Propionyl-CoA carboxylase
Propionyl-CoA carboxylase | |||||||||
---|---|---|---|---|---|---|---|---|---|
Identifiers | |||||||||
EC number | 6.4.1.3 | ||||||||
CAS number | 9023-94-3 | ||||||||
Databases | |||||||||
IntEnz | IntEnz view | ||||||||
BRENDA | BRENDA entry | ||||||||
ExPASy | NiceZyme view | ||||||||
KEGG | KEGG entry | ||||||||
MetaCyc | metabolic pathway | ||||||||
PRIAM | profile | ||||||||
PDB structures | RCSB PDB PDBe PDBsum | ||||||||
Gene Ontology | AmiGO / EGO | ||||||||
|
Methylmalonyl-CoA decarboxylase | |||||||||
---|---|---|---|---|---|---|---|---|---|
Identifiers | |||||||||
EC number | 4.1.1.41 | ||||||||
CAS number | 37289-44-4 | ||||||||
Databases | |||||||||
IntEnz | IntEnz view | ||||||||
BRENDA | BRENDA entry | ||||||||
ExPASy | NiceZyme view | ||||||||
KEGG | KEGG entry | ||||||||
MetaCyc | metabolic pathway | ||||||||
PRIAM | profile | ||||||||
PDB structures | RCSB PDB PDBe PDBsum | ||||||||
Gene Ontology | AmiGO / EGO | ||||||||
|
Propionyl-CoA carboxylase catalyses the carboxylation reaction of propionyl CoA in the mitochondrial matrix. The enzyme is biotin-dependent. The product of the reaction is (S)-methylmalonyl CoA. Propionyl CoA is the end product of metabolism of odd-chain fatty acids, and is also a metabolite of most methyl-branched fatty acids. It is also the main metabolite of valine, and together with acetyl-CoA, is a metabolite of isoleucine, as well as a methionine metabolite. Propionyl-CoA is thus of great importance as a glucose precursor. (S)-Methylmalonyl-CoA is not directly utilizable by animals; it is acted on by a racemase to give (R)-methylmalonyl-CoA. The latter is converted by methylmalonyl-CoA mutase (one of a very few Vitamin B12-dependent enzymes) to give succinyl-CoA. The latter is converted to oxaloacetate and then malate in the Krebs cycle. Export of malate into the cytosol leads to formation of oxaloacetate, phosphoenol pyruvate, and other gluconeogenic intermediates.
- ATP + propionyl-CoA + HCO3− <=> ADP + phosphate + (S)-methylmalonyl-CoA
It has been classified both as a ligase[1] and a lyase.[2]
Enzyme Structure
Propionyl-CoA Carboxylase (PCC) is a 750 kDa alpha(6)-beta(6)-dodecamer. (Only approximately 540 kDa is native enzyme.[3] ) The alpha subunits are arranged as monomers, decorating the central beta-6 hexameric core. Said core is oriented as a short cylinder with a hole along its axis.
The alpha subunit of PCC contains the biotin carboxylase (BC) and biotin carboxyl carrier protein (BCCP) domains. A domain known as the BT domain is also located on the alpha subunit and is essential for interactions with the beta subunit. The 8-stranded anti-parallel beta barrel fold of this domain is particularly interesting. The beta subunit contains the carboxyltransferase (CT) activity.[4]
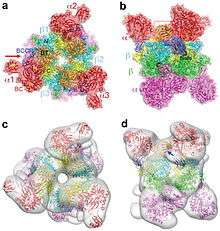
The BC and CT sites are approximately 55 Å apart, indicative of the entire BCCP domain translocating during catalysis of the carboxylation of propionyl-CoA.[5] This provides clear evidence of crucial dimeric interaction between alpha and beta subunits.
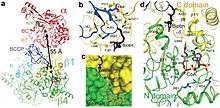
The biotin-binding pocket of PCC is hydrophobic and highly conserved. Biotin and propionyl-CoA bind perpendicular to each other in the oxyanion hole-containing active site. The native enzyme to biotin ratio has been determined to be one mole native enzyme to 4 moles biotin.[3] The N1 of biotin is thought to be the active site base.[4]
Site-directed mutagenesis at D422 shows a change in the substrate specificity of the propionyl-CoA binding site, thus indicating this residue’s importance in PCC’s catalytic activity.[6] In 1979, inhibition by phenylglyoxal determined that a phosphate group from either propionyl-CoA or ATP reacts with an essential arginine residue in the active site during catalysis.[7] Later (2004), it was suggested that Arginine-338 serves to orient the carboxyphosphate intermediate for optimal carboxylation of biotin.[8]
The KM values for ATP, propionyl-CoA, and bicarbonate has been determined to be 0.08 mM, 0.29 mM, and 3.0 mM, respectively. The isoelectric point falls at pH 5.5. PCC’s structural integrity is conserved over the temperature range of -50 to 37 degrees Celsius and the pH range of 6.2 to 8.8. Optimum pH was shown to be between 7.2 and 8.8 without biotin bound.[3] With biotin, optimum pH is 8.0-8.5.[9]
Enzyme Mechanism
The normal catalytic reaction mechanism involves a carbanion intermediate and does not proceed through a concerted process.[10] Figure 3 shows a probable pathway.

The reaction has been shown to be slightly reversible at low propionyl-CoA flux.[11]
Isozymes
Humans express the following two propionyl-CoA carboxylase isozymes:
|
|
Pathology
A deficiency is associated with propionic acidemia.[12][13][14]
PCC activity is the most sensitive indicator of biotin status tested to date. In future pregnancy studies, the use of lymphocyte PCC activity data should prove valuable in assessment of biotin status.[15]
Regulation
Of Propionyl-CoA Carboxylase
a. Carbamazepine (antiepileptic drug): significantly lowers enzyme levels in the liver[16]
b. E. coli chaperonin proteins groES and groEL: essential for folding and assembly of human PCC heteromeric subunits[17]
c. Bicarbonate: negative cooperativity[8]
d. Mg2+ and MgATP2−: allosteric activation[18]
By Propionyl-CoA Carboxylase
a. 6-Deoxyerythronolide B: decrease in PCC levels lead to increased production [19]
b. Glucokinase in pancreatic beta cells: precursor of beta-PCC shown to decrease KM and increase Vmax; activation [20]
See also
References
- ↑ EC 6.4.1.3
- ↑ EC 4.1.1.41
- 1 2 3 Kalousek F, Darigo MD, Rosenberg LE (1980). "Isolation and characterization of propionyl-CoA carboxylase from normal human liver. Evidence for a protomeric tetramer of nonidentical subunits". The Journal of Biological Chemistry. 255 (1): 60–65. PMID 6765947.
- 1 2 Diacovich L, Mitchell DL, Pham H, Gago G, Melgar MM, Khosla C, Gramajo H, Tsai SC (2004). "Crystal Structure of theβ-Subunit of Acyl-CoA Carboxylase: Structure-Based Engineering of Substrate Specificity†,‡". Biochemistry. 43 (44): 14027–14036. PMID 15518551. doi:10.1021/bi049065v.
- 1 2 3 Huang CS, Sadre-Bazzaz K, Shen Y, Deng B, Zhou ZH, Tong L (2010). "Crystal structure of the α6β6 holoenzyme of propionyl-coenzyme a carboxylase". Nature. 466 (7309): 1001–1005. PMC 2925307
. PMID 20725044. doi:10.1038/nature09302.
- ↑ Arabolaza A, Shillito ME, Lin TW, Diacovich L, Melgar M, Pham H, Amick D, Gramajo H, Tsai SC (2010). "Crystal Structures and Mutational Analyses of Acyl-CoA Carboxylase β Subunit ofStreptomyces coelicolor". Biochemistry. 49 (34): 7367–7376. PMC 2927733
. PMID 20690600. doi:10.1021/bi1005305.
- ↑ Wolf B, Kalousek F, Rosenberg LE (1979). "Essential arginine residues in the active sites of propionyl CoA carboxylase and beta-methylcrotonyl CoA carboxylase". Enzyme. 24 (5): 302–306. PMID 510274.
- 1 2 Sloane V, Waldrop GL (2004). "Kinetic characterization of mutations found in propionic acidemia and methylcrotonylglycinuria: evidence for cooperativity in biotin carboxylase.". Journal of Biological Chemistry. 279 (16): 15772–15778. PMID 14960587. doi:10.1074/jbc.M311982200.
- ↑ Hsia YE, Scully KJ, Rosenberg LE (1979). "Human propionyl CoA carboxylase: Some properties of the partially purified enzyme in fibroblasts from controls and patients with propionic acidemia". Pediatric research. 13 (6): 746–751. PMID 481943. doi:10.1203/00006450-197906000-00005.
- ↑ Stubbe J, Fish S, Abeles RH (1980). "Are carboxylations involving biotin concerted or nonconcerted?". The Journal of Biological Chemistry. 255 (1): 236–242. PMID 7350155.
- ↑ Reszko AE, Kasumov T, Pierce BA, David F, Hoppel CL, Stanley WC, Des Rosiers C, Brunengraber H (2003). "Assessing the Reversibility of the Anaplerotic Reactions of the Propionyl-CoA Pathway in Heart and Liver". Journal of Biological Chemistry. 278 (37): 34959–34965. PMID 12824185. doi:10.1074/jbc.M302013200.
- ↑ Ugarte M, Pérez-Cerdá C, Rodríguez-Pombo P, Desviat LR, Pérez B, Richard E, Muro S, Campeau E, Ohura T, Gravel RA (1999). "Overview of mutations in thePCCA andPCCB genes causing propionic acidemia". Human Mutation. 14 (4): 275–282. PMID 10502773. doi:10.1002/(SICI)1098-1004(199910)14:4<275::AID-HUMU1>3.0.CO;2-N.
- ↑ Desviat LR, Pérez B, Pérez-Cerdá C, Rodríguez-Pombo P, Clavero S, Ugarte M (2004). "Propionic acidemia: Mutation update and functional and structural effects of the variant alleles". Molecular Genetics and Metabolism. 83 (1–2): 28–37. PMID 15464417. doi:10.1016/j.ymgme.2004.08.001.
- ↑ Deodato, F.; Boenzi, S.; Santorelli, F. M.; Dionisi-Vici, C. (2006). "Methylmalonic and propionic aciduria". American Journal of Medical Genetics Part C. 142C (2): 104–112. PMID 16602092. doi:10.1002/ajmg.c.30090.
- ↑ Stratton SL, Bogusiewicz A, Mock MM, Mock NI, Wells AM, Mock DM (2006). "Lymphocyte propionyl-CoA carboxylase and its activation by biotin are sensitive indicators of marginal biotin deficiency in humans". The American Journal of Clinical Nutrition. 84 (2): 384–388. PMC 1539098
. PMID 16895887.
- ↑ Rathman SC, Eisenschenk S, McMahon RJ (2002). "The abundance and function of biotin-dependent enzymes are reduced in rats chronically administered carbamazepine". The Journal of Nutrition. 132 (11): 3405–3410. PMID 12421859.
- ↑ Kelson TL, Ohura T, Kraus JP (1996). "Chaperonin-mediated assembly of wild-type and mutant subunits of human propionyl-CoA carboxylase expressed in Escherichia coli". Human Molecular Genetics. 5 (3): 331–337. PMID 8852656. doi:10.1093/hmg/5.3.331.
- ↑ McKeon C, Wolf B (1982). "Magnesium and magnesium adenosine triphosphate activation of human propionyl CoA carboxylase and beta-methylcrotonyl CoA carboxylase". Enzyme. 28 (1): 76–81. PMID 6981505.
- ↑ Zhang H, Boghigian BA, Pfeifer BA (2010). "Investigating the role of native propionyl-CoA and methylmalonyl-CoA metabolism on heterologous polyketide production inEscherichia coli". Biotechnology and Bioengineering. 105 (3): 567–573. PMID 19806677. doi:10.1002/bit.22560.
- ↑ Shiraishi A, Yamada Y, Tsuura Y, Fijimoto S, Tsukiyama K, Mukai E, Toyoda Y, Miwa I, Seino Y (2000). "A novel glucokinase regulator in pancreatic beta cells: precursor of propionyl-CoA carboxylase beta subunit interacts with glucokinase and augments its activity.". Journal of Biological Chemistry. 276 (4): 2325–2328. PMID 11085976. doi:10.1074/jbc.C000530200.
External links
- Propionyl-CoA Carboxylase at the US National Library of Medicine Medical Subject Headings (MeSH)