.1038/scientificamerican0481-88.
- ↑ Woese CR (1983). The primary lines of descent and the universal ancestor. Chapter in Bendall DS (1983). Evolution from molecules to men. Cambridge, UK: Cambridge University Press. ISBN 0-521-28933-5. pp. 209-233.
- 1 2 Bernstein H, Byerly HC, Hopf FA, Michod RE (Oct 1984). "Origin of sex". Journal of Theoretical Biology. 110 (3): 323–51. PMID 6209512. doi:10.1016/S0022-5193(84)80178-2.
- ↑ Bernstein C, Bernstein H (1991). Aging, sex, and DNA repair. Boston: Academic Press. ISBN 0-12-092860-4. see pgs. 293-297
- ↑ Lamb RA, Choppin PW (1983). "The gene structure and replication of influenza virus". Annual Review of Biochemistry. 52: 467–506. PMID 6351727. doi:10.1146/annurev.bi.52.070183.002343.
- ↑ Barry RD (Aug 1961). "The multiplication of influenza virus. II. Multiplicity reactivation of ultraviolet irradiated virus". Virology. 14 (4): 398–405. PMID 13687359. doi:10.1016/0042-6822(61)90330-0.
- ↑ Gilker JC, Pavilanis V, Ghys R (Jun 1967). "Multiplicity reactivation in gamma irradiated influenza viruses". Nature. 214 (5094): 1235–7. Bibcode:1967Natur.214.1235G. PMID 6066111. doi:10.1038/2141235a0.
- 1 2 3 Forterre P (March 2006). "Three RNA cells for ribosomal lineages and three DNA viruses to replicate their genomes: a hypothesis for the origin of cellular domain". Proc. Natl. Acad. Sci. U.S.A. 103: 3669–74. PMC 1450140
. PMID 16505372. doi:10.1073/pnas.0510333103.
- ↑ Zimmer C (May 2006). "Did DNA come from viruses?". Science. 312 (5775): 870–2. PMID 16690855. doi:10.1126/science.312.5775.870.
- ↑ Muller AW (Oct 2005). "Thermosynthesis as energy source for the RNA World: a model for the bioenergetics of the origin of life". Bio Systems. 82 (1): 93–102. PMID 16024164. doi:10.1016/j.biosystems.2005.06.003.
- ↑ Kumar A, Sharma S, Maurya RA (2010). "Single Nucleotide-Catalyzed Biomimetic Reductive Amination". Advanced Synthesis and Catalyst. 352 (13): 2227–2232. doi:10.1002/adsc.201000178.
- ↑ Zimmer, Carl (September 12, 2013). "A Far-Flung Possibility for the Origin of Life". New York Times. Retrieved September 12, 2013.
- ↑ Webb R (August 29, 2013). "Primordial broth of life was a dry Martian cup-a-soup". New Scientist. Retrieved September 13, 2013.
- ↑ Egholm M, Buchardt O, Christensen L, Behrens C, Freier SM, Driver DA, Berg RH, Kim SK, Norden B, Nielsen PE (Oct 1993). "PNA hybridizes to complementary oligonucleotides obeying the Watson-Crick hydrogen-bonding rules". Nature. 365 (6446): 566–8. Bibcode:1993Natur.365..566E. PMID 7692304. doi:10.1038/365566a0.
- ↑ Platts, Simon Nicholas, "The PAH World – Discotic polynuclear aromatic compounds as a mesophase scaffolding at the origin of life"
- ↑ Allamandola, Louis et Al. "Cosmic Distribution of Chemical Complexity"
- ↑ Atkinson, Nancy (2010-10-27). "Buckyballs Could Be Plentiful in the Universe". Universe Today. Retrieved 2010-10-28.
- ↑ García-Hernández DA, Manchado A, García-Lario P, Stanghellini L, Villaver E, Shaw RA, Szczerba R, Perea-Calderón JV (2010-10-28). "Formation Of Fullerenes In H-Containing Planetary Nebulae". The Astrophysical Journal Letters. 724: L39. Bibcode:2010ApJ...724L..39G. arXiv:1009.4357
. doi:10.1088/2041-8205/724/1/L39.
- ↑ Bernstein MP, Sandford SA, Allamandola LJ, Gillette JS, Clemett SJ, Zare RN (Feb 1999). "UV irradiation of polycyclic aromatic hydrocarbons in ices: production of alcohols, quinones, and ethers". Science. 283 (5405): 1135–8. Bibcode:1999Sci...283.1135B. PMID 10024233. doi:10.1126/science.283.5405.1135.
- ↑ Kunin V (Oct 2000). "A system of two polymerases--a model for the origin of life". Origins of Life and Evolution of the Biosphere. 30 (5): 459–66. Bibcode:2000OLEB...30..459K. PMID 11002892. doi:10.1023/A:1006672126867.
- ↑ Pascal, Robert (2007), "A scenario starting from the first chemical building blocks", in Reisse, Jacques, From Suns to Life: A Chronological Approach to the History of Life on Earth, Springer Science & Business Media, pp. 163–166, ISBN 0-387-45083-1
- 1 2 "Challenging Assumptions About the Origin of Life". Astrobiology Magazine. 18 September 2013. Retrieved 2014-05-07.
- 1 2 Patel BH, Percivalle C, Ritson DJ, Duffy CD, Sutherland JD (Apr 2015). "Common origins of RNA, protein and lipid precursors in a cyanosulfidic protometabolism". Nature Chemistry. Nature Publishing Group. 7 (4): 301–7. Bibcode:2015NatCh...7..301P. PMC 4568310
. PMID 25803468. doi:10.1038/nchem.2202.
- ↑ Service, Robert F. (16 March 2015). "Researchers may have solved origin-of-life conundrum". Science (News). Washington, D.C.: American Association for the Advancement of Science. ISSN 1095-9203. Retrieved 2015-07-26.
- ↑ Witzany G (2008). "The Viral Origins of Telomeres and Telomerases and their Important Role in Eukaryogenesis and Genome Maintenance". Biosemiotics. 1 (2): 191–206. doi:10.1007/s12304-008-9018-0.
Further reading
- Cairns-Smith AG (1993). Genetic Takeover: And the Mineral Origins of Life. Cambridge University Press. ISBN 0-521-23312-7.
- Orgel LE (Oct 1994). "The origin of life on the earth". Scientific American. 271 (4): 76–83. PMID 7524147. doi:10.1038/scientificamerican1094-76.
- Orgel LE (2004). "Prebiotic chemistry and the origin of the RNA world". Critical Reviews in Biochemistry and Molecular Biology. 39 (2): 99–123. PMID 15217990. doi:10.1080/10409230490460765.
- Woolfson A (September 2000). Life Without Genes. London: Flamingo. ISBN 978-0-00-654874-4.
- Vlassov AV, Kazakov SA, Johnston BH, Landweber LF (Aug 2005). "The RNA world on ice: a new scenario for the emergence of RNA information". Journal of Molecular Evolution. 61 (2): 264–73. PMID 16044244. doi:10.1007/s00239-004-0362-7.
- Engelhart AE, Hud NV (Dec 2010). "Primitive genetic polymers" (PDF). Cold Spring Harbor Perspectives in Biology. 2 (12): a002196. PMC 2982173
. PMID 20462999. doi:10.1101/cshperspect.a002196.
- Bernhardt HS (2012). "The RNA world hypothesis: the worst theory of the early evolution of life (except for all the others)(a)". Biology Direct. 7 (1): 23. PMC 3495036
. PMID 22793875. doi:10.1186/1745-6150-7-23.
- Sutherland JD (Apr 2010). "Ribonucleotides". Cold Spring Harbor Perspectives in Biology. 2 (4): a005439. PMC 2845210
. PMID 20452951. doi:10.1101/cshperspect.a005439.
External links
- "Understanding the RNA World". Exploring Life's Origins. Exploring Origins Project.
- Ferris, James P. "The Formation of the RNA World". The New York Center for Studies of the Origins of Life, Rensselaer Polytechnic Institute. Archived from the original on 2012-03-01.
- Altman, Sidney (2001). "The RNA World". NobelPrize.org. Nobel Media.
- Kuska, Robert (June 2002). "A World Apart" (PDF). HHMI Bulletin. Howard Hughes Medical Institute. pp. 14–19. Archived (PDF) from the original on 2004-05-22.
- Cech, Thomas R. (2004). "Exploring the New RNA World". NobelPrize.org. Nobel Media.
- Sutherland, J. D. (April 2010). "Ribonucleotides". Cold Spring Harbor Perspectives in Biology. 2 (4): a005439. PMC 2845210
. PMID 20452951. doi:10.1101/cshperspect.a005439.