Molecular wire
Part of a series of articles on |
Nanoelectronics |
---|
Single-molecule electronics |
Solid state nanoelectronics |
Related approaches |
|
Molecular wires (or sometimes called molecular nanowires) are molecular chains that conduct electric current. They are the proposed building blocks for molecular electronic devices. Their typical diameters are less than three nanometers, while their lengths may be macroscopic, extending to centimeters or more.
Examples
Most types of molecular wires are derived from organic molecules. One naturally occurring molecular wire is DNA. Prominent inorganic examples include polymeric materials such as Li2Mo6Se6[1] and Mo6S9−xIx,[2][3][4] [Pd4(CO)4(OAc)4Pd(acac)2],[5] and single-molecule extended metal atom chains (EMACs) which comprise strings of transition metal atoms directly bonded to each other.[6] Molecular wires containing paramagnetic inorganic moieties can exhibit Kondo peaks.
Structure
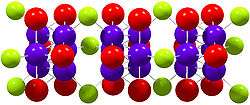
Unlike the more usual nanowires (which are very thin crystals), molecular nanowires are composed of repeating monomers. In the case of DNA, the repeat units are the nucleotides with a backbone made of sugars and phosphate groups joined by ester bonds. Attached to each sugar is one of four types of bases. In case of Mo6S9−xIx, the repeat units are Mo6S9−xIx clusters, which are joined together by flexible sulfur or iodine bridges. Molecular nanowires can be manipulated and investigated as single molecules, but they often aggregate in solution into swatches or bundles. In the case of the Mo chalcogenide-halides, they grow in the form of ordered strands, in which the individual strands are linked by very weak Van der Waals forces. Individual molecules can be manipulated, ordered and their length can be controlled with atomic force microsope tips.
Conduction of electrons
Molecular wires conduct electricity. They typically have non-linear current-voltage characteristics, and do not behave as simple ohmic conductors. The conductance follows typical power law behavior as a function of temperature or electric field, whichever is the greater, arising from their strong one-dimensional character. Numerous theoretical ideas have been used in an attempt to understand the conductivity of one-dimensional systems, where strong interactions between electrons lead to departures from normal metallic (Fermi liquid) behavior. Important concepts are those introduced by Tomonaga, Luttinger and Wigner. Effects caused by classical Coulomb repulsion (called Coulomb blockade), interactions with vibrational degrees of freedom (called phonons) and Quantum Decoherence [7] have also been found to be important in determining the properties of molecular wires.
Nanowires in molecular electronics
To be of use for connecting molecules, MWs need to self-assemble following well-defined routes and form reliable electrical contacts between them. To reproducibly self-assemble a complex circuit based on single molecules. Ideally, they would connect to diverse materials, such as gold metal surfaces (for connections to outside world), biomolecules (for nanosensors, nanoelectrodes, molecular switches) and most importantly, they must allow branching. The connectors should also be available of pre-determined diameter and length. They should also have covalent bonding to ensure reproducible transport and contact properties. DNA-like molecules have specific molecular-scale recognition and can be used in molecular scaffold fabrication. Complex shapes have been demonstrated, but unfortunately metal coated DNA which is electrically conducting is too thick to connect to individual molecules. Thinner coated DNA lacks electronic connectivity and is unsuited for connecting molecular electronics components. Some varieties of carbon nanotubes (CNTs) are conducting, and connectivity at their ends can be achieved by attachment of connecting groups. Unfortunately manufacturing CNTs with pre-determined properties is impossible at present, and the functionalized ends are typically not conducting, limiting their usefulness as molecular connectors. Individual CNTs can be soldered in an electron microscope, but the contact is not covalent and cannot be self-assembled. Possible routes for the construction of larger functional circuits using Mo6S9−xIx MWs have been demonstrated, either via gold nanoparticles as linkers, or by direct connection to thiolated molecules. The two approaches may lead to different possible applications. The use of GNPs offers the possibility of branching and construction of larger circuits.
Other research
Molecular wires can be incorporated into polymers, enhancing their mechanical and/or conducting properties. The enhancement of these properties relies on uniform dispersion of the wires into the host polymer. MoSI wires have been made in such composites, relying on their superior solubility within the polymer host compared to other nanowires or nanotubes. Bundles of wires can be used to enhance tribological properties of polymers, with applications in actuators and potentiometers. It has been recently proposed that twisted nanowires could work as electromechanical nanodevices (or torsion nanobalances) to measure forces and torques at nanoscale with great precision.[8]
References
- ↑ Tarascon, J.M.; Hull, G.W.; Disalvo, F.J. (1984). "A facile synthesis of pseudo one-monodimensional ternary molybdenum chalcogenides M2Mo6X6 (X = Se,Te; M = Li,Na..Cs)". Mater. Res. Bull. 19 (7): 915–924. doi:10.1016/0025-5408(84)90054-0.
- ↑ Vrbani, Daniel; Rem Kar, Maja; Jesih, Adolf; Mrzel, Ale; Umek, Polona; Ponikvar, Maja; Jan Ar, Bo Tjan; Meden, Anton; Novosel, Barbara; Pejovnik, Stane; Venturini, Peter; Coleman, J C; Mihailovi, Dragan (2004). "Air-stable monodispersed Mo6S3I6 nanowires". Nanotechnology. 15 (5): 635–638. Bibcode:2004Nanot..15..635V. doi:10.1088/0957-4484/15/5/039.
- ↑ Perrin, C. & Sergent, M. (1983). "A new family of monodimensional compounds with octahedral molybdenum clusters: Mo6X8Y2". J. Chem. Res. 5: 38–39.
- ↑ D. Mihailovic (2009). "Inorganic molecular wires: Physical and functional properties of transition metal chalco-halide polymers". Progress in Materials Science. 54 (3): 309–350. doi:10.1016/j.pmatsci.2008.09.001.
- ↑ Yin, Xi; Warren, Steven A.; Pan, Yung-Tin; Tsao, Kai-Chieh; Gray, Danielle L.; Bertke, Jeffery; Yang, Hong (2014). "A Motif for Infinite Metal Atom Wires". Angewandte Chemie International Edition. 53: 14087–14091. PMID 25319757. doi:10.1002/anie.201408461.
- ↑ Cotton, F. Albert; Murillo, Carlos A. & Walton, Richard A. (2005). Multiple Bonds Between Metal Atoms (3 ed.). Springer. pp. 669–706. ISBN 0-387-25829-9.
- ↑ Cattena, C. J.; Bustos-Marun, R. A.; Pastawski, H. M. (2010). "Crucial role of decoherence for electronic transport in molecular wires: Polyaniline as a case study". Physical Review B. 82 (14): 144201. Bibcode:2010PhRvB..82n4201C. arXiv:1004.5552
. doi:10.1103/PhysRevB.82.144201.
- ↑ Garcia, J. C.; Justo, J. F. (2014). "Twisted ultrathin silicon nanowires: A possible torsion electromechanical nanodevice". Europhys. Lett. 108: 36006. Bibcode:2014EL....10836006G. doi:10.1209/0295-5075/108/36006.