Pyrolysis
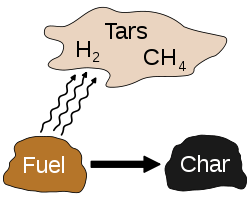
Pyrolysis is a thermochemical decomposition of organic material at elevated temperatures in the absence of oxygen (or any halogen). It involves the simultaneous change of chemical composition and physical phase, and is irreversible. The word is coined from the Greek-derived elements pyro "fire" and lysis "separating".
Pyrolysis is a type of thermolysis, and is most commonly observed in organic materials exposed to high temperatures. It is one of the processes involved in charring wood, starting at 200–300 °C (390–570 °F).[1] It also occurs in fires where solid fuels are burning or when vegetation comes into contact with lava in volcanic eruptions. In general, pyrolysis of organic substances produces gas and liquid products and leaves a solid residue richer in carbon content, char. Extreme pyrolysis, which leaves mostly carbon as the residue, is called carbonization.
The process is used heavily in the chemical industry, for example, to produce charcoal, activated carbon, methanol, and other chemicals from wood, to convert ethylene dichloride into vinyl chloride to make PVC, to produce coke from coal, to convert biomass into syngas and biochar, to turn waste plastics back into usable oil,[2] or waste into safely disposable substances, and for transforming medium-weight hydrocarbons from oil into lighter ones like gasoline. These specialized uses of pyrolysis may be called various names, such as dry distillation, destructive distillation, or cracking. Pyrolysis is also used in the creation of nanoparticles,[3] zirconia[4] and oxides[5] utilizing an ultrasonic nozzle in a process called ultrasonic spray pyrolysis (USP).
Pyrolysis also plays an important role in several cooking procedures, such as baking, frying, grilling, and caramelizing. It is a tool of chemical analysis, for example, in mass spectrometry and in carbon-14 dating. Indeed, many important chemical substances, such as phosphorus and sulfuric acid, were first obtained by this process. Pyrolysis has been assumed to take place during catagenesis, the conversion of buried organic matter to fossil fuels. It is also the basis of pyrography. In their embalming process, the ancient Egyptians used a mixture of substances, including methanol, which they obtained from the pyrolysis of wood.
Pyrolysis differs from other processes like combustion and hydrolysis in that it usually does not involve reactions with oxygen, water, or any other reagents.[6] In practice, it is not possible to achieve a completely oxygen-free atmosphere. Because some oxygen is present in any pyrolysis system, a small amount of oxidation occurs.
The term has also been applied to the decomposition of organic material in the presence of superheated water or steam (hydrous pyrolysis), for example, in the steam cracking of oil.
Occurrence and uses
Fire
Pyrolysis is usually the first chemical reaction that occurs in the burning of many solid organic fuels, like wood, cloth, and paper, and also of some kinds of plastic. In a wood fire, the visible flames are not due to combustion of the wood itself, but rather of the gases released by its pyrolysis, whereas the flame-less burning of a solid, called smouldering, is the combustion of the solid residue (char or charcoal) left behind by pyrolysis. Thus, the pyrolysis of common materials like wood, plastic, and clothing is extremely important for fire safety and firefighting. In pyrolysis there is a gas phase present. It should not be confused with hydrothermal reactions such as hydrothermal gasification, hydrothermal liquidation, and hydrothermal carbonization, which occur in aqueous environments because the temperatures and reaction pathways differ, with ionic reactions favored in aqueous reactions and radical reactions favored in the absence of water.
Cooking
Pyrolysis occurs whenever food is exposed to high enough temperatures in a dry environment, such as roasting, baking, toasting, or grilling. It is the chemical process responsible for the formation of the golden-brown crust in foods prepared by those methods.
In normal cooking, the main food components that undergo pyrolysis are carbohydrates (including sugars, starch, and fibre) and proteins. (See: Maillard reaction.) Pyrolysis of fats requires a much higher temperature, and, since it produces toxic and flammable products (such as acrolein), it is, in general, avoided in normal cooking. It may occur, however, when grilling fatty meats over hot coals.
Even though cooking is normally carried out in air, the temperatures and environmental conditions are such that there is little or no combustion of the original substances or their decomposition products. In particular, the pyrolysis of proteins and carbohydrates begins at temperatures much lower than the ignition temperature of the solid residue, and the volatile subproducts are too diluted in air to ignite. (In flambé dishes, the flame is due mostly to combustion of the alcohol, while the crust is formed by pyrolysis as in baking.)
Pyrolysis of carbohydrates and proteins requires temperatures substantially higher than 100 °C (212 °F), so pyrolysis does not occur as long as free water is present, e.g., in boiling food — not even in a pressure cooker. When heated in the presence of water, carbohydrates and proteins suffer gradual hydrolysis rather than pyrolysis. Indeed, for most foods, pyrolysis is usually confined to the outer layers of food, and begins only after those layers have dried out.
Food pyrolysis temperatures are, however, lower than the boiling point of lipids, so pyrolysis occurs when frying in vegetable oil or suet, or basting meat in its own fat.
Pyrolysis also plays an essential role in the production of barley tea, coffee, and roasted nuts such as peanuts and almonds. As these consist mostly of dry materials, the process of pyrolysis is not limited to the outermost layers but extends throughout the materials. In all these cases, pyrolysis creates or releases many of the substances that contribute to the flavor, color, and biological properties of the final product. It may also destroy some substances that are toxic, unpleasant in taste, or those that may contribute to spoilage.
Controlled pyrolysis of sugars starting at 170 °C (338 °F) produces caramel, a beige to brown water-soluble product widely used in confectionery and (in the form of caramel coloring) as a coloring agent for soft drinks and other industrialized food products.
Solid residue from the pyrolysis of spilled and splattered food creates the brown-black encrustation often seen on cooking vessels, stove tops, and the interior surfaces of ovens.
Charcoal
People have used pyrolysis for turning wood into charcoal on an industrial scale since ancient times. Besides wood, the process can also use sawdust and other wood-waste products.
Charcoal is obtained by heating wood until its complete pyrolysis (carbonization) occurs, leaving only carbon and inorganic ash. In many parts of the world charcoal is still produced semi-industrially by burning a pile of wood that has been mostly covered with mud or with bricks. The heat generated by burning part of the wood and the volatile byproducts pyrolyzes the rest of the pile. The limited supply of oxygen prevents the charcoal from burning. A more modern alternative is to heat the wood in an airtight metal vessel, which is much less polluting and allows the volatile products to be condensed.
The original vascular structure of the wood and the pores created by escaping gases combine to produce a light and porous material. By starting with a dense wood-like material, such as nutshells or peach stones, one obtains a form of charcoal with particularly fine pores (and hence a much larger pore surface area), called activated carbon, which is used as an adsorbent for a wide range of chemical substances.
Biochar
Residues of incomplete organic pyrolysis, e.g., from cooking fires, are thought to be the key component of the terra preta soils associated with ancient indigenous communities of the Amazon basin.[7] Terra preta is much sought by local farmers for its superior fertility compared to the natural red soil of the region. Efforts are underway to recreate these soils through biochar, the solid residue of pyrolysis of various materials, mostly organic waste.
Biochar improves the soil texture and ecology, increasing its ability to retain fertilizers and release them slowly. It naturally contains many of the micronutrients needed by plants, such as selenium. It is also safer than other "natural" fertilizers such as animal manure, since it has been disinfected at high temperature. And, since it releases its nutrients at a slow rate, it greatly reduces the risk of water table contamination.[8]
Biochar is also being considered for carbon sequestration, with the aim of mitigation of global warming.[9][10][11] The solid, carbon-containing char produced can be sequestered in the ground, where it could remain for several hundred to a few thousand years.[12] Research continues about the processes responsible for long-term sequestration of carbon in soils.[13]
Coke
Pyrolysis is used on a massive scale to turn coal into coke for metallurgy, especially steelmaking. Coke can also be produced from the solid residue left from petroleum refining.
Those starting materials typically contain hydrogen, nitrogen, or oxygen atoms combined with carbon into molecules of medium to high molecular weight. The coke-making or "coking" process consists of heating the material in closed vessels to very high temperatures (up to 2,000 °C or 3,600 °F) so that those molecules are broken down into lighter volatile substances, which leave the vessel, and a porous but hard residue that is mostly carbon and inorganic ash. The amount of volatiles varies with the source material, but is typically 25–30% of it by weight.
Carbon fiber
Carbon fibers are filaments of carbon that can be used to make very strong yarns and textiles. Carbon fiber items are often produced by spinning and weaving the desired item from fibers of a suitable polymer, and then pyrolyzing the material at a high temperature (from 1,500–3,000 °C or 2,730–5,430 °F).
The first carbon fibers were made from rayon, but polyacrylonitrile has become the most common starting material.
For their first workable electric lamps, Joseph Wilson Swan and Thomas Edison used carbon filaments made by pyrolysis of cotton yarns and bamboo splinters, respectively.
Pyrolytic carbon
Pyrolysis is the reaction used to coat a preformed substrate with a layer of pyrolytic carbon. This is typically done in a fluidized bed reactor heated to 1,000–2,000 °C or 1,830–3,630 °F. Pyrolytic carbon coatings are used in many applications, including artificial heart valves.[14]
Biofuel
Pyrolysis is the basis of several methods that are being developed for producing fuel from biomass, which may include either crops grown for the purpose or biological waste products from other industries.[15] Crops studied as biomass feedstock for pyrolysis include native North American prairie grasses such as switchgrass and bred versions of other grasses such as Miscantheus giganteus. Crops and plant material wastes provide biomass feedstock on the basis of their lignocellulose portions.
Although synthetic diesel fuel cannot yet be produced directly by pyrolysis of organic materials, there is a way to produce similar liquid (bio-oil) that can be used as a fuel, after the removal of valuable bio-chemicals that can be used as food additives or pharmaceuticals.[16] Higher efficiency is achieved by flash pyrolysis, in which finely divided feedstock is quickly heated to between 350 and 500 °C (660 and 930 °F) for less than 2 seconds.
Fuel bio-oil can also be produced by hydrous pyrolysis from many kinds of feedstock, including waste from pig and turkey farming, by a process called thermal depolymerization (which may, however, include other reactions besides pyrolysis).
Adhesives
Neanderthals used pyrolysis of birch bark to produce a pitch with which they secured flaked stones to spear shafts.[17] Recently, researchers have developed a process to pyrolyze birch bark to produce an oil that can replace phenol in phenol formaldehyde resin (these resins are mostly used to manufacture plywood).[18]
Pesticides
Pyrolysis can also be used to produce pesticides from biomass.[19]
Plastic waste disposal
Anhydrous pyrolysis can also be used to produce liquid fuel similar to diesel from plastic waste, with a higher cetane value and lower sulphur content than conventional diesel.[20] Using pyrolysis to extract fuel from end-of-life plastic is a second-best option after recycling, is environmentally preferable to landfill, and can help reduce dependency on fossil fuels and geo-extraction.[21]
Waste tire disposal
In the United States alone, over 290 million car tires are discarded annually. Pyrolysis of scrap or waste tires (WT) is an attractive alternative to disposal in landfills, allowing the high energy content of the tire to be recovered as fuel. Using tires as fuel produces equal energy as burning oil and 25% more energy than burning coal.[22]
An average car tire is made up of 50–60% hydrocarbons, resulting in a yield of 38–56% oil, 10–30% gas and 14–56% char. The oil produced is largely composed of benzene, diesel, kerosene, fuel oil and heavy fuel oil, while the produced gas has a similar composition to natural gas. The proportion and the purity of the products are governed by two major factors:[23]
- Environment (e.g. pressure, temperature, time, reactor type)
- Material (e.g. age, composition, size, type)
As car tires age, they increase in hardness, making it more difficult for pyrolysis to break the molecules into shorter chains. This shifts the yield composition towards diesel oil which is composed of larger molecules. Conversely, an increase in temperature increases the likelihood of breaking the molecule chain and shifts the yield composition towards benzene oil which is composed of smaller molecules.[23] Other products from car tire pyrolysis include steel wires, carbon black and bitumen.[24]
Although the pyrolysis of WT has been widely developed throughout the world, there are legislative, economic, and marketing obstacles to widespread adoption.[25] Oil derived from tire rubber pyrolysis contains high sulfur content, which gives it high potential as a pollutant and should be desulfurized.[26] A number of prototype and full-scale pyrolysis plants specialized in carbon black production have successfully established across the world, including the United States, France, Germany and Japan. Because carbon black is used for pigment, rubber strengthening and UV protection, it is a relatively large and growing market. Pyrolysis plants specialized in fuel oil production is not an implausible concept. However, as profits of such ventures come from the added value between the production and distillation of oil, there is little profit without vertical integration in the oil industry. The inconsistency of the feedstock makes it very difficult to control the uniformity of the products and makes oil companies hesitant to purchase oil produced via pyrolysis. Finally, the cost of producing oil through conventional means is generally less expensive than this alternative. To date, there is no known commercially profitable standalone pyrolysis plant that specializes in oil production.[23] However, with funding to upgrade pyrolysis oil to light fuel grade, this may be possible. Nevertheless, pyrolysis is a valuable method for disposing of waste tires.[27]
Chemical analysis
Pyrolysis can be used for the molecular characterisation of molecules when used in conjunction with gas chromatography-mass spectrometry (Py-GC-MS). This technique has been used to analyse the method and products of fungal decay of wood.[28][29]
Thermal cleaning
Pyrolysis is also used for thermal cleaning, an industrial application to remove organic substances such as polymers, plastics and coatings from parts, products or production components like extruder screws, spinnerets[30] and static mixers. During the thermal cleaning process, at temperatures between 600 °F to 1000 °F (310 C° to 540 C°),[31] organic material is converted by pyrolysis and oxidation into volatile organic compounds, hydrocarbons and carbonized gas.[32] Inorganic elements remain.[33]
Several types of thermal cleaning systems use pyrolysis:
- Molten Salt Baths belong to the oldest thermal cleaning systems; cleaning with a molten salt bath is very fast but implies the risk of dangerous splatters, or other potential hazards connected with the use of salt baths, like explosions or highly toxic hydrogen cyanide gas.[31]
- Fluidized Bed Systems[34] use sand or aluminium oxide as heating medium;[35] these systems also clean very fast but the medium does not melt or boil, nor emit any vapors or odors;[31] the cleaning process takes one to two hours.[32]
- Vacuum Ovens use pyrolysis in a vacuum[36] avoiding uncontrolled combustion inside the cleaning chamber;[31] the cleaning process takes 8[32] to 30 hours.[37]
- Burn-Off Ovens, also known as Heat-Cleaning Ovens, are gas-fired and used in the painting, coatings, electric motors and plastics industries for removing organics from heavy and large metal parts.[38]
Processes
In many industrial applications, the process is done under pressure and at operating temperatures above 430 °C (806 °F). For agricultural waste, for example, typical temperatures are 450 to 550 °C (840 to 1,000 °F).
Processes
Since pyrolysis is endothermic,[39] various methods to provide heat to the reacting biomass particles have been proposed:
- Partial combustion of the biomass products through air injection. This results in poor-quality products.
- Direct heat transfer with a hot gas, the ideal one being product gas that is reheated and recycled. The problem is to provide enough heat with reasonable gas flow-rates.
- Indirect heat transfer with exchange surfaces (wall, tubes): it is difficult to achieve good heat transfer on both sides of the heat exchange surface.
- Direct heat transfer with circulating solids: solids transfer heat between a burner and a pyrolysis reactor. This is an effective but complex technology.
For flash pyrolysis, the biomass must be ground into fine particles and the insulating char layer that forms at the surface of the reacting particles must be continuously removed. The following technologies have been proposed for biomass pyrolysis:[40]
- Fixed beds used for the traditional production of charcoal: poor, slow heat transfer result in very low liquid yields.
- Augers: this technology is adapted from a Lurgi process for coal gasification. Hot sand and biomass particles are fed at one end of a screw. The screw mixes the sand and biomass and conveys them along. It provides a good control of the biomass residence time. It does not dilute the pyrolysis products with a carrier or fluidizing gas. However, sand must be reheated in a separate vessel, and mechanical reliability is a concern. There is no large-scale commercial implementation.
- Electrically heated augers: one process uses an electrical current passed through an auger to heat the material giving excellent heat transfer by contact and radiation to the waste material.
- Ablative processes: biomass particles are moved at high speed against a hot metal surface. Ablation of any char forming at a particle's surface maintains a high rate of heat transfer. This can be achieved by using a metal surface spinning at high speed within a bed of biomass particles, which may present mechanical reliability problems but prevents any dilution of the products. As an alternative, the particles may be suspended in a carrier gas and introduced at high speed through a cyclone whose wall is heated; the products are diluted with the carrier gas.[41] A problem shared with all ablative processes is that scale-up is made difficult, since the ratio of the wall surface to the reactor volume decreases as the reactor size is increased.[42] There is no large-scale commercial implementation.
- Rotating cone: pre-heated hot sand and biomass particles are introduced into a rotating cone. Due to the rotation of the cone, the mixture of sand and biomass is transported across the cone surface by centrifugal force. The process is offered by BTG-BTL, a subsidiary from BTG Biomass Technology Group B.V. in The Netherlands. Like other shallow transported-bed reactors relatively fine particles (several mm) are required to obtain a liquid yield of around 70 wt.%. Larger-scale commercial implementation (up to 5 t/h input) is underway.[43]
- Fluidized beds: biomass particles are introduced into a bed of hot sand fluidized by a gas, which is usually a recirculated product gas. High heat transfer rates from fluidized sand result in rapid heating of biomass particles. There is some ablation by attrition with the sand particles, but it is not as effective as in the ablative processes. Heat is usually provided by heat exchanger tubes through which hot combustion gas flows. There is some dilution of the products, which makes it more difficult to condense and then remove the bio-oil mist from the gas exiting the condensers. This process has been scaled up by companies such as Dynamotive and Agri-Therm. The main challenges are in improving the quality and consistency of the bio-oil.
- Circulating fluidized beds: biomass particles are introduced into a circulating fluidized bed of hot sand. Gas, sand, and biomass particles move together, with the transport gas usually being a recirculated product gas, although it may also be a combustion gas. High heat transfer rates from sand ensure rapid heating of biomass particles and ablation stronger than with regular fluidized beds. A fast separator separates the product gases and vapors from the sand and char particles. The sand particles are reheated in a fluidized burner vessel and recycled to the reactor. Although this process can be easily scaled up, it is rather complex and the products are much diluted, which greatly complicates the recovery of the liquid products.
- Mechanical Fluidized Reactor (MFR). A mechanical stirrer agitates a hot bed of pure char particles into which biomass particles are injected. The stirrer also enhances heat transfer from the reactor wall to the agitated bed. No fluidization gas is required: evolving vapors aerate the bed and greatly reduce the power consumption of the mechanical stirrer.[44] This compact reactor has been used for a mobile pyrolysis plant.[45]
- Chain grate: dry biomass is fed onto a hot (500 °C) heavy cast metal grate or apron which forms a continuous loop. A small amount of air aids in heat transfer and in primary reactions for drying and carbonization. Volatile products are combusted for process and boiler heating.
Use of vacuum
In vacuum pyrolysis, organic material is heated in a vacuum to decrease its boiling point and avoid adverse chemical reactions. Called flash vacuum pyrolysis, this approach is used in organic synthesis.
Industrial sources
Many sources of organic matter can be used as feedstock for pyrolysis. Suitable plant material includes greenwaste, sawdust, waste wood, woody weeds; and agricultural sources including nut shells, straw, cotton trash, rice hulls, switch grass; and animal waste including poultry litter, dairy manure, and potentially other manures. Pyrolysis is used as a form of thermal treatment to reduce waste volumes of domestic refuse. Some industrial byproducts are also suitable feedstock including paper sludge and distillers grain.[46]
There is also the possibility of integrating with other processes such as mechanical biological treatment and anaerobic digestion.[47]
Industrial products
- syngas (flammable mixture of carbon monoxide and hydrogen): can be produced in sufficient quantities to provide both the energy needed for pyrolysis and some excess production[9][48]
- solid char that can either be burned for energy or be recycled as a fertilizer (biochar).
Fire protection
Destructive fires in buildings will often burn with limited oxygen supply, resulting in pyrolysis reactions. Thus, pyrolysis reaction mechanisms and the pyrolysis properties of materials are important in fire protection engineering for passive fire protection. Pyrolytic carbon is also important to fire investigators as a tool for discovering origin and cause of fires.
Chemistry
Current research examines the multiple reaction pathways of pyrolysis to understand how to manipulate the formation of pyrolysis' multiple products (oil, gas, char, and miscellaneous chemicals) to enhance the economic value of pyrolysis; identifying catalysts to manipulate pyrolysis reactions is also a goal of some pyrolysis research. Published research suggests that pyrolysis reactions have some dependence upon the structural composition of feedstocks (e.g. lignocellulosic biomass), with contributions from some minerals present in the feedstocks; some minerals present in feedstock are thought to increase the cost of operation of pyrolysis or decrease the value of oil produced from pyrolysis, through corrosive reactions.[49] The low quality of oils produced through pyrolysis can be improved by subjecting the oils to one or many physical and chemical processes,[50] which might drive production costs, but may make sense economically as circumstances change.
See also
References
- ↑ Burning of wood, InnoFireWood's website. Accessed on 2010-02-06.
- ↑ The Plastic to Oil Machine | A\J – Canada's Environmental Voice. Alternativesjournal.ca (2016-12-07). Retrieved on 2016-12-16.
- ↑ Pingali, Kalyana C.; Rockstraw, David A.; Deng, Shuguang (2005). "Silver Nanoparticles from Ultrasonic Spray Pyrolysis of Aqueous Silver Nitrate" (PDF). Aerosol Science and Technology. 39 (10): 1010–1014. doi:10.1080/02786820500380255.
- ↑ Song, Y. L.; Tsai, S. C.; Chen, C. Y.; Tseng, T. K.; Tsai, C. S.; Chen, J. W.; Yao, Y. D. (2004). "Ultrasonic Spray Pyrolysis for Synthesis of Spherical Zirconia Particles" (PDF). Journal of the American Ceramic Society. 87 (10): 1864–1871. doi:10.1111/j.1151-2916.2004.tb06332.x.
- ↑ Hamedani, Hoda Amani (2008) Investigation of Deposition Parameters in Ultrasonic Spray Pyrolysis for Fabrication of Solid Oxide Fuel Cell Cathode, Georgia Institute of Technology
- ↑ Cory A. Kramer, Reza Loloee, Indrek S. Wichman and Ruby N. Ghosh, 2009, Time Resolved Measurements of Pyrolysis Products From Thermoplastic Poly-Methyl-Methacrylate (PMMA) ASME 2009 International Mechanical Engineering Congress and Exposition
- ↑ Lehmann, Johannes. "Biochar: the new frontier". Archived from the original on 2008-06-18. Retrieved 2008-07-10.
- ↑ Cundall, Peter (2007-10-27). "Fact Sheet: Pete's Patch". Gardening Australia. Australian Broadcasting Corporation. Retrieved 2008-07-10.
- 1 2 Horstman, Mark (2007-09-23). "Agrichar – A solution to global warming?". ABC TV Science: Catalyst. Australian Broadcasting Corporation. Retrieved 2008-07-10.
- ↑ "Trial to reverse global warming". BBC News. 2009-04-09. Retrieved 2009-04-21.
- ↑ "The virtues of biochar: A new growth industry?". The Economist. 2009-08-27. Retrieved 2009-08-30.
- ↑ Frequently Asked Questions about Biochar | International Biochar Initiative. Biochar-international.org (2013-04-19). Retrieved on 2013-06-01.
- ↑ Schmidt, Michael W. I.; Torn, Margaret S.; Abiven, Samuel; Dittmar, Thorsten; Guggenberger, Georg; Janssens, Ivan A.; Kleber, Markus; Kögel-Knabner, Ingrid; Lehmann, Johannes (2011-10-06). "Persistence of soil organic matter as an ecosystem property". Nature. 478 (7367): 49–56. ISSN 0028-0836. doi:10.1038/nature10386.
- ↑ Ratner, Buddy D. (2004). Pyrolytic carbon. In Biomaterials science: an introduction to materials in medicine. Academic Press. pp. 171-180. ISBN 0-12-582463-7.
- ↑ Evans, G. "Liquid Transport Biofuels – Technology Status Report" Archived September 19, 2008, at the Wayback Machine., "National Non-Food Crops Centre", 14-04-08. Retrieved on 2009-05-05.
- ↑ "Pyrolysis and Other Thermal Processing". US DOE. Archived from the original on 2007-08-14.
- ↑ NOVA – Official Website | Neanderthals Defy Stereotypes. Pbs.org. Retrieved on 2016-12-16.
- ↑ Dongbing Li, Franco Berruti, Cedric Briens, "Autothermal fast pyrolysis of birch bark with partial oxidation in a fluidized bed reactor", Fuel, Volume 121, 1 April 2014, Pages 27–38
- ↑ Smoking them out. The Economist (2010-11-18). Retrieved on 2016-12-16.
- ↑ Cynar Plastics to Diesel. Ricardo – AEA Ltd (2013)
- ↑ N.J. Themelis et al. "Energy and Economic Value of Nonrecyclable Plastics and Municipal Solid Wastes that are Currently Landfilled in the Fifty States" Columbia University Earth Engineering Center
- ↑ US EPA. "Tire-Derived Fuel – Scrap Tires". Archived from the original on March 26, 2015. Retrieved 18 February 2015.
- 1 2 3 ผศ.ดร.ศิริรัตน์ จิตการค้า, "ไพโรไลซิสยางรถยนต์หมดสภาพ : กลไกการผลิตน้ำมันเชื้อเพลิงคุณภาพสูง"วิทยาลัยปิโตรเลียมและปิโตรเคมี จุฬาลงกรณ์มหาวิทยาลัย (in Thai) Jidgarnka, S. "Pyrolysis of Expired Car Tires: Mechanics of Producing High Quality Fuels". Chulalongkorn University Department of Petrochemistry
- ↑ Roy, C.; Chaala, A.; Darmstadt, H. (1999). "The vacuum pyrolysis of used tires". Journal of Analytical and Applied Pyrolysis. 51: 201. doi:10.1016/S0165-2370(99)00017-0.
- ↑ Martínez, Juan Daniel; Puy, Neus; Murillo, Ramón; García, Tomás; Navarro, María Victoria; Mastral, Ana Maria (2013). "Waste tyre pyrolysis – A review, Renewable and Sustainable". Energy Reviews. 23: 179–213. doi:10.1016/j.rser.2013.02.038.
- ↑ Choi, G.-G.; Jung, S.-H.; Oh, S.-J.; Kim, J.-S. (2014). "Total utilization of waste tire rubber through pyrolysis to obtain oils and CO2 activation of pyrolysis char". Fuel Processing Technology. 123: 57. doi:10.1016/j.fuproc.2014.02.007.
- ↑ Ringer, M.; Putsche, V.; Scahill, J. (2006) Large-Scale Pyrolysis Oil Production: A Technology Assessment and Economic Analysis; NREL/TP-510-37779; National Renewable Energy Laboratory (NREL), Golden, CO.
- ↑ Vane, C. H.; et al. (2001). "The effect of fungal decay (Agaricus bisporus) on wheat straw lignin using pyrolysis–GC–MS in the presence of tetramethylammonium hydroxide (TMAH)". Journal of Analytical and Applied Pyrolysis. 60 (1): 69–78. doi:10.1016/s0165-2370(00)00156-x.
- ↑ Vane, C. H.; et al. (2003). "Biodegradation of Oak (Quercus alba) Wood during Growth of the Shiitake Mushroom (Lentinula edodes): A Molecular Approach". Journal of Agricultural and Food Chemistry. 51 (4): 947–956. PMID 12568554. doi:10.1021/jf020932h.
- ↑ Heffungs, Udo (June 2010). "Effective Spinneret Cleaning". Fiber Journal. Retrieved 19 April 2016.
- 1 2 3 4 Mainord, Kenneth (September 1994). "Cleaning with Heat: Old Technology with a Bright New Future" (PDF). Pollution Prevention Regional Information Center. The Magazine of Critical Cleaning Technology. Retrieved 4 December 2015.
- 1 2 3 "A Look at Thermal Cleaning Technology". ThermalProcessing.org. Process Examiner. 14 March 2014. Retrieved 4 December 2015.
- ↑ Davis, Gary; Brown, Keith (April 1996). "Cleaning Metal Parts and Tooling" (PDF). Pollution Prevention Regional Information Center. Process Heating. Retrieved 4 December 2015.
- ↑ Schwing, Ewald; Uhrner, Horst (7 October 1999). "Method for removing polymer deposits which have formed on metal or ceramic machine parts, equipment and tools". Espacenet. European Patent Office. Retrieved 19 April 2016.
- ↑ Staffin, Herbert Kenneth; Koelzer, Robert A. (28 November 1974). "Cleaning objects in hot fluidised bed – with neutralisation of resultant acidic gas esp. by alkaline metals cpds". Espacenet. European Patent Office. Retrieved 19 April 2016.
- ↑ Dwan, Thomas S. (2 September 1980). "Process for vacuum pyrolysis removal of polymers from various objects". Espacenet. European Patent Office. Retrieved 26 December 2015.
- ↑ "Vacuum pyrolysis systems". thermal-cleaning.com. Retrieved 11 February 2016.
- ↑ "Paint Stripping: Reducing Waste and Hazardous Material". Minnesota Technical Assistance Program. University of Minnesota. July 2008. Retrieved 4 December 2015.
- ↑ He, Fang; Yi, Weiming; Bai, Xueyuan (September 2006). "Investigation on caloric requirement of biomass pyrolysis using TG–DSC analyzer". Energy Conversion and Management. 47 (15–16): 2461–2469. doi:10.1016/j.enconman.2005.11.011.
- ↑ Briens, Cedric; Berruti, Franco & Piskorz, Jan (2008). "Biomass Valorization for Fuel and Chemicals Production – A Review". International Journal of Chemical Reactor Engineering. 6. doi:10.2202/1542-6580.1674.
- ↑ Bramer, E. A. and Brem, G. A new technology for fast pyrolysis of biomass: development of PyRos reactor. University of Twente
- ↑ Bridgwater, A. V; Meier, D.; Radlein, D. (1999). "An Overview of Fast Pyrolysis of Biomass". Organic Geochemistry. 30: 1479–1493. doi:10.1016/s0146-6380(99)00120-5.
- ↑ "BTG Biomass Technology Group b.v. :: Technology:: Pyrolysis". Archived from the original on 2007-07-03.
- ↑ Lago, Valentina (2015) "Application of Mechanically Fluidized Reactors to Lignin Pyrolysis". Electronic Thesis and Dissertation Repository. Paper 2779.
- ↑ An Awaited Arrival – BioFuelNet. Biofuelnet.ca (2016-04-08). Retrieved on 2016-12-16.
- ↑ "Biomass Feedstock for Slow Pyrolysis". BEST Pyrolysis, Inc. website. BEST Energies, Inc. Retrieved 2010-07-30.
- ↑ Marshall, A. T. & Morris, J. M. (2006) A Watery Solution and Sustainable Energy Parks, CIWM Journal, pp. 22–23
- ↑ "Designer Fuels: The Next Fuel Generation" (PDF). Archived from the original (PDF) on 2006-12-18.
- ↑ Westerhof, Roel Johannes Maria (2011). Refining fast pyrolysis of biomass. Thermo-Chemical Conversion of Biomass (Thesis). University of Twente. Retrieved 2012-05-30.
- ↑ Ramirez, Jerome; Brown, Richard; Rainey, Thomas (1 July 2015). "A Review of Hydrothermal Liquefaction Bio-Crude Properties and Prospects for Upgrading to Transportation Fuels". Energies. 8: 6765–6794. doi:10.3390/en8076765.
External links
-
The dictionary definition of pyrolysis at Wiktionary
- In Situ Catalytic Fast Pyrolysis Technology Pathway National Renewable Energy Laboratory