Liquid rocket propellant
The highest specific impulse chemical rockets use liquid propellants (liquid-propellant rockets). They can consist of a single chemical (a monopropellant) or a mix of two chemicals, called bipropellants. Bipropellants can further be divided into two categories; hypergolic propellants, which ignite when the fuel and oxidizer make contact, and non-hypergolic propellants which require an ignition source.
Description
About 170 different propellants made of liquid fuel have been tested, excluding minor changes to a specific propellant such as propellant additives, corrosion inhibitors, or stabilizers. In the U.S. alone at least 25 different propellant combinations have been flown.[1] No completely new propellant has been used for nearly 30 years.[2] Many factors go into choosing a propellant for a liquid propellant rocket engine. The primary factors include ease of operation, cost, hazards/environment and performance. They can consist of a single chemical, a monopropellant, or two, called bipropellants or other mixtures. Bipropellants can be either hypergolic propellant or nonhypergolic. A hypergolic combination of oxidizer and fuel will start to burn upon contact. A nonhypergolic needs an ignition source.[3]
History
Early development, 1926
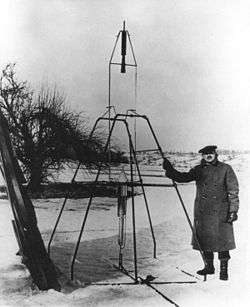
On March 16, 1926, Robert H. Goddard used liquid oxygen (LOX) and gasoline as rocket fuels for his first partially successful liquid-propellant rocket launch. Both propellants are readily available, cheap and highly energetic. Oxygen is a moderate cryogen as air will not liquefy against a liquid oxygen tank, so it is possible to store LOX briefly in a rocket without excessive insulation.
World War II era
Germany had very active rocket development before and during World War II, both for the strategic V-2 rocket and other missiles. The V-2 used an alcohol/LOX liquid propellant engine, with hydrogen peroxide to drive the fuel pumps. The alcohol was mixed with water for engine cooling. Both Germany and the United States developed reusable liquid propellant rocket engines that used a storeable liquid oxidizer with much greater density than LOX and a liquid fuel that would ignite spontaneously on contact with the high density oxidizer. The German engine was powered by hydrogen peroxide and a fuel mixture of hydrazine hydrate and methyl alcohol. The U.S. engine was powered by nitric acid oxidizer and aniline. Both engines were used to power aircraft, the Me-163B Komet interceptor in the case of the German engine and RATO units to assist take-off of aircraft in the case of the U.S. engine.
1950s and 1960s
During the 1950s and 1960s there was a great burst of activity by propellant chemists to find high-energy liquid and solid propellants better suited to the military. Large strategic missiles need to sit in land-based or submarine-based silos for many years, able to launch at a moment's notice. Propellants requiring continuous refrigeration, which cause their rockets to grow ever-thicker blankets of ice, were not practical. As the military was willing to handle and use hazardous materials, a great number of dangerous chemicals were brewed up in large batches, most of which wound up being deemed unsuitable for operational systems. In the case of nitric acid, the acid itself (HNO3) was unstable, and corroded most metals, making it difficult to store. The addition of a modest amount of nitrogen tetroxide, N2O4, turned the mixture red and kept it from changing composition, but left the problem that nitric acid corrodes containers it is placed in, releasing gases that can build up pressure in the process. The breakthrough was the addition of a little hydrogen fluoride (HF), which forms a self-sealing metal fluoride on the interior of tank walls that Inhibited Red Fuming Nitric Acid. This made "IRFNA" storeable. Propellant combinations based on IRFNA or pure N2O4 as oxidizer and kerosene or hypergolic (self igniting) aniline, hydrazine or unsymmetrical dimethylhydrazine (UDMH) as fuel were then adopted in the United States and the Soviet Union for use in strategic and tactical missiles. The self-igniting storeable liquid bi-propellants have somewhat lower specific impulse than LOX/kerosene but have higher density so a greater mass of propellant can be placed in the same sized tanks. Gasoline was replaced by different hydrocarbon fuels, for example RP-1 - a highly refined grade of kerosene. This combination is quite practical for rockets that need not be stored. As of 2017, it is used in the first stages of many orbital launchers.
Kerosene
The V-1 and V-2 rockets developed by Nazi Germany used LOX and ethyl alcohol. One of the main advantages of alcohol was its water content which provided cooling in larger rocket engines. Petroleum-based fuels offered more power than alcohol, but standard gasoline and kerosene left too much silt and combustion by-products that could clog engine plumbing. In addition they lacked the cooling properties of ethyl alcohol.
During the early 1950s, the chemical industry in the US was assigned the task of formulating an improved petroleum-based rocket propellant which would not leave residue behind and also ensure that the engines would remain cool. The result was RP-1, the specifications of which were finalized by 1954. A highly refined form of jet fuel, RP-1 burned much more cleanly than conventional petroleum fuels and also posed less of a danger to ground personnel from explosive vapors. It became the propellant for most of the early American rockets and ballistic missiles such as the Atlas, Titan I, and Thor. The Soviets quickly adopted RP-1 for their R-7 missile, but the majority of Soviet launch vehicles ultimately used storable hypergolic propellants.
Hydrogen
Gaseous hydrogen is commercially produced by the fuel-rich burning of natural gas. Carbon forms a stronger bond with oxygen so the gaseous hydrogen is left behind. Liquid hydrogen is stored and transported without boil-off, because helium, which has a lower boiling point than hydrogen, acts as cooling refrigerant. Only when hydrogen is loaded on a launch vehicle, where no refrigeration exists, it vents to the atmosphere.[4] Many early rocket theorists believed that hydrogen would be a marvelous propellant, since it gives the highest specific impulse. It is also considered the cleanest when used with a liquid oxygen oxidizer because the only by-product is water. Hydrogen in any state is very bulky; for lightweight vehicles it is typically stored as a deeply cryogenic liquid, a technique mastered in the early 1950s as part of the hydrogen bomb development program at Los Alamos. In the late 1950s and early 1960s it was adopted for hydrogen fueled stages such as Centaur and Saturn upper stages. Even as a liquid, hydrogen has low density, requiring large tanks and pumps, and the extreme cold requires tank insulation. This extra weight reduces the mass fraction of the stage or requires extraordinary measures such as pressure stabilization of the tanks to reduce weight. Pressure stabilized tanks support most of the loads with internal pressure rather than with solid structures.
The Soviet rocket program, in part due to a lack of technical capabilities, did not use LH2 as a propellant until the 1980s when it was used for the Energiya core stage.
Comparison to kerosene
Launch pad fires due to spilled kerosene are more damaging than hydrogen fires, primarily for two reasons. First, kerosene burns about 20% hotter in absolute temperature than hydrogen. The second reason is its buoyancy. Since hydrogen is a deep cryogen it boils quickly and rises due to its very low density as a gas. Even when hydrogen burns, the gaseous H2O that is formed has a molecular weight of only 18 u compared to 29.9 u for air, so it rises quickly as well. Kerosene on the other hand falls to the ground and burns for hours when spilled in large quantities, unavoidably causing extensive heat damage that requires time consuming repairs and rebuilding. This is a lesson most frequently experienced by test stand crews involved with firings of large, unproven rocket engines. Hydrogen-fueled engines have special design requirements such as running propellant lines horizontally, so traps do not form in the lines and cause ruptures due to boiling in confined spaces. These considerations apply to all cryogens, such as liquid oxygen and liquid natural gas as well. Use of liquid hydrogen fuel has an excellent safety record and superb performance that is well above that of all other practical chemical rocket propellants.
Lithium and fluorine
The highest specific impulse chemistry ever test-fired in a rocket engine was lithium and fluorine, with hydrogen added to improve the exhaust thermodynamics (all propellants had to be kept in their own tanks, making this a tripropellant). The combination delivered 542 s specific impulse in a vacuum, equivalent to an exhaust velocity of 5320 m/s. The impracticality of this chemistry highlights why exotic propellants are not actually used: to make all three components liquids, the hydrogen must be kept below –252 °C (just 21 K) and the lithium must be kept above 180 °C (453 K). Lithium and fluorine are both extremely corrosive, lithium ignites on contact with air, fluorine ignites on contact with most fuels, including hydrogen. Fluorine and the hydrogen fluoride (HF) in the exhaust are very toxic, which makes working around the launch pad difficult, damages the environment, and makes getting a launch license that much more difficult. Both lithium and fluorine are expensive compared to most rocket propellants. This combination has therefore never flown.
During the 1950s, the Department of Defense initially proposed lithium/fluorine as ballistic missile propellants. A 1954 accident at a chemical works where a cloud of fluorine was released into the atmosphere convinced them to instead use LOX/RP-1.
Methane
In November 2012, SpaceX CEO Elon Musk announced plans to develop liquid methane/LOX rocket engines.[5] It had previously used only RP-1/LOX in the SpaceX rocket engine family. As of March 2014, SpaceX was developing the Raptor methalox bipropellant rocket engine, which by 2016 was predicted to generate 690,000 lbf (3,100 kN) of thrust.[6] The engine was slated to be used on a future super-heavy rocket, the MCT launch vehicle.[7][8]
In July 2014, Firefly Space Systems announced their plans to use methane fuel for their small satellite launch vehicle, Firefly Alpha with an aerospike engine design.[9]
In September 2014, Blue Origin and United Launch Alliance announced the joint development of the BE-4 lox/methane engine. The BE-4 will provide 550,000 lbf of thrust.[10]
Monopropellants
Hydrogen peroxide decomposes to steam and oxygen. Hydrazine decomposes energetically to nitrogen, hydrogen, and ammonia (2N2H4 → N2+H2+2NH3) and is the most widely used in space vehicles. Ammonia decomposition is endothermic and would decrease performance. Nitrous oxide decomposes to nitrogen and oxygen. Steam when externally heated gives a reasonably modest Isp of up to 190 seconds, depending on material corrosion and thermal limits.
Present use
Rocket | Propellants | Isp, vacuum (s) |
---|---|---|
Space shuttle liquid engines |
LOX/LH2 | 453[11] |
Space shuttle solid motors |
APCP | 268[11] |
Space shuttle OMS |
NTO/MMH | 313[11] |
Saturn V stage 1 |
LOX/RP-1 | 304[11] |
Common liquid fuel combinations in current use:
- LOX and kerosene (RP-1). Used for the lower stages of the Soyuz boosters, and the first stage of the U.S. Saturn V, Atlas, and Falcon 9 boosters. Very similar to Robert Goddard's first rocket.
- LOX and liquid hydrogen, used in the stages of the Space Shuttle, Space Launch System, Ariane 5, Delta IV, New Shepard, H-IIB, GSLV and Centaur.
- Nitrogen tetroxide (N2O4) and UDMH or MMH. Used in three first stages of the Russian Proton booster, Indian Vikas engine for PSLV and GSLV rockets, most Chinese boosters, a number of military, orbital and deep space rockets, as this fuel combination is hypergolic and storable for long periods at reasonable temperatures and pressures.
- Hydrazine (N
2H
4) and Aerozine-50 are also used in deep space missions because they are storable and hypergolic, and can be used as a monopropellant with a catalyst.
Upper stage use
The liquid rocket engine propellant combination of liquid oxygen and hydrogen offers the highest specific impulse of currently used conventional rockets. This extra performance largely offsets the disadvantage of low density. Low density of a propellant leads to larger fuel tanks. However, a small increase in specific impulse in an upper stage application can have a significant increase in payload to orbit capability.[2]
Table
Absolute pressure (atm) {psi} | Multiply by |
---|---|
6,895 kPa (68.05) {1000} | 1.00 |
6,205 kPa (61.24) {900} | 0.99 |
5,516 kPa (54.44) {800} | 0.98 |
4,826 kPa (47.63) {700} | 0.97 |
4,137 kPa (40.83) {600} | 0.95 |
3,447 kPa (34.02) {500} | 0.93 |
2,758 kPa (27.22) {400} | 0.91 |
2,068 kPa (20.41) {300} | 0.88 |
The table uses data from the JANAF thermochemical tables (Joint Army-Navy-NASA-Air Force (JANNAF) Interagency Propulsion Committee) throughout, with best-possible specific impulse calculated by Rocketdyne under the assumptions of adiabatic combustion, isentropic expansion, one-dimensional expansion and shifting equilibrium[12] Some units have been converted to metric, but pressures have not.
Definitions
- Ve
- Average exhaust velocity, m/s. The same measure as specific impulse in different units, numerically equal to specific impulse in N·s/kg.
- r
- Mixture ratio: mass oxidizer / mass fuel
- Tc
- Chamber temperature, °C
- d
- Bulk density of fuel and oxidizer, g/cm³
- C*
- Characteristic velocity, m/s. Equal to chamber pressure multiplied by throat area, divided by mass flow rate. Used to check experimental rocket's combustion efficiency.
Bipropellants
Oxidizer | Fuel | Comment | Optimum expansion from 68.05 atm to 1 atm |
Expansion in vacuum (0 atm) from 68.05 atm to almost 0 atm (Areanozzle = 40:1) | ||||||||
---|---|---|---|---|---|---|---|---|---|---|---|---|
Ve | r | Tc | d | C* | Ve | r | Tc | d | C* | |||
LOX | H2 | Hydrolox. Common. | 3816 | 4.13 | 2740 | 0.29 | 2416 | 4462 | 4.83 | 2978 | 0.32 | 2386 |
H2:Be 49:51 | 4498 | 0.87 | 2558 | 0.23 | 2833 | 5295 | 0.91 | 2589 | 0.24 | 2850 | ||
CH4 (methane) | Methalox. Many engines under development in the 2010s. | 3034 | 3.21 | 3260 | 0.82 | 1857 | 3615 | 3.45 | 3290 | 0.83 | 1838 | |
C2H6 | 3006 | 2.89 | 3320 | 0.90 | 1840 | 3584 | 3.10 | 3351 | 0.91 | 1825 | ||
C2H4 | 3053 | 2.38 | 3486 | 0.88 | 1875 | 3635 | 2.59 | 3521 | 0.89 | 1855 | ||
RP-1 (kerosene) | Kerolox. Common. | 2941 | 2.58 | 3403 | 1.03 | 1799 | 3510 | 2.77 | 3428 | 1.03 | 1783 | |
N2H4 | 3065 | 0.92 | 3132 | 1.07 | 1892 | 3460 | 0.98 | 3146 | 1.07 | 1878 | ||
B5H9 | 3124 | 2.12 | 3834 | 0.92 | 1895 | 3758 | 2.16 | 3863 | 0.92 | 1894 | ||
B2H6 | 3351 | 1.96 | 3489 | 0.74 | 2041 | 4016 | 2.06 | 3563 | 0.75 | 2039 | ||
CH4:H2 92.6:7.4 | 3126 | 3.36 | 3245 | 0.71 | 1920 | 3719 | 3.63 | 3287 | 0.72 | 1897 | ||
GOX | GH2 | Gaseous form | 3997 | 3.29 | 2576 | - | 2550 | 4485 | 3.92 | 2862 | - | 2519 |
F2 | H2 | 4036 | 7.94 | 3689 | 0.46 | 2556 | 4697 | 9.74 | 3985 | 0.52 | 2530 | |
H2:Li 65.2:34.0 | 4256 | 0.96 | 1830 | 0.19 | 2680 | |||||||
H2:Li 60.7:39.3 | 5050 | 1.08 | 1974 | 0.21 | 2656 | |||||||
CH4 | 3414 | 4.53 | 3918 | 1.03 | 2068 | 4075 | 4.74 | 3933 | 1.04 | 2064 | ||
C2H6 | 3335 | 3.68 | 3914 | 1.09 | 2019 | 3987 | 3.78 | 3923 | 1.10 | 2014 | ||
MMH | 3413 | 2.39 | 4074 | 1.24 | 2063 | 4071 | 2.47 | 4091 | 1.24 | 1987 | ||
N2H4 | 3580 | 2.32 | 4461 | 1.31 | 2219 | 4215 | 2.37 | 4468 | 1.31 | 2122 | ||
NH3 | 3531 | 3.32 | 4337 | 1.12 | 2194 | 4143 | 3.35 | 4341 | 1.12 | 2193 | ||
B5H9 | 3502 | 5.14 | 5050 | 1.23 | 2147 | 4191 | 5.58 | 5083 | 1.25 | 2140 | ||
OF2 | H2 | 4014 | 5.92 | 3311 | 0.39 | 2542 | 4679 | 7.37 | 3587 | 0.44 | 2499 | |
CH4 | 3485 | 4.94 | 4157 | 1.06 | 2160 | 4131 | 5.58 | 4207 | 1.09 | 2139 | ||
C2H6 | 3511 | 3.87 | 4539 | 1.13 | 2176 | 4137 | 3.86 | 4538 | 1.13 | 2176 | ||
RP-1 | 3424 | 3.87 | 4436 | 1.28 | 2132 | 4021 | 3.85 | 4432 | 1.28 | 2130 | ||
MMH | 3427 | 2.28 | 4075 | 1.24 | 2119 | 4067 | 2.58 | 4133 | 1.26 | 2106 | ||
N2H4 | 3381 | 1.51 | 3769 | 1.26 | 2087 | 4008 | 1.65 | 3814 | 1.27 | 2081 | ||
MMH:N2H4:H2O 50.5:29.8:19.7 | 3286 | 1.75 | 3726 | 1.24 | 2025 | 3908 | 1.92 | 3769 | 1.25 | 2018 | ||
B2H6 | 3653 | 3.95 | 4479 | 1.01 | 2244 | 4367 | 3.98 | 4486 | 1.02 | 2167 | ||
B5H9 | 3539 | 4.16 | 4825 | 1.20 | 2163 | 4239 | 4.30 | 4844 | 1.21 | 2161 | ||
F2:O2 30:70 | H2 | 3871 | 4.80 | 2954 | 0.32 | 2453 | 4520 | 5.70 | 3195 | 0.36 | 2417 | |
RP-1 | 3103 | 3.01 | 3665 | 1.09 | 1908 | 3697 | 3.30 | 3692 | 1.10 | 1889 | ||
F2:O2 70:30 | RP-1 | 3377 | 3.84 | 4361 | 1.20 | 2106 | 3955 | 3.84 | 4361 | 1.20 | 2104 | |
F2:O2 87.8:12.2 | MMH | 3525 | 2.82 | 4454 | 1.24 | 2191 | 4148 | 2.83 | 4453 | 1.23 | 2186 | |
Oxidizer | Fuel | Comment | Ve | r | Tc | d | C* | Ve | r | Tc | d | C* |
N2F4 | CH4 | 3127 | 6.44 | 3705 | 1.15 | 1917 | 3692 | 6.51 | 3707 | 1.15 | 1915 | |
C2H4 | 3035 | 3.67 | 3741 | 1.13 | 1844 | 3612 | 3.71 | 3743 | 1.14 | 1843 | ||
MMH | 3163 | 3.35 | 3819 | 1.32 | 1928 | 3730 | 3.39 | 3823 | 1.32 | 1926 | ||
N2H4 | 3283 | 3.22 | 4214 | 1.38 | 2059 | 3827 | 3.25 | 4216 | 1.38 | 2058 | ||
NH3 | 3204 | 4.58 | 4062 | 1.22 | 2020 | 3723 | 4.58 | 4062 | 1.22 | 2021 | ||
B5H9 | 3259 | 7.76 | 4791 | 1.34 | 1997 | 3898 | 8.31 | 4803 | 1.35 | 1992 | ||
ClF5 | MMH | 2962 | 2.82 | 3577 | 1.40 | 1837 | 3488 | 2.83 | 3579 | 1.40 | 1837 | |
N2H4 | 3069 | 2.66 | 3894 | 1.47 | 1935 | 3580 | 2.71 | 3905 | 1.47 | 1934 | ||
MMH:N2H4 86:14 | 2971 | 2.78 | 3575 | 1.41 | 1844 | 3498 | 2.81 | 3579 | 1.41 | 1844 | ||
MMH:N2H4:N2H5NO3 55:26:19 | 2989 | 2.46 | 3717 | 1.46 | 1864 | 3500 | 2.49 | 3722 | 1.46 | 1863 | ||
ClF3 | MMH:N2H4:N2H5NO3 55:26:19 | Hypergolic | 2789 | 2.97 | 3407 | 1.42 | 1739 | 3274 | 3.01 | 3413 | 1.42 | 1739 |
N2H4 | Hypergolic | 2885 | 2.81 | 3650 | 1.49 | 1824 | 3356 | 2.89 | 3666 | 1.50 | 1822 | |
N2O4 | MMH | Hypergolic, common | 2827 | 2.17 | 3122 | 1.19 | 1745 | 3347 | 2.37 | 3125 | 1.20 | 1724 |
MMH:Be 76.6:29.4 | 3106 | 0.99 | 3193 | 1.17 | 1858 | 3720 | 1.10 | 3451 | 1.24 | 1849 | ||
MMH:Al 63:27 | 2891 | 0.85 | 3294 | 1.27 | 1785 | |||||||
MMH:Al 58:42 | 3460 | 0.87 | 3450 | 1.31 | 1771 | |||||||
N2H4 | Hypergolic, common | 2862 | 1.36 | 2992 | 1.21 | 1781 | 3369 | 1.42 | 2993 | 1.22 | 1770 | |
N2H4:UDMH 50:50 | Hypergolic, common | 2831 | 1.98 | 3095 | 1.12 | 1747 | 3349 | 2.15 | 3096 | 1.20 | 1731 | |
N2H4:Be 80:20 | 3209 | 0.51 | 3038 | 1.20 | 1918 | |||||||
N2H4:Be 76.6:23.4 | 3849 | 0.60 | 3230 | 1.22 | 1913 | |||||||
B5H9 | 2927 | 3.18 | 3678 | 1.11 | 1782 | 3513 | 3.26 | 3706 | 1.11 | 1781 | ||
NO:N2O4 25:75 | MMH | 2839 | 2.28 | 3153 | 1.17 | 1753 | 3360 | 2.50 | 3158 | 1.18 | 1732 | |
N2H4:Be 76.6:23.4 | 2872 | 1.43 | 3023 | 1.19 | 1787 | 3381 | 1.51 | 3026 | 1.20 | 1775 | ||
IRFNA IIIa | UDMH:DETA 60:40 | Hypergolic | 2638 | 3.26 | 2848 | 1.30 | 1627 | 3123 | 3.41 | 2839 | 1.31 | 1617 |
MMH | Hypergolic | 2690 | 2.59 | 2849 | 1.27 | 1665 | 3178 | 2.71 | 2841 | 1.28 | 1655 | |
UDMH | Hypergolic | 2668 | 3.13 | 2874 | 1.26 | 1648 | 3157 | 3.31 | 2864 | 1.27 | 1634 | |
IRFNA IV HDA | UDMH:DETA 60:40 | Hypergolic | 2689 | 3.06 | 2903 | 1.32 | 1656 | 3187 | 3.25 | 2951 | 1.33 | 1641 |
MMH | Hypergolic | 2742 | 2.43 | 2953 | 1.29 | 1696 | 3242 | 2.58 | 2947 | 1.31 | 1680 | |
UDMH | Hypergolic | 2719 | 2.95 | 2983 | 1.28 | 1676 | 3220 | 3.12 | 2977 | 1.29 | 1662 | |
H2O2 | MMH | 2790 | 3.46 | 2720 | 1.24 | 1726 | 3301 | 3.69 | 2707 | 1.24 | 1714 | |
N2H4 | 2810 | 2.05 | 2651 | 1.24 | 1751 | 3308 | 2.12 | 2645 | 1.25 | 1744 | ||
N2H4:Be 74.5:25.5 | 3289 | 0.48 | 2915 | 1.21 | 1943 | 3954 | 0.57 | 3098 | 1.24 | 1940 | ||
B5H9 | 3016 | 2.20 | 2667 | 1.02 | 1828 | 3642 | 2.09 | 2597 | 1.01 | 1817 | ||
Oxidizer | Fuel | Comment | Ve | r | Tc | d | C* | Ve | r | Tc | d | C* |
Definitions of some of the mixtures:
- IRFNA IIIa
- 83.4% HNO3, 14% NO2, 2% H2O, 0.6% hydrogen fluoride
- IRFNA IV HDA
- 54.3% HNO3, 44% NO2, 1% H2O, 0.7% HF
- RP-1
- See MIL-P-25576C, basically kerosene (approximately C10H18)
- monomethylhydrazine
- CH3NHNH2
Has not all data for CO/O2, purposed for NASA for Martian-based rockets, only a specific impulse about 250 s.
- r
- Mixture ratio: mass oxidizer / mass fuel
- Ve
- Average exhaust velocity, m/s. The same measure as specific impulse in different units, numerically equal to specific impulse in N·s/kg.
- C*
- Characteristic velocity, m/s. Equal to chamber pressure multiplied by throat area, divided by mass flow rate. Used to check experimental rocket's combustion efficiency.
- Tc
- Chamber temperature, °C
- d
- Bulk density of fuel and oxidizer, g/cm³
Monopropellants
Propellant | Comment | Optimum expansion from 68.05 atm to 1 atm |
Expansion in vacuum (0 atm) from 68.05 atm to almost 0 atm (Areanozzle = 40:1) | ||||||
---|---|---|---|---|---|---|---|---|---|
Ve | Tc | d | C* | Ve | Tc | d | C* | ||
Hydrazine | Common | ||||||||
100% hydrogen peroxide | Common | 1610 | 1270 | 1.4 | 1040 | 1860 | 1270 | 1.4 | 1040 |
LMP-103s[13][14] | Recently test-flown | 1608[15] | |||||||
Nitromethane | |||||||||
Propellant | Comment | Ve | Tc | d | C* | Ve | Tc | d | C* |
References
- ↑ Sutton, G. P. (2003). "History of liquid propellant rocket engines in the united states". Journal of Propulsion and Power. 19 (6): 978–1007.
- 1 2 Sutton, E.P; Biblarz, O. (2010). Rocket Propulsion Elements. New York: Wiley.
- ↑ Larson, W.J.; Wertz, J. R. (1992). Space Mission Analysis and Design. Boston: Kluver Academic Publishers.
- ↑ Richard Rhodes, Dark Sun: The Making of the Hydrogen Bomb, 1995, pp. 483-504, Simon & Schuster, NY ISBN 978-0-684-82414-7
- ↑ Todd, David (2012-11-20). "Musk goes for methane-burning reusable rockets as step to colonise Mars". FlightGlobal Hyperbola. Retrieved 2012-11-22.
"We are going to do methane." Musk announced as he described his future plans for reusable launch vehicles including those designed to take astronauts to Mars within 15 years, "The energy cost of methane is the lowest and it has a slight Isp (Specific Impulse) advantage over Kerosene," said Musk adding, "And it does not have the pain in the ass factor that hydrogen has".
- ↑ Belluscio, Alejandro G. (2016-10-03). "ITS Propulsion – The evolution of the SpaceX Raptor engine". NASASpaceFlight.com. Retrieved 2016-10-03.
- ↑ "SpaceX propulsion chief elevates crowd in Santa Barbara". Pacific Business Times. 19 February 2014. Retrieved 22 February 2014.
- ↑ Belluscio, Alejandro G. (2014-03-07). "SpaceX advances drive for Mars rocket via Raptor power". NASAspaceflight.com. Retrieved 2014-03-07.
- ↑ "Firefly α - Firefly Space Systems". Retrieved 5 October 2014.
- ↑ "United Launch Alliance and Blue Origin Announce Partnership to Develop New American Rocket Engine". United Launch Alliance. Retrieved 5 October 2014.
- 1 2 3 4 http://www.braeunig.us/space/propel.htm
- ↑ Huzel, D. K.; Huang, D. H. (1971), NASA SP-125, "Modern Engineering for Design of Liquid-Propellant Rocket Engines", (2nd ed.), NASA
- ↑ "SSC". sscspace.com. Retrieved 22 May 2015.
- ↑ Anflo 1 23rd Annual AIAA/USU Conference on Small Satellites SSC09-II-4 EXPANDING THE ADN-BASED MONOPROPELLANT THRUSTER FAMILY K. Anflo
- ↑ https://uppsagd.files.wordpress.com/2012/03/advanced_monopropellants_combustion_chambers_and_monolithic_catalyst_for_small_satellite_propulsion.pdf
External links
- Cpropep-Web an online computer program to calculate propellant performance in rocket engines
- Design Tool for Liquid Rocket Engine Thermodynamic Analysis is a computer program to predict the performance of the liquid-propellant rocket engines.
- Clark, John D. (1972). Ignition! An Informal History of Liquid Rocket Propellants (PDF). Rutgers University Press. p. 214. ISBN 0-8135-0725-1. for a history of liquid rocket propellants in the US by a pioneering rocket propellant developer.