Molecularly imprinted polymer
A Molecularly Imprinted Polymer (MIP) is a polymer that has been processed using the molecular imprinting technique which leaves cavities in polymer matrix with affinity to a chosen "template" molecule. The process usually involves initiating the polymerization of monomers in the presence of a template molecule that is extracted afterwards, thus leaving complementary cavities behind. These polymers have affinity for the original molecule and have been used in applications such as chemical separations, catalysis, or molecular sensors. Published works on the topic date to the 1930s.[1]
Molecular Imprinting technique (State of the art and perspectives)
Molecular imprinting is, in fact, making an artificial tiny lock for a specific molecule that serve as miniature key. Like plastic receptors the imprinted polymer grabs specific chemicals. Many basic biological processes, from sensing of odors to signaling between nerve and muscle cells, rely on such lock-and-key combinations. For decades, scientists trying to understand these interactions often play locksmith, searching for the right key to fit a particular receptor. Now, the elegance of molecular imprinting in nature has been spurring many scientists to build the locks themselves. They etch a material to create specific cavities which in size, shape and functional groups, fit the target molecule. However, one of the greatest advantages of artificial receptors over naturally occurring ones is freedom of molecular design. Their frameworks are never restricted to proteins, and a variety of skeletons (e.g., carbon chains and fused aromatic rings) can be used. Thus, the stability, flexibility, and other properties are freely modulated according to need. Even functional groups that are not found in nature can be employed in these man-made compounds. Furthermore, when necessary, the activity in response towards outer stimuli (photo-irradiation, pH change, electric or magnetic field, and others) can be provided by using appropriate functional groups. The spectrum of functions is far wider than that of naturally occurring ones. In a molecular imprinting processes, one needs a 1) template, 2) functional monomer(s) 3) cross-linker, 4) initiator, 5) porogenic solvent and 6) extraction solvent. According to polymerization method and final polymer format one or some of the reagent can be avoided.[2]
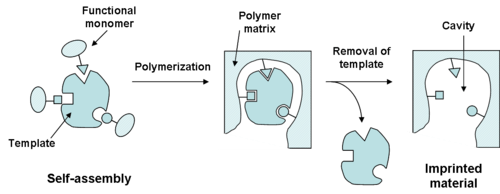
There are two main methods for creating these specialized polymers. The first is known as self-assembly, which involves the formation of polymer by combining all elements of the MIP and allowing the molecular interactions to form the cross-linked polymer with the template molecule bound. The second method of formation of MIPs involves covalently linking the imprint molecule to the monomer. After polymerization, the monomer is cleaved from the template molecule.[3] The selectivity is greatly influenced by the kind and amount of cross-linking agent used in the synthesis of the imprinted polymer. The selectivity is also determined by the covalent and non-covalent interactions between the target molecule and monomer functional groups. The careful choice of functional monomer is another important choice to provide complementary interactions with the template and substrates.[4] In an imprinted polymer, the cross-linker fulfills three major functions: First of all, the cross-linker is important in controlling the morphology of the polymer matrix, whether it is gel-type, macroporous or a microgel powder. Secondly, it serves to stabilize the imprinted binding site. Finally, it imparts mechanical stability to the polymer matrix. From a polymerization point of view, high cross-link ratios are generally preferred in order to access permanently porous materials and in order to be able to generate materials with adequate mechanical stability.
The self-assembly method has advantages in the fact that it forms a more natural binding site, and also offers additional flexibility in the types of monomers that can be polymerized. The covalent method has its advantages in generally offering a high yield of homogeneous binding sites, but first requires the synthesis of a derivatized imprint molecule and may not imitate the "natural" conditions that could be present elsewhere.[5] Over the recent years, interest in the technique of molecular imprinting has increased rapidly, both in the academic community and in the industry. Consequently, significant progress has been made in developing polymerization methods that produce adequate MIP formats with rather good binding properties expecting an enhancement in the performance or in order to suit the desirable final application, such as beads, films or nanoparticles. One of the key issues that have limited the performance of MIPs in practical applications so far is the lack of simple and robust methods to synthesize MIPs in the optimum formats required by the application. Chronologically, the first polymerization method encountered for MIP was based on "bulk" or solution polymerization. This method is the most common technique used by groups working on imprinting especially due to its simplicity and versatility. It is used exclusively with organic solvents mainly with low dielectric constant and consists basically of mixing all the components (template, monomer, solvent and initiator) and subsequently polymerizing them. The resultant polymeric block is then pulverized, freed from the template, crushed and sieved to obtain particles of irregular shape and size between 20 and 50 µm. Depending on the target (template) type and the final application of the MIP, MIPs are appeared in different formats such as nano/micro spherical particles, nanowires and thin film or membranes. They are produced with different polymerization techniques like bulk, precipitation, emulsion, suspension, dispersion, gelation, and multi-step swelling polymerization. Most of investigators in the field of MIP are making MIP with heuristic techniques such as hierarchical imprinting method. The technique for the first time was used for making MIP by Sellergren et al.[6] for imprinting small target molecules. With the same concept, Nematollahzadeh et al.[7] developed a general technique, so-called polymerization packed bed, to obtain a hierarchically structured high capacity protein imprinted porous polymer beads by using silica porous particles for protein recognition and capture.
Applications
Niche areas for application of MIPs are in sensors and separation. Despite the current good health of molecular imprinting in general, one difficulty which appears to remain to this day is the commercialization of molecularly imprinted polymers. Even though no molecularly imprinted silica product has reached the market yet, at least several patents (123 patents, up to 2010, according to Scifinder data base), on molecular imprinting, were held by different groups. That some commercial interest existed is also confirmed by the fact that MIP Technologies,[8] offers a range of commercially available MIP products and Sigma-Aldrich produces SupelMIP for Beta-agonists, Beta-blockers, pesticides and some drugs of abuse such as Amphetamine and that POLYINTELL[9] designs, manufactures and markets AFFINIMIPSPE products[10] for instance for mycotoxins such as Patulin, Zearalenone, Fumonisins, Ochratoxin A, for endocrine disruptors (Bisphenol A, Estrogens derivatives etc...) or for the purification of radiotracers before their use in Positron emission tomography (PET).
Fast and cost-effective molecularly imprinted polymer technique has applications in many fields of chemistry, biology and engineering, particularly as an affinity material for sensors,[11] detection of chemical, antimicrobial, and dye, residues in food, adsorbents for solid phase extraction, binding assays, artificial antibodies, chromatographic stationary phase, catalysis, drug development and screening, and byproduct removal in chemical reaction.[12] Molecular imprinted polymers pose this wide range of capabilities in extraction through highly specific micro-cavity binding sites.[13] Due to the specific binding site created in a MIP this technique is showing promise in analytical chemistry as a useful method for solid phase extraction.[14] The capability for MIPs to be a cheaper easier production of antibody/enzyme like binding sites doubles the use of this technique as a valuable breakthrough in medical research and application.[15] Such possible medical applications include "controlled release drugs, drug monitoring devices, and biological receptor mimetics".[16] Beyond this MIPs show a promising future in the developing knowledge and application in food sciences.[17][18]
"Plastic Antibodies" The binding activity of MIPs can be two magnitudes of activity lower than the use of specific antibodies.[19] This magnitude, though lower than antibodies, are still highly specific binding sites[19] that can be made easily and are relatively cheap. This yields a wide variety of applications for MIPs from efficient extraction to pharmaceutical/medical uses.[14][16] MIP's offer many advantages over protein binding sites. Proteins are difficult and expensive to purify, denature (pH, heat, proteolysis), and are difficult to immobilize for reuse. Synthetic polymers are cheap, easy to synthesize, and allow for elaborate, synthetic side chains to be incorporated. Unique side chains allow for higher affinity, selectivity, and specificity.
Molecularly imprinted polymers have also been used to enrich low abundant phosphopeptides from a cell lysate,[20] outperforming titanium dioxide (TiO2) enrichment- which is a golden standard to enrich phosphopeptides.
History
In a paper published in 1931,[21] Polyakov reported the effects of presence of different solvents (benzene, toluene and xylene) on the silica pore structure during drying a newly prepared silica. When H2SO4 was used as the polymerization initiator (acidifying agent), a positive correlation was found between surface areas, e.g. load capacities, and the molecular weights of the respective solvents. Later on, in 1949 Dickey reported the polymerization of sodium silicate in the presence of four different dyes (namely methyl, ethyl, n-propyl and n-butyl orange). The dyes were subsequently removed, and in rebinding experiments it was found that silica prepared in the presence of any of these "pattern molecules" would bind the pattern molecule in preference to the other three dyes. Shortly after this work had appeared, several research groups pursued the preparation of specific adsorbents using Dickey's method. Some commercial interest was also shown by the fact that Merck patented a nicotine filter,[22] consisting of nicotine imprinted silica, able to adsorb 10.7% more nicotine than non-imprinted silica. The material was intended for use in cigarettes, cigars and pipes filters. Shortly after this work had appeared, molecular imprinting attracted wide interest from the scientific community as reflected in the 4000 original papers published in the field during for the period 1931–2009 (from Scifinder). However, although interest in the technique is new, commonly the molecularly imprinted technique has been shown to be effective when targeting small molecules of molecular weight <1000.[23] Therefore, in following subsection molecularly imprinted polymers are reviewed into two categories, for small and big templates.
Production Limitations
Production of novel MIPs has implicit challenges unique to this field. These challenges arise chiefly from the fact that all substrates are different and thus require different monomer and cross-linker combinations to adequately form imprinted polymers for that substrate. The first, and lesser, challenge arises from choosing those monomers which will yield adequate binding sites complementary to the functional groups of the substrate molecule. For example, it would be unwise to choose completely hydrophobic monomers to be imprinted with a highly hydrophilic substrate. These considerations need to be taken into account before any new MIP is created.
Secondly, and more troublesome, the yield of properly created MIPs is limited by the capacity to effectively wash the substrate from the MIP once the polymer has been formed around it.[24] In creating new MIPs, a compromise must be created between full removal of the original template and damaging of the substrate binding cavity. Such damage is generally caused by strong removal methods and includes collapsing of the cavity, distorting the binding points, incomplete removal of the template and rupture of the cavity.

Template Removal
Most of the developments in MIP production during the last decade have come in the form of new polymerization techniques in an attempt to control the arrangement of monomers and therefore the polymers structure. However, there have been very few advances in the efficient removal of the template from the MIP once it has been polymerized. Due to this neglect, the process of template removal is now the least cost efficient and most time consuming process in MIP production.[25] Furthermore, in order of MIPs to reach their full potential in analytical and biotechnological applications, an efficient removal process must be demonstrated.
There are several different methods of extraction which are currently being used for template removal. These have been grouped into 3 main categories: Solvent extraction, physically assisted extraction, and subcritical or supercritical solvent extraction.
Solvent Extraction
- Soxhlet Extraction This has been a standard extraction method with organic solvents since its creation over a century ago. This technique consists of placing the MIP particles into a cartridge inside the extraction chamber, and the extraction solvent in poured into a flask connected to the extractor chamber. The solvent is then heated and condenses inside the cartridge thereby contacting the MIP particles and extracting the template.[26] The main advantages to this technique are the repeated washing of MIP particles with fresh extracting solvent, favors solubilization because it uses hot solvent, no filtration is required upon completion to collect the MIP particles, the equipment is affordable, and it is very versatile and can be applied to nearly any polymer matrix.[27] The main disadvantages are the long extraction time, the large amount of organic solvent used, the possibility or degradation for temperature sensitive polymers, the static nature of the technique does not facilitate solvent flow through MIP, and the automation is difficult.[27]
- Incubation This involves the immersion of the MIPs into solvents that can induce swelling of the polymer network and simultaneously favor the dissociation of the template from the polymer. Generally this method is carried out under mild conditions and the stability of the polymer is not affected. However, much like the Soxhlet extraction technique, this method also is very time consuming.[28]
Physically-Assisted Extraction
- Ultrasound-assisted extraction (UAE) This method uses Ultrasound which is a cyclic sound pressure with a frequency greater than 20 kHz. This method works through the process known as cavitation which forms small bubbles in liquids and the mechanical erosion of solid particles. This causes a local increase in temperature and pressure which favor solubility, diffusivity, penetration and transport of solvent and template molecules.[29][30]
- Microwave-Assisted Extraction (MAE) This method uses microwaves which directly interact with the molecules causing Ionic conduction and dipole rotation. The use of microwaves for extraction make the extraction of the template occur rapidly, however, one must be careful to avoid excessively high temperatures if the polymers are heat sensitive. This has the best results when the technique is used in concert with strong organic acids, however, this poses another problem because it may cause partial MIP degradation as well.[31] This method does have some benefits in that it significantly reduces the time required to extract the template, decreases the solvent costs, and is considered to be a clean technique.[32]
Mechanical method. The recent study has shown that the microcontact molecular imprinting technology allows mechanical removal of the target (large biomolecules, proteins etc.) from template. This technology combined with the biosensor applications is a promising technology for solution of various biotechnological, environmental and medical issues.[15]
Subcritical or Supercritical Solvent Extraction
- Subcritical Water (PHWE) This method employs the use of water, which is the cheapest and greenest solvent, under high temperatures (100–374 C) and pressures ( 10–60 bar). This method is based upon the high reduction in polarity that liquid water undergoes when heated to high temperatures. This allows water to solubilize a wide variety of polar, ionic and non-polar compounds. The decreased surface tension and viscosity under these conditions also favor diffusivity. Furthermore, the high thermal energy helps break intermolecular forces such as dipole-dipole interactions, vander Waals forces, and hydrogen bonding between the template and the matrix.[33][34][35]
- Supercritical CO2 (SFE)
See also
References
- ↑ http://mipdatabase.com/Database.html
- ↑ Sellergren, Börje (2001). Molecularly Imprinted Polymers: Man-made mimics of antibodies and their applications in analytical chemistry. Amsterdam: Elsevier.
- ↑ Tse Sum Bui, Bernadette (2010). "Molecularly imprinted polymers: synthetic receptors in bioanalysis". Anal Bioanal Chem.
- ↑ "Characteristic and Synthetic Approach of Molecularly Imprinted Polymer" Int. J. Mol. Sci. 2006, 7, 155–178
- ↑ Mosbach, Haupt (2000). "Molecularly Imprinted Polymers and Their Use in Biomimetic Sensors". Chem Rev.
- ↑ Sellergren, Börje; Buechel, Gunter (1999). "A porous, molecularly imprinted polymer and preparation". PCT Int. Appl.
- ↑ Nematollahzadeh, Ali; Sun, Wei; Aureliano, Carla S. A.; Lütkemeyer, Dirk; Stute, Jörg; Abdekhodaie, Mohammad J.; Shojaei, Akbar; Sellergren, Börje (2011). "High capacity hierarchically imprinted polymer beads for protein recognition and capture". Angewandte Chemie International Edition. 50 (2): 495–498. PMID 21140388. doi:10.1002/anie.201004774.
- ↑ MIP Technologies, a producer of custom designed molecularly imprinted polymer resins
- ↑ POLYINTELL, an expert in sample clean-up by Solid Phase Extraction
- ↑ list of AFFINIMIPSPE Molecularly Imprinted Polymers based SPE products
- ↑ Delaney, T. L.; Zimin, D.; Rahm, M.; Weiss, D.; Wolfbeis, O. S.; Mirsky, V. M. (2007). "Capacitive detection in ultrathin chemosensors prepared by molecularly imprinted grafting photopolymerization". Analyt. Chemistry. 79: 3220–3225. doi:10.1021/ac062143v.
- ↑ Lok, CM; Son, R. (2009). "Application of molecularly imprinted polymers in food sample analysis – a perspective" (PDF). International Food Research Journal. 16: 127–140.
- ↑ G. Wulff, A. Sarhan, Angew. Chem. 1972,84,364; Angew. Chem. Int. Ed. Engl. 1972, / I, 341; G. Wulff, A. Sarhan, K. Zabrocki, Tetrahedron Lett. 1973, 44, 4329–4332; G. Wulff, A. Sarhan, DE-A 2242796,1974 Cisem. Abstr. 197'583, P 60300~1.
- 1 2 Olsen, Jørgen; Martin, Paul; Wilson, Ian D. (1998). "Molecular imprints as sorbents for solid phase extraction: potential and applications". Anal. Commun. 35: 13H–14H. doi:10.1039/A806379F.
- 1 2 Ertürk, Gizem; et al. (2014). "Microcontact-BSA imprinted capacitive biosensor for real-time, sensitive and selective detection of BSA". Biotechnology Reports. 3: 65–72. doi:10.1016/j.btre.2014.06.006.
- 1 2 Allender, C. J., Richardson, C., Woodhouse, B., Heard, C. M., and Brain, K. R. Int. J. Pharm. 2000 195 39
- ↑ "Food Analyses Using Molecularly Imprinted Polymers". J. Agric. Food Chem. 49 (5): 2105–2114. 2001. doi:10.1021/jf001444h.
- ↑ Sensor Laboratory CNR-IDASC & University of Brescia. Biosensors. http://sensor.ing.unibs.it/?page_id=79 (accessed Feb, 29 2012)
- 1 2 Wulff, G.; Gross, T.; Schönfeld, R. Angew (1997). Chem. Int. Ed. Engl. 36: 1962. Missing or empty
|title=
(help) - ↑ Chen, Jing; Shinde, Sudhirkumar; Koch, Markus-Hermann; Eisenacher, Martin; Galozzi, Sara; Lerari, Thilo; Barkovits, Katalin; Subedi, Prabal; Krüger, Rejko (2015-07-01). "Low-bias phosphopeptide enrichment from scarce samples using plastic antibodies". Scientific Reports. 5: 11438. PMC 4486973
. PMID 26126808. doi:10.1038/srep11438.
- ↑ Polyakov, M.V. (1931). "Adsorption properties and structure of silica gel". Zhurnal fizicheskoi khimii. 2: S. 799–804.
- ↑ Hans, Erlenmeyer (1965). "Silica gel filter for removing nicotine from tobacco smoke". Patent. DE 1965-M64131.
- ↑ Turner, Nicholas W.; Christopher W. Jeans; Keith R. Brain; Christopher J. Allender; Vladimir Hlady; David W. Britt (2006). "From 3D to 2D: A Review of the Molecular Imprinting of Proteins". Biotechnology Progress. 22 (6): 1474–89. PMC 2666979
. PMID 17137293. doi:10.1021/bp060122g.
- ↑ Lorenzo, Rosa A.; Antonia M. Carro; Carmen Alvarez-Lorenzo; Angel Concheiro. Britt (2011). "To Remove or Not to Remove? The Challenge of Extracting the Template to Make the Cavities Available in Molecularly Imprinted Polymers (MIPs)". Int. J. Mol. Sci. 12: 4327–4347. doi:10.3390/ijms12074327.
- ↑ Ellwanger, A.; S. Bayoudh; C. Crecenzi; L. Karlsson; P.K. Owens; K. Ensing; P. Cormack; D. Sherrington; B. Sellergren (2001). "Evaluation of methods aimed at complete removal of template from molecularly imprinted polymers". Analyst. 126: 784–792. doi:10.1039/b009693h.
- ↑ Soxhlet, F. "Die gewichtsanalytische Bestimmung des Milchfettes". Polytechnisches J. (Dingler's) 1879, 232, 461.
- 1 2 Luque de Castro, M.D.; Priego-Capote, F. "Soxhlet extraction: Past and present panacea." J. Chromatogr. A 2010, 1217, 2383–2389.
- ↑ Hillberg, A.L.; Brain, K.R.; Allender, C.J. (2009). "Design and evaluation of thin and flexible theophylline imprinted polymer membrane materials". J. Mol. Recognit. 22: 223–231. doi:10.1002/jmr.935.
- ↑ Cintas, P.; Luche, J.L. (1999). "Green chemistry. The sonochemical approach". Green Chem. 1: 115–125. doi:10.1039/a900593e.
- ↑ Luque-Garcia, J.L.; de Castro, Luque (2003). "Ultrasound: A powerful tool for leaching". Trends Anal. Chem. 22: 90–99.
- ↑ Ellwanger, A.; Berggren, C.; Bayoudh, S.; Crecenzi, C.; Karlsson, L.; Owens, P.K.; Ensing, K.; Cormack, P.; Sherrington, D.; Sellergren, B. (2001). "Evaluation of methods aimed at complete removal of template from molecularly imprinted polymers". Analyst. 126: 784–792. doi:10.1039/b009693h.
- ↑ Tobiszewski, M.; Mechlińska, A.; Zygmunt, B.; Namieśnik, J. (2009). "Green analytical chemistry in sample preparation for determination of trace organic pollutants". Trends Anal. Chem. 28: 943–951. doi:10.1016/j.trac.2009.06.001.
- ↑ Mendiola, J.A.; Herrero, M.; Cifuentes, A.; Ibañez, E. (2007). "Use of compressed fluids for sample preparation: Food applications". J. Chromatogr. A. 1152: 234–246. doi:10.1016/j.chroma.2007.02.046.
- ↑ Teo, C.C.; Tan, S.N.; Hong Yong, J.W.; Hew, C.S.; Ong, E.S. (2010). "Pressurized hot water extraction (PHWE)". J. Chromatogr. A. 1217: 2484–2494. doi:10.1016/j.chroma.2009.12.050.
- ↑ Ong, E.S.; Cheong, J.S.H.; Goh, D. (2006). "Pressurized hot water extraction of bioactive or marker compounds in botanicals and medicinal plant materials". J. Chromatogr. A. 1112: 92–102. doi:10.1016/j.chroma.2005.12.052.