Long-term effects of global warming

- See also Effects of global warming. The focus of this article is on the effects beyond 2100.
There are expected to be various long-term effects of global warming. Most discussion and research, including that by the Intergovernmental Panel on Climate Change (IPCC) reports, concentrates on the effects of global warming up to 2100, with only an outline of the effects beyond this.
Ice loss and sea level rise

Meltwater from melting ice sheets and glacier retreat contributes to a rise in the future sea level.[1]
Antarctica
Concern has been expressed about the stability of the West Antarctic ice sheet (WAIS). In 2002 Vaughan & Spouge argued that the "WAIS most likely will not collapse in the next few centuries."[2] In an inaugural article for members of the National Academy of Sciences elected in 2005, Timothy Lenton and others suggest that a collapse of the West Antarctic ice sheet could occur within a millennium. Specifically, they state "Although the timescale is highly uncertain, a qualitative WAIS change could occur within this millennium, with collapse within 300 years being a worst-case scenario. Rapid sea-level rise (greater than 1 m per century) is more likely to come from the WAIS than from the Greenland ice sheet.".[3] A study in 2015 found that assuming cumulative fossil fuel emissions of 10 000 gigatonnes of carbon, the Antarctic Ice Sheet could melt completely over the following millennia, contributing 58 m to global sea-level rise, and 30 m within the first 1000 years.[4]
Greenland
Greenland's ice sheet contains enough fresh water as ice to raise sea level worldwide by 7 metres (23 ft).[1] Greenland may become warm enough by 2100 to begin an almost complete melt over more than 1,000 years.[5][6] James E. Hansen suggests that inadequate attention is being given to this issue.[7]
One study suggests it would take 3,000 years to completely melt the Greenland ice sheet.[8] This figure was derived from the assumed levels of greenhouse gases over the duration of the experiment.
As the Greenland ice sheet loses mass from calving of icebergs as well as by melting of ice, any such processes tend to accelerate the loss of the ice sheet.[9]
Millennial-timescale events
Some long-term effects happen over thousands, not hundreds, of years.
Disruption to thermohaline circulation
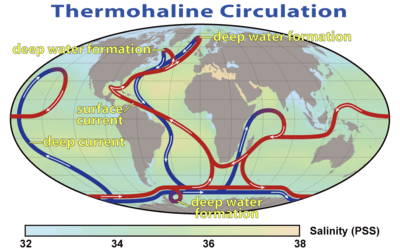
Early work with simplified models suggested that global warming could cause a shutdown of the thermohaline circulation.[10] This effect is not replicated in more sophisticated coupled ocean-atmosphere global climate models, which do not shutdown but do display varying degrees of slowdown.
This may take a considerable time to occur, as Knutti and Stocker found, again from a simplified model, when :"…thermohaline shutdowns can occur thousands of years after the warming has stopped."[11]
Ocean anoxia
One study suggests that the amount of oxygen dissolved in the oceans may decline, with adverse consequences for ocean life.[12] This effect was determined using a model run of 100,000 years. The researchers predicted:
…severe, long-term ocean oxygen depletion, as well as a great expansion of ocean oxygen-minimum zones for scenarios with high emissions or high climate sensitivity. We find that climate feedbacks within the Earth system amplify the strength and duration of global warming, ocean heating and oxygen depletion. Decreased oxygen solubility from surface-layer warming accounts for most of the enhanced oxygen depletion in the upper 500 m of the ocean. Possible weakening of ocean overturning and convection lead to further oxygen depletion, also in the deep ocean.
Other research by the Leibniz Institute for Marine Sciences predicts that minimum oxygen zones, particularly in the pacific are expanding, with an estimated total reduction of ocean oxygen levels from 1% to 7% by the end of the century.[13]
Clathrate decomposition

Source: USGS
Methane clathrate, also called methane hydrate, is a form of water ice that contains a large amount of methane within its crystal structure. Extremely large deposits of methane clathrate have been found under sediments on the ocean floors of Earth (estimated at 3000[14]–11,000[15] Gton C).
MacDonald suggests clathrate volumes are "about 11,000 Gt of carbon for ocean sediments, and about 400 Gt for sediments under permafrost regions".[15] Buffett and Archer predict "eventual releases of 2000–4000 Gton C in response to a ~2000 Gton C anthropogenic carbon release",[16] for which they suggest no timescale.
Archer considered the timescale and determined that "…on longer time scales of 1–10 ky, there may be a positive feedback with ocean temperature, amplifying the long-term climate impact of anthropogenic CO2 release."[17]
Long-term return to equilibrium
After the PETM peak there was an extended period of cooling or "climate whiplash".[18]
While the warm surface waters of the oceans have limited ability to absorb anthropogenic carbon dioxide, the coldest surface waters near the poles (2–3% of ocean surfaces) can transfer significant amounts of carbon dioxide to deep-ocean reserves. Over a period of many centuries, this process and the process of calcium carbonate absorption of carbon dioxide on land and in the oceans will remove 60–80% of the excess carbon dioxide.[19]
Igneous rock when exposed to a near surface environment absorbs carbon dioxide through a very slow weathering rate, but weathering increases in a warmer, higher rainfall climate, speeding the process. This geological weathering will absorb the remaining 20–40% of anthropogenic carbon dioxide over the period of tens of thousands to hundreds of thousands of years.[19]
References
- 1 2 "Climate Change 2001: The Scientific Basis" (Table 11.3). Intergovernmental Panel on Climate Change. 2001-02-16. Retrieved 2007-12-24.
- ↑ Vaughan, D. G.; Spouge, J. R. (2002). "Risk Estimation of Collapse of the West Antarctic Ice Sheet". Climatic Change. 52: 65–00. doi:10.1023/A:1013038920600.
- ↑ Lenton, T. M.; Held, H.; Kriegler, E.; Hall, J. W.; Lucht, W.; Rahmstorf, S.; Schellnhuber, H. J. (2008). "Inaugural Article: Tipping elements in the Earth's climate system". Proceedings of the National Academy of Sciences. 105 (6): 1786. Bibcode:2008PNAS..105.1786L. doi:10.1073/pnas.0705414105.
- ↑ Winkelmann, Ricarda; Levermann, Anders; Ridgwell, Andy; Caldeira, Ken (2015). "Combustion of available fossil fuel resources sufficient to eliminate the Antarctic Ice Sheet". Science Advances. 1: e1500589. doi:10.1126/sciadv.1500589.
- ↑ Gregory JM; Huybrechts P; Raper SC (April 2004). "Climatology: threatened loss of the Greenland ice-sheet" (PDF). Nature. 428 (6983): 616. Bibcode:2004Natur.428..616G. PMID 15071587. doi:10.1038/428616a.
The Greenland ice-sheet would melt faster in a warmer climate and is likely to be eliminated — except for residual glaciers in the mountains — if the annual average temperature in Greenland increases by more than about 3 °C. This would raise the global average sea-level by 7 metres over a period of 1000 years or more. We show here that concentrations of greenhouse gasses will probably have reached levels before the year 2100 that are sufficient to raise the temperature past this warming threshold.
- ↑ "Regional Sea Level Change" (Figure 11.16). Intergovernmental Panel on Climate Change.
- ↑ J E Hansen (April–June 2007). "Scientific reticence and sea level rise". Environ. Res. Lett. 2 (2): 024002. Bibcode:2007ERL.....2b4002H. arXiv:physics/0703220
. doi:10.1088/1748-9326/2/2/024002.
- ↑ Lowe, Jason; Jonathan M. Gregory; Jeff Ridley; Philippe Huybrechts; Robert J. Nicholls; Matthew Collins (January 2006). "The Role of Sea-Level Rise and the Greenland Ice Sheet in Dangerous Climate Change: Implications for the Stabilisation of Climate" (PDF). UK Met Office. Archived from the original (PDF) on March 27, 2009. Retrieved 2009-03-29.
- ↑ Zwally, J.; Abdalati, W.; Herring, T.; Larson, K.; Saba, J.; Steffen, K. (Jul 2002). "Surface melt-induced acceleration of Greenland ice-sheet flow". Science. 297 (5579): 218–222. Bibcode:2002Sci...297..218Z. ISSN 0036-8075. PMID 12052902. doi:10.1126/science.1072708.
- ↑ Schmittner, A.; Stocker, T. F. (1999). "The Stability of the Thermohaline Circulation in Global Warming Experiments". Journal of Climate. 12 (4): 1117–1133. Bibcode:1999JCli...12.1117S. ISSN 1520-0442. doi:10.1175/1520-0442(1999)012<1117:TSOTTC>2.0.CO;2.
- ↑ Knutti, R.; Stocker, T. F. (2002). "Limited Predictability of the Future Thermohaline Circulation Close to an Instability Threshold". Journal of Climate. 15 (2): 179–186. Bibcode:2002JCli...15..179K. ISSN 1520-0442. doi:10.1175/1520-0442(2002)015<0179:LPOTFT>2.0.CO;2.
- ↑ Shaffer, G. .; Olsen, S. M.; Pedersen, J. O. P. (2009). "Long-term ocean oxygen depletion in response to carbon dioxide emissions from fossil fuels". Nature Geoscience. 2 (2): 105–109. Bibcode:2009NatGe...2..105S. doi:10.1038/ngeo420.
- ↑ Ocean Deoxygenation in a Warming World Ralph F. Keeling, Arne Körtzinger, and Nicolas Gruber
- ↑ Buffett, B.; Archer, D. (2004). "Global inventory of methane clathrate: sensitivity to changes in the deep ocean" (PDF). Earth and Planetary Science Letters. 227 (3–4): 185. Bibcode:2004E&PSL.227..185B. doi:10.1016/j.epsl.2004.09.005.
- 1 2 Macdonald, G. J. (1990). "Role of methane clathrates in past and future climates". Climatic Change. 16 (3): 247–281. doi:10.1007/BF00144504.
- ↑ Archer, D.; Buffett, B. (2005). "Time-dependent response of the global ocean clathrate reservoir to climatic and anthropogenic forcing" (PDF). Geochemistry Geophysics Geosystems. 6 (3): Q03002. Bibcode:2005GGG.....603002A. doi:10.1029/2004GC000854.
- ↑ Archer, D.; Martin, P.; Buffett, B.; Brovkin, V.; Rahmstorf, S.; Ganopolski, A. (2004). "The importance of ocean temperature to global biogeochemistry". Earth and Planetary Science Letters. 222 (2): 333–348. Bibcode:2004E&PSL.222..333A. doi:10.1016/j.epsl.2004.03.011.
- ↑ Curt Stager (November 28, 2015). "Tales of a Warmer Planet". The New York Times. Retrieved November 30, 2015.
From the perspective of future generations, the whiplash and subsequent cooling that follows our own thermal peak could be as challenging as the warming.
- 1 2 David Archer (2009). The Long Thaw: How Humans Are Changing the Next 100,000 Years of Earth's Climate. Princeton University Press. p. 109. ISBN 978-0-691-13654-7.