Pumped-storage hydroelectricity

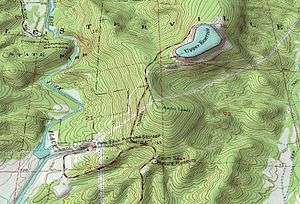
Pumped-storage hydroelectricity (PSH), or pumped hydroelectric energy storage (PHES), is a type of hydroelectric energy storage used by electric power systems for load balancing. The method stores energy in the form of gravitational potential energy of water, pumped from a lower elevation reservoir to a higher elevation. Low-cost surplus off-peak electric power is typically used to run the pumps. During periods of high electrical demand, the stored water is released through turbines to produce electric power. Although the losses of the pumping process makes the plant a net consumer of energy overall, the system increases revenue by selling more electricity during periods of peak demand, when electricity prices are highest.
Pumped-storage hydroelectricity allows energy from intermittent sources (such as solar, wind) and other renewables, or excess electricity from continuous base-load sources (such as coal or nuclear) to be saved for periods of higher demand.[1][2] The reservoirs used with pumped storage are quite small when compared to conventional hydroelectric dams of similar power capacity, and generating periods are often less than half a day.
Pumped storage is the largest-capacity form of grid energy storage available, and, as of 2017, the DOE Global Energy Storage Database reports that PSH accounts for over 96% of all active tracked storage installations worldwide, with a total installed nameplate capacity of over 168 GW.[3] The round-trip energy efficiency of PSH varies between 70%–80%,[4][5][6][7] with some sources claiming up to 87%.[8] The main disadvantage of PHS is the specialist nature of the site required, needing both geographical height and water availability. Suitable sites are therefore likely to be in hilly or mountainous regions, and potentially in areas of outstanding natural beauty, and therefore there are also social and ecological issues to overcome.[9]
Overview

At times of low electrical demand, excess generation capacity is used to pump water into the upper reservoir. When there is higher demand, water is released back into the lower reservoir through a turbine, generating electricity. Reversible turbine/generator assemblies act as a combined pump and turbine generator unit (usually a Francis turbine design). In open-loop systems, pure pumped-storage plants store water in an upper reservoir with no natural inflows, while pump-back plants utilize a combination of pumped storage and conventional hydroelectric plants with an upper reservoir that is replenished in part by natural inflows from a stream or river. Plants that do not use pumped-storage are referred to as conventional hydroelectric plants; conventional hydroelectric plants that have significant storage capacity may be able to play a similar role in the electrical grid as pumped storage by deferring output until needed.
Taking into account evaporation losses from the exposed water surface and conversion losses, energy recovery of 70-80% or more can be regained.[10] This technique is currently the most cost-effective means of storing large amounts of electrical energy, but capital costs and the presence of appropriate geography are critical decision factors in selecting pumped-storage plant sites.
The relatively low energy density of pumped storage systems requires either large flows and/or large differences in height between reservoirs. The only way to store a significant amount of energy is by having a large body of water located relatively near, but as high above as possible, a second body of water. In some places this occurs naturally, in others one or both bodies of water were man-made. Projects in which both reservoirs are artificial and in which no natural inflows are involved with either reservoir are referred to as "closed loop" systems.[11]
These systems may be economical because they flatten out load variations on the power grid, permitting thermal power stations such as coal-fired plants and nuclear power plants that provide base-load electricity to continue operating at peak efficiency, while reducing the need for "peaking" power plants that use the same fuels as many base-load thermal plants, gas and oil, but have been designed for flexibility rather than maximal efficiency. Hence pumped storage systems are crucial when coordinating large groups of heterogeneous generators. However, capital costs for pumped-storage plants are relatively high.

Along with energy management, pumped storage systems help control electrical network frequency and provide reserve generation. Thermal plants are much less able to respond to sudden changes in electrical demand, potentially causing frequency and voltage instability. Pumped storage plants, like other hydroelectric plants, can respond to load changes within seconds.
The most important use for pumped storage has traditionally been to balance baseload powerplants, but may also be used to abate the fluctuating output of intermittent energy sources. Pumped storage provides a load at times of high electricity output and low electricity demand, enabling additional system peak capacity. In certain jurisdictions, electricity prices may be close to zero or occasionally negative on occasions that there is more electrical generation available than there is load available to absorb it; although at present this is rarely due to wind or solar power alone, increased wind and solar generation will increase the likelihood of such occurrences. It is particularly likely that pumped storage will become especially important as a balance for very large scale photovoltaic generation.[12] Increased long distance transmission capacity combined with significant amounts of energy storage will be a crucial part of regulating any large-scale deployment of intermittent renewable power sources.[13] The high non-firm renewable electricity penetration in some regions supplies 40% of annual output, but 60% may be reached before additional storage is necessary.[14][15][16]
There are small-scale installations of such technology, namely in buildings, although these are economically unfeasible given the economies of scale present.[17] Also, a large volume of water is required for a meaningful storage capacity which is a difficult fit for an urban setting.[17] Nevertheless, some authors defend its technological simplicity and secure provision of water as important externalities.[17]
History
The first use of pumped storage was in the 1890s in Italy and Switzerland. In the 1930s reversible hydroelectric turbines became available. These turbines could operate as both turbine-generators and in reverse as electric motor driven pumps. The latest in large-scale engineering technology are variable speed machines for greater efficiency. These machines operate in synchronization with the network frequency when generating, but operate asynchronously (independent of the network frequency) when pumping.
The first use of pumped-storage in the United States was in 1930 by the Connecticut Electric and Power Company, using a large reservoir located near New Milford, Connecticut, pumping water from the Housatonic River to the storage reservoir 230 feet above.[18]
Worldwide use
In 2009, world pumped storage generating capacity was 104 GW,[19] while other sources claim 127 GW, which comprises the vast majority of all types of utility grade electric storage.[20] The EU had 38.3 GW net capacity (36.8% of world capacity) out of a total of 140 GW of hydropower and representing 5% of total net electrical capacity in the EU. Japan had 25.5 GW net capacity (24.5% of world capacity).[19]
In 2010 the United States had 21.5 GW of pumped storage generating capacity (20.6% of world capacity).[21] PHS generated (net) -5.501 GWh of energy in 2010 in the US[22] because more energy is consumed in pumping than is generated. Nameplate pumped storage capacity had grown to 21.6 GW by 2014, with pumped storage comprising 97% of grid-scale energy storage in the US. As of late 2014, there were 51 active project proposals with a total of 39 GW of new nameplate capacity across all stages of the FERC licensing process for new pumped storage hydroelectric plants in the US, but no new plants were currently under construction in the US at the time.[23][24]
The five largest operational pumped-storage plants are listed below (for a detailed list see List of pumped-storage hydroelectric power stations):
Station | Country | Location | Capacity (MW) | Refs |
---|---|---|---|---|
Bath County Pumped Storage Station | United States | 38°12′32″N 79°48′00″W / 38.20889°N 79.80000°W | 3,003 | [25] |
Guangdong Pumped Storage Power Station | China | 23°45′52″N 113°57′12″E / 23.76444°N 113.95333°E | 2,400 | [26][27] |
Huizhou Pumped Storage Power Station | China | 23°16′07″N 114°18′50″E / 23.26861°N 114.31389°E | 2,400 | [28][29][30][31] |
Okutataragi Pumped Storage Power Station | Japan | 35°14′13″N 134°49′55″E / 35.23694°N 134.83194°E | 1,932 | [32] |
Ludington Pumped Storage Power Plant | United States | 43°53′37″N 86°26′43″W / 43.89361°N 86.44528°W | 1,872 | [33][34] |
Note: this table shows the power-generating capacity in megawatts as is usual for power stations. However, the overall energy-storage capacity in megawatt-hours (MWh) is a different intrinsic property and can not be derived from the above given figures. |
Pump-back hydroelectric dams
Conventional hydroelectric dams may also make use of pumped storage in a hybrid system that both generates power from water naturally flowing into the reservoir as well as storing water pumped back to the reservoir from below the dam. The Grand Coulee Dam in the US was expanded with a pump-back system in 1973.[35] Existing dams may be repowered with reversing turbines thereby extending the length of time the plant can operate at capacity. Optionally a pump back powerhouse such as the Russell Dam (1992) may be added to a dam for increased generating capacity. Making use of an existing dams upper reservoir and transmission system can expedite projects and reduce costs.
Potential technologies
Seawater
Pumped storage plants can operate with seawater, although there are additional challenges compared to using fresh water. In 1999, the 30 MW Yanbaru project in Okinawa was the first demonstration of seawater pumped storage. The 300 MW seawater-based Lanai Pumped Storage Project has recently been proposed on Lanai, Hawaii, and seawater-based projects have been proposed in Ireland.[36] A pair of proposed projects in the Atacama Desert in northern Chile would use 600 MW of photovoltaic solar (Skies of Tarapacá) together with 300 MW of pumped storage (Mirror of Tarapacá) raising seawater 600 metres (2,000 ft) up a coastal cliff.[37][38]
Underground reservoirs
The use of underground reservoirs has been investigated. Recent examples include the proposed Summit project in Norton, Ohio, the proposed Maysville project in Kentucky (underground limestone mine), and the Mount Hope project in New Jersey, which was to have used a former iron mine as the lower reservoir. Several new underground pumped storage projects have been proposed. Cost-per-kilowatt estimates for these projects can be lower than for surface projects if they use existing underground mine space. There are limited opportunities involving suitable underground space, but the number of underground pumped storage opportunities may increase if abandoned coal mines prove suitable.[39]
Decentralised systems
Small pumped-storage hydropower plants can be built on streams and within infrastructures, such as drinking water networks[40] and artificial snow making infrastructures. Such plants provide distributed energy storage and distributed flexible electricity production and can contribute to the decentralized integration of intermittent renewable energy technologies, such as wind power and solar power. Reservoirs that can be used for small pumped-storage hydropower plants could include[41] natural or artificial lakes, reservoirs within other structures such as irrigation, or unused portions of mines or underground military installations. In Switzerland one study suggested that the total installed capacity of small pumped-storage hydropower plants in 2011 could be increased by 3 to 9 times by providing adequate policy instruments.[41]
Underwater reservoirs
In March 2017 the research project StEnSea (Storing Energy at Sea) announced their successful completion of a four-week test of a pumped storage underwater reservoir. In this configuration a hollow sphere submerged in deep water acts as the lower reservoir while the upper reservoir is the enclosing body of water. When a reversible turbine integrated into the sphere uses surplus electricity to pump water out of the sphere the force of the pump must act on the entire column of water above the sphere, so the deeper the sphere is located, the more potential energy it can store and convert back to electricity by letting water back in via the turbine.
As such the energy storage capacity of the submerged reservoir is not governed by the gravitational energy in the traditional sense, but rather by the vertical pressure variation.
While StEnSea's test took place at a depth of 100 m in the fresh water Lake Constance, the technology is foreseen to be used in salt water at greater depths. Since the submerged reservoir needs only a connecting electrical cable, the depth at which it can be employed is limited only by the depth at which the turbine can function, currently limited to 700 m. The challenge of designing salt water pumped storage in this underwater configuration brings a range of advantages:
- No land area is required,
- No mechanical structure other than the electrical cable needs to span the distance of the potential energy difference,
- In the presence of sufficient seabed area multiple reservoirs can scale the storage capacity without limits,
- Should a reservoir collapse, the consequences would be limited apart from the loss of the reservoir itself,
- Evaporation from the upper reservoir has no effect on the energy conversion efficiency,
- Transmission of electricity between the reservoir and the grid can be established from a nearby offshore wind farm limiting transmission loss and obviating the need for onshore cabling permits.
A current commercial design featuring a sphere with an inner diameter of 30 m submerged to 700 m would correspond to a 20 MWh capacity which with a 5 MW turbine would lead to a 4-hour discharge time. An energy park with multiple such reservoirs would bring the storage cost to around a few eurocents per kWh with construction and equipment costs in the range €1,200-€1,400 per kW. To avoid excessive transmission cost and loss, the reservoirs should be placed off deep water coasts of densely populated areas, such as Norway, Spain, USA and Japan. With this limitation the concept would allow for worldwide electricity storage of close to 900 GWh.[42][43]
For comparison, a traditional, gravity-based pumped storage capable of storing 20 MWh in a water reservoir the size of a 30 m sphere would need a hydraulic head of 519 m with the elevation spanned by a pressurized water pipe requiring typically a hill or mountain for support.
See also
References
- ↑ http://poppware.de/Storage_for_a_secure_Power_Supply_from_Wind_and_Sun.pdf
- ↑ https://www.researchgate.net/publication/271539381_Pumped_hydro_energy_storage_system_A_technological_review
- ↑ "DOE Global Energy Storage Database". www.energystorageexchange.org. Retrieved 10 February 2017.
- ↑ "Energy storage - Packing some power". The Economist. 2011-03-03. Retrieved 2012-03-11.
- ↑ Jacob, Thierry.Pumped storage in Switzerland - an outlook beyond 2000 Stucky. Accessed: 13 February 2012.
- ↑ Levine, Jonah G. Pumped Hydroelectric Energy Storage and Spatial Diversity of Wind Resources as Methods of Improving Utilization of Renewable Energy Sources page 6, University of Colorado, December 2007. Accessed: 12 February 2012.
- ↑ Yang, Chi-Jen. Pumped Hydroelectric Storage Duke University. Accessed: 12 February 2012.
- ↑ "Energy Storage". Archived from the original on 18 November 2015. Retrieved 26 February 2017.
- ↑ European Renewable Energy Network pp. 188
- ↑ "Pumped Hydroelectric Storage | Energy Storage Association". energystorage.org. Retrieved 15 January 2017.
- ↑ "FERC: Hydropower - Pumped Storage Projects". www.ferc.gov. Retrieved 15 January 2017.
- ↑ Summary Energy from the Desert - Practical Proposals for Very Large Scale Photovoltaic Power Generation (VLS-PV) Systems
- ↑ http://www.nrel.gov/docs/fy17osti/67240.pdf
- ↑ "German grid operator sees 70% wind + solar before storage needed". Renew Economy. 7 December 2015. Retrieved 20 January 2017.
Schucht says, in the region he is operating in, 42 per cent of the power supply (in output, not capacity), came from wind and solar – about the same as South Australia. Schucht believes that integration of 60 to 70 per cent variable renewable energy – just wind and solar – could be accommodated within the German market without the need for additional storage. Beyond that, storage will be needed.
- ↑ Dehmer, Dagmar (8 June 2016). "German electricity transmission CEO: ‘80% renewables is no problem’". Der Tagesspiegel / EurActiv.com. Retrieved 1 February 2017.
There are a certain number of myths in the energy industry. One of them is that we need more flexibility in the system to integrate renewables, like energy storage, interruptible loads or backup power plants. That’s a myth. We are well on track to having a system that can accommodate between 70-80% renewable energy without the need for more flexibility options.
- ↑ "New record-breaking year for Danish wind power". Energinet.dk. 15 January 2016.
- 1 2 3 de Oliveira e Silva, Guilherme; Hendrick, Patrick (2016-10-01). "Pumped hydro energy storage in buildings". Applied Energy. 179: 1242–1250. doi:10.1016/j.apenergy.2016.07.046.
- ↑ "A Ten-Mile Storage Battery." Popular Science, July 1930, p. 60.
- 1 2 International Energy Statistics
- ↑ Rastler et al. Electric Energy Storage Technology Options: A White Paper Primer on Applications, Costs, and Benefits. (Free download) EPRI, Palo Alto, CA, 2010. Accessed: 30 September 2011. Mirror
- ↑ http://www.eia.doe.gov/oiaf/servicerpt/stimulus/excel/aeostimtab_9.xls
- ↑ http://www.eia.gov/electricity/monthly/pdf/chap1.pdf Table 1.1
- ↑ "2014 Hydropower Market Report Highlights" (PDF). U.S. Department of Energy. Retrieved 19 February 2017.
- ↑ "2014 Hydropower Market Report" (PDF). U.S. Department of Energy. Retrieved 19 February 2017.
- ↑ Bath County Pumped-storage Station, retrieved 2011-12-30
- ↑ Pumped-storage hydroelectric power stations in China, retrieved 2010-06-25
- ↑ Guangzhou Pumped-storage Power Station (PDF), retrieved 2010-06-25
- ↑ List of pumped-storage power plants in China 1 (Mandarin)
- ↑ List of pumped-storage power plants in China 2 (Mandarin)
- ↑ List of pumped-storage power plants in China 3 (Mandarin)
- ↑ Huizhou Pumped-storage Power Station, retrieved 2010-06-25
- ↑ "2003-2004 Electricity Review in Japan" (PDF). Japan Nuclear. Retrieved 1 September 2010.
- ↑ Dniester Pumped Storage Plant, Ukraine, retrieved 2010-09-01
- ↑ Tymoshenko launches the first unit of Dnister Hydroelectric Power Plant, retrieved 2010-09-01
- ↑ Alternative Energy and Shale Gas Encyclopedia page 424
- ↑ http://www.sciencemag.org/news/2012/02/massive-energy-storage-courtesy-west-ireland
- ↑ "Project Espejo de Tarapacá". Valhalla. Retrieved 19 June 2017.
- ↑ http://www.power-technology.com/features/featurethe-mirror-of-tarapaca-chilean-power-project-harnesses-both-sun-and-sea-4872272/
- ↑ "German Coal Mine to Be Reborn as Giant Pumped Storage Hydro Facility". Retrieved 20 March 2017.
- ↑ "Senator Wash". www.iid.com. Imperial Irrigation District. Retrieved 6 August 2016.
- 1 2 Crettenand, N. (2012) "The facilitation of mini and small hydropower in Switzerland: shaping the institutional framework. With a particular focus on storage and pumped-storage schemes". Ecole Polytechnique Fédérale de Lausanne (EPFL). PhD Thesis N° 5356. http://infoscience.epfl.ch/record/176337?ln=en
- ↑ "Storing energy at sea". forschung-energiespeicher.info. 2016-10-17. Retrieved 2017-03-06.
- ↑ "Unterwasser-Pumpspeicherkraftwerk erfolgreich getestet" [Underwater Pumped Storage Powerplant successfully tested]. Fraunhofer Institute for Wind Energy and Energy System Technology (in German). 2017-03-03. Retrieved 2017-03-06.
External links
![]() |
Wikimedia Commons has media related to Pumped-storage hydroelectric power plants. |