Mouse models of breast cancer metastasis
Breast cancer metastatic mouse models are experimental approaches in which mice are genetically manipulated to develop a mammary tumor leading to distant focal lesions of mammary epithelium . Recent ameliorations in maneuvering the mouse genome have provided the technology to induce mammary cancers in mice arising from genetic mutations that have been identified in human cancer. This means models can be generated based upon molecular lesions consistent with the human disease.
Breast cancer metastasis
Metastasis is a process of migration of tumour cells from the primary cancer site to a distant location where the cancer cells form secondary tumors. Metastatic breast cancer represents the most devastating attribute of cancer and it is considered an advanced-stage event.[1] Human breast cancer metastasizes to multiple distant organs such as the brain, lungs, bones and liver.
Genetic diversity between primary and metastatic tumor
The classical theory developed in the early 70’s anticipated that metastasis is due to genetically determined subpopulations in primary tumours.[2] The genetic variance between metastatic foci is significant for only particular locus and within specific cell populations or only one-cell population shows differences and some loci are divergent only in one cell subpopulation. This explains the concept of tumour heterogeneity and the order of genetic events during tumor evolution. Many of the genes driving the growth at primary site can determine the dissemination and colonization at the ectopic site.[3][4][5] Breast cancer is consensually considered genetically and clinically as a heterogeneous disease, in that it reflects the heterogeneity of the normal breast tissue at its origin17873350.[6] A number of discrete genetic events have to occur in order to enable individual tumor cells that have the capacity to grow at an ectopic site. The metastatic progression depends on the regulation of developmental programs and environmental events.[7] The metastatic potential of sub populations within mouse mammary cells is now considered as relatively an early event and dissemination occurs at the same time of pre invasive or micro-invasive lesions.[8][9] The genetic profiles of primary and metastatic lesions in breast carcinomas show a large extent of clonal pertinence between lesions.[10][11] There are various patterns of prevalence of genetic mutations in the genomes of primary breast tumour and its metastases.[12][13][14] It also confirms the genetic heterogeneity between the primary neoplasm of breast cancer patients and their respective metastases.[15][16]
Genes involved in organ specific metastasis
Breast cancer phenotypes periodically express genes in metastasis that are indispensable for the metastatic process. Metastatic diversity is mediated by the activation of genes that act as coupling to organ-specific growth.[17] The growth of lesions at the ectopic site depends on multiple complex interactions between metastatic cells and host homeostatic mechanisms. Lethal protein-protein interactions at the metastatic site aid the survival of adapted cells.[18]
Generating mouse models of breast cancer
Targeted expression of oncogenes in mouse mammary epithelial cells is a way of modeling human breast cancer. Mutation or over expression of oncogenes can be kept under controlled expression in a very specific cellular context rather than throughout the organism.
Mice in genetic research
- In 1909, Clarence C. Little developed the first inbred strain, the DBA (Dilute, brown non-Agouti) mouse.
- In 1915, N.M Haldane identified first linkage in mouse between Albino mice and pink eye dilution on chromosome seven.
- In 1921, C57BL became one of the most widely used mice in genetics and was the first strain to have its genome sequenced.
- In 1982, Palmiter and Brinster implanted a foreign gene into fertilized egg, finally generating the first transgenic mice genetically engineered to express dominant oncogenes.[19]
- In 1982, the stimulation of expression from the MMTV-LTR (Mouse mammary tumor virus-Long terminal repeat) was done by multiple rounds of pregnancy and lactation to evaluate the relevance of a cellular proto-oncogene, c-myc.[20]
Human and mouse: a genomic comparison
Genetic studies of common diseases in humans suffer significant limitations for practical and ethical reasons. Human cell lines can be used to model disease but it is difficult to study processes at the tissue level, within an organ or across the entire body. Mice can be a good representation of diseases in humans because:.[21]
- There are close similarities of physiology, development and cell biology between mice and humans.
- Humans and mice both have around 30,000 protein-coding genes. The number of mouse genes without a corresponding human homologue is less than 1%.
- 90% of the human and mouse genomes are syntenic.
- 40% of both human and mouse genomes can be aligned at the nucleotide level.
- Mice have relatively short gestation periods.
- Mice take a brief time to reach sexual maturity.
- Mice have large litter sizes.
- The availability of hundreds of mutations affecting almost every tissue and aspect of development.
Simple tumor transplantation models
Transplantation of tumor cells into immunodeficient mice is a tool to study breast cancer and its metastatic effects. The transplantation occurs as either allotransplants or xenographic transplants.[22] Commonly, human cells are inoculated in an immunocompromised murine recipient. Inoculating cells through intra ductal transplantations,[23] by cleared mammary fat pad injections[24][25] or by transplantations into the tail vein.[26][27][28] Different organs can be seeded with breast cancer cells depending on the route of injection[29]
- Cardiac injection: Bone
- Tail vein injection: Lung
- Splenic injection: Liver
- Carotid artery Injection: Brain
Genetically engineered mice to study metastasis
Genetically engineered mice are constructed to model human phenotypes and pathologies. Mutant mice may include transgenes using different delivery methods:
- The use of bacteria-derived tetracycline-inducible system permitting the switching on or off (Tet-On/Tet-Off system)[30]
- Targeted mutations by knock in gene and knock out sequence by using Cre-Lox recombination system[31]
- Introduction of retro viral mutations[32]
- Introduction of chemically induced mutations
Transgenic mouse models of breast cancer
The mice undergoing the process of transgenesis are known as transgenic mice. A basic transgene has a promoter region, Protein coding sequence, Intron and a stop codon. Mouse mammary tumor virus (MMTV), is a retro virus that has been a known promoter to cause breast tumors once activated.[33] MMTV is a heritable somatic mutagen whose target range is limited. It harbors a regulatory DNA sequence called the long terminal repeat (LTR), which promotes steroid-hormone-inducible transcription.[34][35] Whey acidic protein (WAP),[36] is another common promoter used to generate mouse mammary cancer models. For a list of other mammary gland specific promoters and mouse models see.[37]

MMTV-PyMT
MMTV-PyMT is the model of breast cancer metastasis, in which MMTV-LTR is used to drive the expression of mammary gland specific polyomavirus middle T-antigen, leading to a rapid development of highly metastatic tumors.[38] MMTV-PyMT is the most commonly used model for the study of mammary tumor progression and metastasis. MMTV-PyMT mice are then crossed bred with other genetically modified mice to generate various types of breast cancer models, including:
- PI3K/Akt signalling in metastasis can be demonstrated in MMTV-PyMT; Akt1−/− mice.[39]
- Chemoattractive paracrine loop of colony-stimulating factor-1 (CSF-1) and EGF ligands between tumor-associated macrophages (TAMs) and tumor cells, and the lung metastasis can be studied by crossing MMTV-PyMT mice with Csf-1−/− mice.[40]
- The role of an innate and adaptive immune response to assist metastasis can be studied in MMTV-PyMT; Rag1−/− mice in which CD4+ T cells are selectively lost. Interleukin-4 (IL4) lacking model of MMTV-PyMT; IL4−/− mice.[41]
- Role of the adhesion molecule CD44 in lung metastasis.[42]
- Conditional ablation in MMTV-PyMT breast cancer cells has been done to reveal pro-metastatic actions of the angiogenic factors, Vascular endothelial growth factor A (VEGF-A).[43]
- The role of autocrine transforming growth factor beta 1(TGF-β1) signaling on motility and survival in PyVmT cells derived from an MMTV-PyVmT mouse mammary cancer.[44]
- Others are MMTV-PyMT; uPA-/-[45] and MMTV-PyMT; MEKK1-/-.[46]
MMTV-HER2/neu
The MMTV-LTR can also be used to promote receptor tyrosine-protein kinase ErbB2 to transform the mouse mammary epithelium. ErbB2 is an oncogene amplified and overexpressed in around 20% of human breast cancers. The mice harbouring this oncogene develop multifocal adenocarcinomas with lung metastases at about 15 weeks after pregnancy.[47][48]
Bi-transgenic models
Mouse models having two transgenes are called bi transgenic.To check the cooperation of two oncogenes, Tim Stewert and group made the first bi-transgenic mouse models in 1987, MMTV-Myc and MMTV- Ras mice were crossed with a resulting acceleration in tumorigenesis.[49] Expression of TGFβ in the breast cancer cells of MMTV-ErbB2; MMTV-TGFβ double-transgenic mice can induce higher levels of circulating tumor cells and lung metastasis.[50] Ras gene can be combined with rtTA (reverse tetracycline transactivator) to generate bi-transgenic inducible mouse model through tetracycline-controlled transcriptional activation e.g. mice carrying TetO-KrasG12D (TOR) and MMTV-rtTA (MTB), comes with the transgene expressing the reverse tetracycline transactivator (rtTA) in mammary epithelial cells.[51]
Tri-transgenic models
Tri-transgenic mouse models constitute of more than two genes. Multiple combinations and genetic modifications are made in such a way that either one or all the genes are put into a continuously expressed status, or in a controlled fashion to activate them at different time points. For example, TOM( TetO-myc); TOR; MTB mice, where both the myc (M) and ras (R) genes are under the control of tetracycline operators. They can also both be activated or deactivated by adding doxycycline. Other combinations in this respect are TOM; Kras; MTB, where myc can be induced and uninduced at various time points while Kras is in continuous expressed state, and myc; TOR; MTB model is vice versa.[52]
Applications of genetically modified mice to study metastasis
Metastatic cascade can be studied by keeping the gene activation under control or by adding a reporter gene e.g. Beta actin GFP (Green fluorescent protein) or RFP (Red fluorescent protein).
Identification of genes that regulate metastasis
By knocking in/knocking out specific genes by homologous recombination, the extent of metastasis can be measured and new target genes identification can be achieved e.g. a gene that consistently regulates metastatic behavior of cancer cells is TGF-β1. Acute ablation of TGF-β signaling in MMTV-PyMT mammary tumor cells leads to a five-fold increase in lung metastasis.[53]
Lineage tracing in metastasis models
The quantitative lineage-tracing strategies have proven to be successful in resolving cell fates in normal epithelial tissues either using tissue –specific or stem-cell-specific transgenes. To conduct an inducible lineage-tracing experiment two components must be engineered into the mouse genome: a switch and a reporter. The switch is commonly a drug-regulated form of the bacterial enzyme Cre-recombinase. This enzyme recognizes specific sequences, called LoxP sites.[54] Proteins that are capable of enhancing the identification of labeled cells or a specific population in unlabelled cells are encoded by the reporter transgenes. After harvesting all the ten mouse mammary glands from the transgenic mice, single cell suspension is usually made and transplanted either in tail vein of non transgenic recipient mice[26] or in cleared fat pad of non-transgenic mice repopulating the mammary fat pad.[55] These cells are then followed in the blood stream, lungs, bone marrow and liver to look for the favorable site of metastasis.these transgenic cells can be traced according to their special features of either fluorescence or induced by placing the recipients on doxycycline food.
Circulating tumor cells
Another tool to study breast cancer metastasis is to look for circulating tumor cells in transgenic mice e.g. MMTV-PyMT mice can respond to various therapies in shedding tumor cells in the blood leading to lung metastasis.[56] Not only in blood but cells can be detected in bone marrow e.g. cytokeratin-positive cells in the bone marrow of MMTV-pyMT and MMTV-Neu transgenic mice were identified but not in the wild type controls.[57]
Limitations
In the absence of specific markers for mammary cells, models with genetic marking of tumor cells gives the best experimental advantage, however the low volume of peripheral blood that can be obtained from live animals limits the application of this technique.
In vivo imaging of metastatic mouse models
Transgenic mouse models can be imaged by various non-invasive techniques.
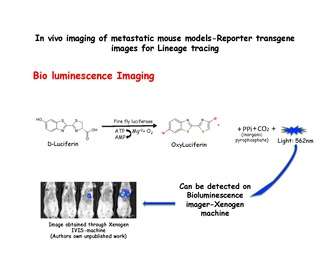
Bioluminescence imaging
Bioluminescence imaging relies on the detection of light produced by the enzymatic oxidation of an exogenous substrate. The substrate luciferin, is oxidized to oxyluciferin in the presence of luciferase and emits light, which can be detected using an IVIS system such as a Xenogen machine. Dissociated mammary cells from MMTV-PyMT: IRES: Luc; MTB (Internal ribosome entry site: Luciferin) animals (which were not exposed to doxycycline) can be injected into the lateral tail veins of immunodeficient mice on a doxycycline-free diet. No bioluminescence signal will be observed in the lungs of recipient mice until they are given doxycycline food. Bioluminescence can then be detected in the chest within 2 weeks of the start of doxycycline exposure.[26] Luciferase is injected just before taking the images.
Fluorescent imaging
Intravital microscopy with multi photon excitation is a technique to visualize genetically engineered cells directly in vivo. Multi step metastatic cascades can be visualized by labelling with unique fluorescent colour under fluorescence microscope.[58][59]
Radioisotopic imaging
Positron emission tomography (PET), single photon emission computed tomography (SPECT) and computed tomography (CT) have been used to compare the efficiency of these in vivo imaging for detecting lesions at an early stage and to evaluate the response to chemotherapy.[60]
See also
- Ensembl genome database of model organisms
- Fate mapping
- Firefly luciferin
- Gene targeting
- Gene trapping
- Genetic recombination
- History of model organisms
- Homologous recombination
- Recombinase-mediated cassette exchange
- Site-specific recombinase technology
References
- ↑ Hanahan, D.; Weinberg, R. (2000). "The hallmarks of cancer". Cell. 100 (1): 57–70. PMID 10647931. doi:10.1016/S0092-8674(00)81683-9.
- ↑ Fidler, I. J. (1973). "Selection of successive tumour lines for metastasis". Nature: New biology. 242 (118): 148–9. PMID 4512654. doi:10.1038/newbio242148a0.
- ↑ Martins, F. C.; De, S; Almendro, V; Gönen, M; Park, S. Y.; Blum, J. L.; Herlihy, W; Ethington, G; Schnitt, S. J.; Tung, N; Garber, J. E.; Fetten, K; Michor, F; Polyak, K (2012). "Evolutionary pathways in BRCA1-associated breast tumors". Cancer Discovery. 2 (6): 503–11. PMC 3738298
. PMID 22628410. doi:10.1158/2159-8290.CD-11-0325.
- ↑ Shah, S. P.; Morin, R. D.; Khattra, J; Prentice, L; Pugh, T; Burleigh, A; Delaney, A; Gelmon, K; Guliany, R; Senz, J; Steidl, C; Holt, R. A.; Jones, S; Sun, M; Leung, G; Moore, R; Severson, T; Taylor, G. A.; Teschendorff, A. E.; Tse, K; Turashvili, G; Varhol, R; Warren, R. L.; Watson, P; Zhao, Y; Caldas, C; Huntsman, D; Hirst, M; Marra, M. A.; Aparicio, S (2009). "Mutational evolution in a lobular breast tumour profiled at single nucleotide resolution". Nature. 461 (7265): 809–13. PMID 19812674. doi:10.1038/nature08489.
- ↑ Geyer, F. C.; Weigelt, B; Natrajan, R; Lambros, M. B.; De Biase, D; Vatcheva, R; Savage, K; MacKay, A; Ashworth, A; Reis-Filho, J. S. (2010). "Molecular analysis reveals a genetic basis for the phenotypic diversity of metaplastic breast carcinomas". The Journal of Pathology. 220 (5): 562–73. PMID 20099298. doi:10.1002/path.2675.
- ↑ Ashkenazi, R; Jackson, T. L.; Dontu, G; Wicha, M. S. (2007). "Breast cancer stem cells-research opportunities utilizing mathematical modeling". Stem cell reviews. 3 (2): 176–82. PMID 17873350. doi:10.1007/s12015-007-0026-2.
- ↑ Müller, A; Homey, B; Soto, H; Ge, N; Catron, D; Buchanan, M. E.; McClanahan, T; Murphy, E; Yuan, W; Wagner, S. N.; Barrera, J. L.; Mohar, A; Verástegui, E; Zlotnik, A (2001). "Involvement of chemokine receptors in breast cancer metastasis". Nature. 410 (6824): 50–6. PMID 11242036. doi:10.1038/35065016.
- ↑ Klein, C. A. (2009). "Parallel progression of primary tumours and metastases". Nature Reviews Cancer. 9 (4): 302–12. PMID 19308069. doi:10.1038/nrc2627.
- ↑ Weng, D; Penzner, J. H.; Song, B; Koido, S; Calderwood, S. K.; Gong, J (2012). "Metastasis is an early event in mouse mammary carcinomas and is associated with cells bearing stem cell markers". Breast Cancer Research. 14 (1): R18. PMC 3496135
. PMID 22277639. doi:10.1186/bcr3102.
- ↑ Liu, W; Laitinen, S; Khan, S; Vihinen, M; Kowalski, J; Yu, G; Chen, L; Ewing, C. M.; Eisenberger, M. A.; Carducci, M. A.; Nelson, W. G.; Yegnasubramanian, S; Luo, J; Wang, Y; Xu, J; Isaacs, W. B.; Visakorpi, T; Bova, G. S. (2009). "Copy number analysis indicates monoclonal origin of lethal metastatic prostate cancer". Nature Medicine. 15 (5): 559–65. PMC 2839160
. PMID 19363497. doi:10.1038/nm.1944.
- ↑ Torres, L; Ribeiro, F. R.; Pandis, N; Andersen, J. A.; Heim, S; Teixeira, M. R. (2007). "Intratumor genomic heterogeneity in breast cancer with clonal divergence between primary carcinomas and lymph node metastases". Breast Cancer Research and Treatment. 102 (2): 143–55. PMID 16906480. doi:10.1007/s10549-006-9317-6.
- ↑ Pandis, N; Teixeira, M. R.; Adeyinka, A; Rizou, H; Bardi, G; Mertens, F; Andersen, J. A.; Bondeson, L; Sfikas, K; Qvist, H; Apostolikas, N; Mitelman, F; Heim, S (1998). "Cytogenetic comparison of primary tumors and lymph node metastases in breast cancer patients". Genes, Chromosomes and Cancer. 22 (2): 122–9. PMID 9598799. doi:10.1002/(SICI)1098-2264(199806)22:2<122::AID-GCC6>3.0.CO;2-Z.
- ↑ Kuukasjärvi, T; Karhu, R; Tanner, M; Kähkönen, M; Schäffer, A; Nupponen, N; Pennanen, S; Kallioniemi, A; Kallioniemi, O. P.; Isola, J (1997). "Genetic heterogeneity and clonal evolution underlying development of asynchronous metastasis in human breast cancer". Cancer Research. 57 (8): 1597–604. PMID 9108466.
- ↑ Bonsing, B. A.; Corver, W. E.; Fleuren, G. J.; Cleton-Jansen, A. M.; Devilee, P; Cornelisse, C. J. (2000). "Allelotype analysis of flow-sorted breast cancer cells demonstrates genetically related diploid and aneuploid subpopulations in primary tumors and lymph node metastases". Genes, Chromosomes and Cancer. 28 (2): 173–83. PMID 10825002. doi:10.1002/(SICI)1098-2264(200006)28:2<173::AID-GCC6>3.0.CO;2-1.
- ↑ Wu, J. M.; Fackler, M. J.; Halushka, M. K.; Molavi, D. W.; Taylor, M. E.; Teo, W. W.; Griffin, C; Fetting, J; Davidson, N. E.; De Marzo, A. M.; Hicks, J. L.; Chitale, D; Ladanyi, M; Sukumar, S; Argani, P (2008). "Heterogeneity of breast cancer metastases: Comparison of therapeutic target expression and promoter methylation between primary tumors and their multifocal metastases". Clinical Cancer Research. 14 (7): 1938–46. PMC 2965068
. PMID 18381931. doi:10.1158/1078-0432.CCR-07-4082.
- ↑ Schmidt-Kittler, O; Ragg, T; Daskalakis, A; Granzow, M; Ahr, A; Blankenstein, T. J.; Kaufmann, M; Diebold, J; Arnholdt, H; Muller, P; Bischoff, J; Harich, D; Schlimok, G; Riethmuller, G; Eils, R; Klein, C. A. (2003). "From latent disseminated cells to overt metastasis: Genetic analysis of systemic breast cancer progression". Proceedings of the National Academy of Sciences. 100 (13): 7737–42. PMC 164657
. PMID 12808139. doi:10.1073/pnas.1331931100.
- ↑ Martín, B; Sanz, R; Aragüés, R; Oliva, B; Sierra, A (2008). "Functional clustering of metastasis proteins describes plastic adaptation resources of breast-cancer cells to new microenvironments". Journal of Proteome Research. 7 (8): 3242–53. PMID 18582095. doi:10.1021/pr800137w.
- ↑ Langley, R. R.; Fidler, I. J. (2007). "Tumor cell-organ microenvironment interactions in the pathogenesis of cancer metastasis". Endocrine Reviews. 28 (3): 297–321. PMID 17409287. doi:10.1210/er.2006-0027.
- ↑ Palmiter, R. D.; Brinster, R. L.; Hammer, R. E.; Trumbauer, M. E.; Rosenfeld, M. G.; Birnberg, N. C.; Evans, R. M. (1992). "Dramatic growth of mice that develop from eggs microinjected with metallothionein-growth hormone fusion genes. 1982". Biotechnology (Reading, Mass.). 24: 429–33. PMID 1422050.
- ↑ Nusse, R; Varmus, H. E. (1982). "Many tumors induced by the mouse mammary tumor virus contain a provirus integrated in the same region of the host genome". Cell. 31 (1): 99–109. PMID 6297757. doi:10.1016/0092-8674(82)90409-3.
- ↑ Chinwalla, A. T.; Waterston, L. L.; Lindblad-Toh, K. D.; Birney, G. A.; Rogers, L. A.; Abril, R. S.; Agarwal, T. A.; Agarwala, L. W.; Ainscough, E. R.; Alexandersson, J. D.; An, T. L.; Antonarakis, W. E.; Attwood, J. O.; Baertsch, M. N.; Bailey, K. H.; Barlow, C. S.; Beck, T. C.; Berry, B.; Birren, J.; Bloom, E.; Bork, R. H.; Botcherby, M. C.; Bray, R. K.; Brent, S. P.; Brown, P.; Brown, E.; Bult, B.; Burton, T.; Butler, D. G.; Campbell, J. (2002). "Initial sequencing and comparative analysis of the mouse genome". Nature. 420 (6915): 520–562. PMID 12466850. doi:10.1038/nature01262.
- ↑ Khanna, C; Hunter, K (2005). "Modeling metastasis in vivo". Carcinogenesis. 26 (3): 513–23. PMID 15358632. doi:10.1093/carcin/bgh261.
- ↑ Behbod, F; Kittrell, F. S.; Lamarca, H; Edwards, D; Kerbawy, S; Heestand, J. C.; Young, E; Mukhopadhyay, P; Yeh, H. W.; Allred, D. C.; Hu, M; Polyak, K; Rosen, J. M.; Medina, D (2009). "An intraductal human-in-mouse transplantation model mimics the subtypes of ductal carcinoma in situ". Breast Cancer Research. 11 (5): R66. PMC 2790841
. PMID 19735549. doi:10.1186/bcr2358.
- ↑ Aslakson, C. J.; Miller, F. R. (1992). "Selective events in the metastatic process defined by analysis of the sequential dissemination of subpopulations of a mouse mammary tumor". Cancer Research. 52 (6): 1399–405. PMID 1540948.
- ↑ Yang, Jing; Mani, Sendurai A; Donaher, Joana Liu; Ramaswamy, Sridhar; Itzykson, Raphael A; Come, Christophe; Savagner, Pierre; Gitelman, Inna; Richardson, Andrea; Weinberg, Robert A (2004). "Twist, a Master Regulator of Morphogenesis, Plays an Essential Role in Tumor Metastasis". Cell. 117 (7): 927–39. PMID 15210113. doi:10.1016/j.cell.2004.06.006.
- 1 2 3 Podsypanina, K; Du, Y. C.; Jechlinger, M; Beverly, L. J.; Hambardzumyan, D; Varmus, H (2008). "Seeding and propagation of untransformed mouse mammary cells in the lung". Science. 321 (5897): 1841–4. PMC 2694414
. PMID 18755941. doi:10.1126/science.1161621.
- ↑ Talmadge, J. E.; Singh, R. K.; Fidler, I. J.; Raz, A (2007). "Murine models to evaluate novel and conventional therapeutic strategies for cancer". The American Journal of Pathology. 170 (3): 793–804. PMC 1864878
. PMID 17322365. doi:10.2353/ajpath.2007.060929.
- ↑ Kim, M. Y.; Oskarsson, T; Acharyya, S; Nguyen, D. X.; Zhang, X. H.; Norton, L; Massagué, J (2009). "Tumor self-seeding by circulating cancer cells". Cell. 139 (7): 1315–26. PMC 2810531
. PMID 20064377. doi:10.1016/j.cell.2009.11.025.
- ↑ Vargo-Gogola, T; Rosen, J. M. (2007). "Modelling breast cancer: One size does not fit all". Nature Reviews Cancer. 7 (9): 659–72. PMID 17721431. doi:10.1038/nrc2193.
- ↑ Gossen, M; Bujard, H (1992). "Tight control of gene expression in mammalian cells by tetracycline-responsive promoters". Proceedings of the National Academy of Sciences of the United States of America. 89 (12): 5547–51. PMC 49329
. PMID 1319065. doi:10.1073/pnas.89.12.5547.
- ↑ Sauer, B; Henderson, N (1989). "Cre-stimulated recombination at loxP-containing DNA sequences placed into the mammalian genome". Nucleic Acids Research. 17 (1): 147–61. PMC 331541
. PMID 2783482. doi:10.1093/nar/17.1.147.
- ↑ Du, Z; Podsypanina, K; Huang, S; McGrath, A; Toneff, M. J.; Bogoslovskaia, E; Zhang, X; Moraes, R. C.; Fluck, M; Allred, D. C.; Lewis, M. T.; Varmus, H. E.; Li, Y (2006). "Introduction of oncogenes into mammary glands in vivo with an avian retroviral vector initiates and promotes carcinogenesis in mouse models". Proceedings of the National Academy of Sciences. 103 (46): 17396–401. PMC 1635021
. PMID 17090666. doi:10.1073/pnas.0608607103.
- ↑ Callahan, R; Smith, G. H. (2000). "MMTV-induced mammary tumorigenesis: Gene discovery, progression to malignancy and cellular pathways". Oncogene. 19 (8): 992–1001. PMID 10713682. doi:10.1038/sj.onc.1203276.
- ↑ Ringold, G. M.; Yamamoto, K. R.; Tomkins, G. M.; Bishop, M; Varmus, H. E. (1975). "Dexamethasone-mediated induction of mouse mammary tumor virus RNA: A system for studying glucocorticoid action". Cell. 6 (3): 299–305. PMID 212202. doi:10.1016/0092-8674(75)90181-6.
- ↑ Yamamoto, K. R.; Payvar, F; Firestone, G. L.; Maler, B. A.; Wrange, O; Carlstedt-Duke, J; Gustafsson, J. A.; Chandler, V. L. (1983). "Biological activity of cloned mammary tumor virus DNA fragments that bind purified glucocorticoid receptor protein in vitro". Cold Spring Harbor symposia on quantitative biology. 47 (2): 977–84. PMID 6305596. doi:10.1101/sqb.1983.047.01.111.
- ↑ Campbell, S. M.; Rosen, J. M.; Hennighausen, L. G.; Strech-Jurk, U; Sippel, A. E. (1984). "Comparison of the whey acidic protein genes of the rat and mouse". Nucleic Acids Research. 12 (22): 8685–97. PMC 320407
. PMID 6095207. doi:10.1093/nar/12.22.8685.
- ↑ Fantozzi, A; Christofori, G (2006). "Mouse models of breast cancer metastasis". Breast Cancer Research. 8 (4): 212. PMC 1779475
. PMID 16887003. doi:10.1186/bcr1530.
- ↑ Guy, C. T.; Cardiff, R. D.; Muller, W. J. (1992). "Induction of mammary tumors by expression of polyomavirus middle T oncogene: A transgenic mouse model for metastatic disease". Molecular and Cellular Biology. 12 (3): 954–61. PMC 369527
. PMID 1312220. doi:10.1128/mcb.12.3.954.
- ↑ Klarenbeek, S; Van Miltenburg, M. H.; Jonkers, J (2013). "Genetically engineered mouse models of PI3K signaling in breast cancer". Molecular Oncology. 7 (2): 146–64. PMID 23478237. doi:10.1016/j.molonc.2013.02.003.
- ↑ Lin, E. Y.; Nguyen, A. V.; Russell, R. G.; Pollard, J. W. (2001). "Colony-stimulating factor 1 promotes progression of mammary tumors to malignancy". The Journal of Experimental Medicine. 193 (6): 727–40. PMC 2193412
. PMID 11257139. doi:10.1084/jem.193.6.727.
- ↑ Denardo, D. G.; Barreto, J. B.; Andreu, P; Vasquez, L; Tawfik, D; Kolhatkar, N; Coussens, L. M. (2009). "CD4(+) T cells regulate pulmonary metastasis of mammary carcinomas by enhancing protumor properties of macrophages". Cancer Cell. 16 (2): 91–102. PMC 2778576
. PMID 19647220. doi:10.1016/j.ccr.2009.06.018.
- ↑ Lopez, J. I.; Camenisch, T. D.; Stevens, M. V.; Sands, B. J.; McDonald, J; Schroeder, J. A. (2005). "CD44 attenuates metastatic invasion during breast cancer progression". Cancer Research. 65 (15): 6755–63. PMID 16061657. doi:10.1158/0008-5472.CAN-05-0863.
- ↑ Schoeffner, D. J.; Matheny, S. L.; Akahane, T; Factor, V; Berry, A; Merlino, G; Thorgeirsson, U. P. (2005). "VEGF contributes to mammary tumor growth in transgenic mice through paracrine and autocrine mechanisms". Laboratory Investigation. 85 (5): 608–23. PMID 15765121. doi:10.1038/labinvest.3700258.
- ↑ Muraoka-Cook, R. S.; Kurokawa, H; Koh, Y; Forbes, J. T.; Roebuck, L. R.; Barcellos-Hoff, M. H.; Moody, S. E.; Chodosh, L. A.; Arteaga, C. L. (2004). "Conditional overexpression of active transforming growth factor beta1 in vivo accelerates metastases of transgenic mammary tumors". Cancer Research. 64 (24): 9002–11. PMID 15604265. doi:10.1158/0008-5472.CAN-04-2111.
- ↑ Almholt, K; Lund, L. R.; Rygaard, J; Nielsen, B. S.; Danø, K; Rømer, J; Johnsen, M (2005). "Reduced metastasis of transgenic mammary cancer in urokinase-deficient mice". International Journal of Cancer. 113 (4): 525–32. PMID 15472905. doi:10.1002/ijc.20631.
- ↑ Cuevas, B. D.; Winter-Vann, A. M.; Johnson, N. L.; Johnson, G. L. (2006). "MEKK1 controls matrix degradation and tumor cell dissemination during metastasis of polyoma middle-T driven mammary cancer". Oncogene. 25 (36): 4998–5010. PMID 16568086. doi:10.1038/sj.onc.1209507.
- ↑ Slamon, D. J.; Clark, G. M.; Wong, S. G.; Levin, W. J.; Ullrich, A; McGuire, W. L. (1987). "Human breast cancer: Correlation of relapse and survival with amplification of the HER-2/neu oncogene". Science. 235 (4785): 177–82. PMID 3798106. doi:10.1126/science.3798106.
- ↑ Muller, W. J.; Sinn, E; Pattengale, P. K.; Wallace, R; Leder, P (1988). "Single-step induction of mammary adenocarcinoma in transgenic mice bearing the activated c-neu oncogene". Cell. 54 (1): 105–15. PMID 2898299. doi:10.1016/0092-8674(88)90184-5.
- ↑ Sinn, E; Muller, W; Pattengale, P; Tepler, I; Wallace, R; Leder, P (1987). "Coexpression of MMTV/v-Ha-ras and MMTV/c-myc genes in transgenic mice: Synergistic action of oncogenes in vivo". Cell. 49 (4): 465–75. PMID 3032456. doi:10.1016/0092-8674(87)90449-1.
- ↑ Siegel, P. M.; Shu, W; Cardiff, R. D.; Muller, W. J.; Massagué, J (2003). "Transforming growth factor beta signaling impairs Neu-induced mammary tumorigenesis while promoting pulmonary metastasis". Proceedings of the National Academy of Sciences. 100 (14): 8430–5. PMC 166246
. PMID 12808151. doi:10.1073/pnas.0932636100.
- ↑ Gunther, E. J.; Belka, G. K.; Wertheim, G. B.; Wang, J; Hartman, J. L.; Boxer, R. B.; Chodosh, L. A. (2002). "A novel doxycycline-inducible system for the transgenic analysis of mammary gland biology". The FASEB Journal. 16 (3): 283–92. PMID 11874978. doi:10.1096/fj.01-0551com.
- ↑ Podsypanina, K; Politi, K; Beverly, L. J.; Varmus, H. E. (2008). "Oncogene cooperation in tumor maintenance and tumor recurrence in mouse mammary tumors induced by Myc and mutant Kras". Proceedings of the National Academy of Sciences. 105 (13): 5242–7. PMC 2278195
. PMID 18356293. doi:10.1073/pnas.0801197105.
- ↑ Bierie, B; Stover, D. G.; Abel, T. W.; Chytil, A; Gorska, A. E.; Aakre, M; Forrester, E; Yang, L; Wagner, K. U.; Moses, H. L. (2008). "Transforming growth factor-beta regulates mammary carcinoma cell survival and interaction with the adjacent microenvironment". Cancer Research. 68 (6): 1809–19. PMID 18339861. doi:10.1158/0008-5472.CAN-07-5597.
- ↑ Srinivas, S; Watanabe, T; Lin, C. S.; William, C. M.; Tanabe, Y; Jessell, T. M.; Costantini, F (2001). "Cre reporter strains produced by targeted insertion of EYFP and ECFP into the ROSA26 locus". BMC Developmental Biology. 1: 4. PMC 31338
. PMID 11299042.
- ↑ Liao, M. J.; Zhang, C. C.; Zhou, B; Zimonjic, D. B.; Mani, S. A.; Kaba, M; Gifford, A; Reinhardt, F; Popescu, N. C.; Guo, W; Eaton, E. N.; Lodish, H. F.; Weinberg, R. A. (2007). "Enrichment of a population of mammary gland cells that form mammospheres and have in vivo repopulating activity". Cancer Research. 67 (17): 8131–8. PMID 17804725. doi:10.1158/0008-5472.CAN-06-4493.
- ↑ Biswas, S; Guix, M; Rinehart, C; Dugger, T. C.; Chytil, A; Moses, H. L.; Freeman, M. L.; Arteaga, C. L. (2007). "Inhibition of TGF-beta with neutralizing antibodies prevents radiation-induced acceleration of metastatic cancer progression". Journal of Clinical Investigation. 117 (5): 1305–13. PMC 1838926
. PMID 17415413. doi:10.1172/JCI30740.
- ↑ Hüsemann, Y; Geigl, J. B.; Schubert, F; Musiani, P; Meyer, M; Burghart, E; Forni, G; Eils, R; Fehm, T; Riethmüller, G; Klein, C. A. (2008). "Systemic spread is an early step in breast cancer". Cancer Cell. 13 (1): 58–68. PMID 18167340. doi:10.1016/j.ccr.2007.12.003.
- ↑ Egeblad, M; Nakasone, E. S.; Werb, Z (2010). "Tumors as organs: Complex tissues that interface with the entire organism". Developmental Cell. 18 (6): 884–901. PMC 2905377
. PMID 20627072. doi:10.1016/j.devcel.2010.05.012.
- ↑ Entenberg, D; Wyckoff, J; Gligorijevic, B; Roussos, E. T.; Verkhusha, V. V.; Pollard, J. W.; Condeelis, J (2011). "Setup and use of a two-laser multiphoton microscope for multichannel intravital fluorescence imaging". Nature Protocols. 6 (10): 1500–20. PMC 4028841
. PMID 21959234. doi:10.1038/nprot.2011.376.
- ↑ Alberini, Jean-Louis; Boisgard, Raphaël; Guillermet, Stéphanie; Siquier, Karine; Jego, Benoît; Thézé, Benoît; Urien, Saik; Rezaï, Keyvan; Menet, Emmanuelle (2016-08-01). "Multimodal In Vivo Imaging of Tumorigenesis and Response to Chemotherapy in a Transgenic Mouse Model of Mammary Cancer". Molecular imaging and biology: MIB: the official publication of the Academy of Molecular Imaging. 18 (4): 617–626. ISSN 1860-2002. PMC 4927598
. PMID 26630973. doi:10.1007/s11307-015-0916-7.
External links
- http://www.zmbh.uni-heidelberg.de/Bujard/default.shtml Hermann Bujard Lab, ZMBH, Heidelberg, Germany
- https://www.youtube.com/watch?v=a4WR6K6gPgw Tet-On Advanced Animation in YouTube
- Jackson Laboratory
- http://www.la-press.com/tetracycline-regulated-systems-in-functional-oncogenomics-article-a200 A detailed overview of Tet-systems in functional oncogenomics
- http://jaxmice.jax.org/news/2014/BreastCancerMetastasis.html Jackson Laboratory