Lascar (volcano)
Lascar | |
---|---|
![]() The Lascar volcano, seen from the Chaxas lagoon on the Gran Salar. To the left, in the background, the Aguas Calientes volcano. | |
Highest point | |
Elevation | 5,592 m (18,346 ft) [1] |
Coordinates | 23°22′S 67°44′W / 23.367°S 67.733°WCoordinates: 23°22′S 67°44′W / 23.367°S 67.733°W [1] |
Geography | |
![]() ![]() Lascar Chile | |
Location | Northern Chile |
Parent range | Andes |
Geology | |
Mountain type | Stratovolcano |
Last eruption | October 30, 2015[2] |
Lascar, a stratovolcano of the Central Volcanic Zone of the Andes, is a volcanic arc that spans the countries of Peru, Bolivia, Argentina and Chile. It is the most active volcano of the region, with records of eruptions going back to 1848. It is constructed from two separate cones with six or five summit craters. The westernmost crater of the eastern cone is presently active. Volcanic activity is characterized by constant release of volcanic gas and occasional Vulcanian eruptions.
Lascar has been active since at least 56,000 or 220,000 years ago. The first known activity occurred at the eastern cone, before shifting to the western cone and back again. The magma supplied to the volcano ultimately comes from the subduction of the Nazca Plate beneath the South America Plate. A number of other volcanoes are found in the neighbourhood, such as Aguas Calientes and Cordon de Puntas Negras and the giant La Pacana caldera.
Major eruptions of Lascar occurred 26,500, 26,450 ± 500, and between 9,200 and 9,100 years ago. The second of these eruptions released 10–15 cubic kilometres (2.4–3.6 cu mi) of material and is known as the Soncor eruption. The largest historical eruption of Lascar occurred in April 1993 and was accompanied by ash fall as far as Buenos Aires. Because Lascar is located in a remote area, it is monitored primarily by remote sensing. Explosive eruptions are the biggest hazard at Lascar volcano.
Etymology and human interactions
There are two theories about how the name Lascar came to pass. The first derives the name from the Atacameno word Láskar or lassi, tongue. This name probably refers to the shape of the volcano.[3] Other names for the volcano are Toconado and Hlàscar.[4] Unlike the neighbouring volcano Acamarachi, Licancabur and Quimal, there is no evidence for archeological sites on Lascar,[5] possibly because of the volcanic activity.[6]
Lascar, like El Tatio, is a destination for volcano tourism.[7]
Geography and Geology
Regional setting
Volcanoes in the Andes occur in three separate regions, the Northern Volcanic Zone between 2°N and 5°S, the Central Volcanic Zone between 16°S and 28°S, and the Southern Volcanic Zone between 33°S and 46°S.[8] An additional Austral Volcanic Zone is found south of the Southern Volcanic Zone.[9] These volcanic zones are separated by areas where recent volcanism is absent; one common theory is that the subduction processes responsible for volcanism form an overly shallow subducting plate to trigger the formation of magma.[10] This shallow subduction appears to be triggered by the Nazca Ridge and the Juan Fernandez Ridge;[11] the areas where they subduct beneath the Peru-Chile Trench coincide with the limits of the Central Volcanic Zone.[12] Perhaps, when these ridges are subducted, the buoyancy they carry disrupts the subduction process and reduces the supply of water, which is important for the formation of melts.[13]
Of these volcanic zones, the Central Volcanic Zone is the largest, covering parts of Peru, Bolivia, Argentina and Chile.[14] The Central Volcanic Zone is located between two areas where subduction is shallower and volcanic activity absent. In the Central Volcanic Zone, volcanism has been active for 120 million years although it has undergone eastward migration during this time.[15] Water released from the subducting plate triggers the formation of basaltic magmas that are then injected into the crust.[16]
About 122 volcanoes with Holocene eruptions exist in the Andean Volcanic Belt. Many of them are covered by snow and ice. Ojos del Salado with a height of 6,887 metres (22,595 ft) is the highest volcano in the world.[9] A number of supervolcanoes exist in the Central Volcanic Zone in the Altiplano-Puna volcanic complex.[17]
Local setting



Lascar is part of the Central Volcanic Zone of the Andes.[18] The volcanism of Lascar relates to the subduction of the Nazca Plate beneath the South America Plate.[19][20] The Central Andes contain many hundreds of volcanoes, extending over the countries of Argentina, Bolivia, Chile and Peru. In this remote territory, where eruptions are poorly recorded, many volcanoes are higher than 6,000 metres (20,000 ft).[21] They are constructed on a crust that is between 50–70 kilometres (31–43 mi) thick.[8] Volcanic centres include calderas and associated large ignimbrites, lava domes, and stratovolcanoes.[18] The better researched centres include: Galan, Nevados de Payachata, Ollague, Purico Complex, San Pedro-San Pablo, La Pacana, Tata Sabaya, and Tumisa.[22] Over 44 volcanoes in the region are considered potentially active, with a number of young volcanoes featuring fumarolic or hydrothermal activity.[14][23] Guallatiri, for example, features fumarolic activity that is visible in space-born images.[24] Also fumarolically active are: Sabancaya, El Misti, Ubinas, Tacora, Isluga, Irruputuncu, Olca, Ollague, San Pedro, Putana and Lastarria.[25] The largest historical eruption occurred at Huaynaputina in 1600.[21] Given the low population density around many of these volcanoes, there is often little information on their activity.[26]
Lascar volcano is located in the Antofagasta Region of Chile,[25] and is 5,641 metres (18,507 ft),[27] 5,592 metres (18,346 ft),[25][18][8][1] 5,450 metres (17,880 ft),[28] or 5,641 metres (18,507 ft) high.[3][29] With a surface area of 54 square kilometres (21 sq mi), the volcano has a volume of 15 cubic kilometres (3.6 cu mi).[30] The Salar de Atacama is 30 kilometres (19 mi) farther west,[31] the terrain at Lascar dips in its direction.[32]
The new town of Talabre is 17 square kilometres (6.6 sq mi) west of Lascar. As of 2012, it had a population of 50 inhabitants.[14] As of 2002, Cattle breeding was the principal economic activity in Talabre.[33] Chile Route 23 passes c. 10 kilometres (6.2 mi) west of Lascar.[34]








Lascar is located in the main volcanic arc, on the western margin of the Altiplano.[20] Geographically, the area is located between the Salar de Atacama and the Altiplano.[18] Andesitic-dacitic Aguas Calientes is located 5 kilometres (3.1 mi) east of Lascar; it may have formed a lava flow close to the summit during the Holocene.[1][35] Lascar and Aguas Calientes might share a magma chamber.[36] Miocene-Quaternary volcanic centres in the neighbourhood include Cerro Negro in the north, Acamarachi northeast, Tumisa southwest, and the Cordon de Puntas Negras in the south.[37] Sometimes Lascar is considered part of the Cordon de Puntas Negras chain.[38] Tumisa to the south of Lascar was active between 2.5 and 0.4 million years ago.[39] It is constructed by dacite and surrounded by pyroclastic flow deposits.[40] Aguas Calientes is older than Lascar.[30] East of Lascar lies the La Pacana caldera.[39]
Cerro Opla 20 kilometres (12 mi) west of Lascar is a hill formed by Permian-Triassic granite.[41] An area of increased electrical conductivity has been identified beneath Lascar and extends to some neighbouring volcanoes, reaching a depth of over 6 kilometres (3.7 mi) south of Lascar.[42]
The 9 kilometres (5.6 mi) long Quebrada de Chaile, the 17 kilometres (11 mi) long Quebrada de Soncor, and the 17 kilometres (11 mi) long Quebrada de Talabre canyons run towards Salar de Atacama; they are 30–80 metres (98–262 ft) deep and 80–500 metres (260–1,640 ft) wide.[43] These valleys probably formed from erosion during glacial periods.[30] These valleys drain the western, northern and southwestern slopes of Lascar. The southeastern slopes drain into the Laguna Lejia and the northwestern slope drains through the Quebrada de Morro Blanco.[34]
Lascar is constructed on top of a ridge formed by the 5,293 metres (17,365 ft) high Cerro Corona and 5,192 metres (17,034 ft) high Cerro de Saltar lava domes south and north of Lascar respectively.[39][44] Cerro Corona gets its name from a crown shaped structure at its top.[45] These domes cover a surface area of about 90 square kilometres (35 sq mi).[40] These lava domes are about 5 million years old.[46] They are constructed by dacite and smaller amounts of two–pyroxene andesite.[44] Rhyolite is also found. Minerals include biotite and hornblende.[40] An eruption 16,700 years ago from Corona deposited tephra containing biotite and quartz in the Laguna Lejia and generated a rhyodacitic lava flow. Another debris flow from Corona flowed towards Salar de Atacama.[30]
Geology
Lascar volcano is formed by two truncated cones with irregular shapes that extend east-west.[47][48] Aguas Calientes is also located on this trend.[49] Six craters are located on the volcano,[23] showing evidence that activity has migrated westward.[29] Sometimes only five craters are counted, in which case the central crater is considered to be the active one.[50] The extinct western cone (also known as Apagado) is constructed by layers of lava and pyroclastics. Its large crater is filled by another cone,[49] which forms the highest summit of the Lascar system.[3] Immediately east of it lies the eastern cone which is contiguous with the western cone. The eastern cone (also known as Activo)[49] is capped off with three distinct craters. Of these the eastern crater had the largest diameter of 1 kilometre (0.62 mi) but the western crater was the active centre in 1964.[48] Measurements made in 1961–1997 determined that the eastern crater is 1 kilometre (0.62 mi) wide and 150–200 metres (490–660 ft) deep, the central crater is 600 metres (2,000 ft) wide and 100–200 metres (330–660 ft) deep and the western crater is 800 metres (2,600 ft) wide and 200–300 metres (660–980 ft) deep. These craters are not collapsed calderas.[51] Traces of a previous edifice are visible in the craters; this edifice constitutes the bulk of the eastern cone. Traces of a sector collapse towards the northeast and an associated horseshoe-shaped scar are found.[49]
The western of these eastern craters is the currently active one, surrounded by rims that reach heights of 150 metres (490 ft). In 1985, a 150 by 150 metres (490 ft × 490 ft) hot spot in this crater was observed in satellite images.[29] As of 2006, this active crater is 150 metres (490 ft) deep and 800 metres (2,600 ft) wide. In its centre lies a smaller crater, 250 metres (820 ft) deep and 300 metres (980 ft) wide. Many fumaroles occur along the rim of the inner crater.[52]


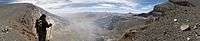
Large lava flows are noticeable on the flanks of the volcano.[29] They extend from the summit craters, although none of them appears to be associated with the currently active crater.[28] A total of eight lava flows has been recognized.[53] Flows from the first stage of Lascar's activity are exposed at its western foot.[49] On the eastern flank, lava flows are buried beneath pyroclastic material.[54] A 6 kilometres (3.7 mi) long lava flow on the northern flank reaches almost to Talabre.[29] This lava flow is known as the Tumbres-Talabre lava flow, its margins are 10–40 metres (33–131 ft) high and it features a central channel. The flow advances just north of the head of Quebrada Talabre before entering it over cliffs.[55] Another lava flow on the southwest flank is known as "Capricorn Lava".[46] This dacitic lava was erupted high on Lascar and has a blocky surface. It features well developed levees and a 10 metres (33 ft) thick flow front. Its rocks have a pale gray-blue colour and compositionally resembles the Soncor flow, despite more mafic lavas and pyroclastics being erupted between the times these two were erupted.[56]
An early pyroclastic flow, the Saltar Flow, is exposed on the eastern flank. It was emplaced after the collapse of the oldest edifice, covering Aguas Calientes' western slopes. It was later modified by glacial activity.[49] The Soncor flow is found on the western side of Lascar, part of it also southeast of Lascar. On the western slope, it buries the even older Piedras Grandes flow which only crops out at its margins.[57] While the Piedras Grandes flow was formed by a glacier run that transported blocks with sizes of up to 8 metres (26 ft), Soncor was formed by a large eruption. It is a pyroclastic flow that extended 27 kilometres (17 mi) westward and contained breccia and various magmas. It was accompanied by a Plinian fall deposit. The andesitic pumice Tumbres flow is found on the northwest-west-southwestern slopes of Lascar.[58]
The Quebrada Talabre cuts into the upper flanks of Lascar. Lahar deposits are found in adjacent valleys, suggesting that wetter periods had occurred during Lascar's activity.[55] Traces of glacial action are found on the older parts of Lascar at altitudes above 4,600 metres (15,100 ft) and include meltwater gorges, striated rock surfaces, and U-shaped valleys.[59] Moraines are found at Tumisa down to an altitude of 4,850 metres (15,910 ft).[30] The Quebrada Talabre was scoured by pyroclastic flows from the 1993 eruption, exposing bedrock and Tertiary ignimbrites.[60] The Quebrada Talabre later becomes a tributary of the Quebrada Soncor.[34]
The volcano sits above a major local geological trend, the north -south Miscanti Line. Other volcanic centres are also located on this line,[49] including the Corona and Saltar lava domes and the Miscanti and Lejia volcanoes.[30][61] The Miscanti line may be a hinge of a fold that is being propagated by faults.[54] The formation of the first cone at Lascar may have been facilitated by the intersection between the Miscanti Line and another east-west oriented lineament, which would have worked as an ascent path for magma.[62] At least four alignments of volcanoes are recognized in the region.[63]
Composition
Lascar rocks consist of andesite and dacite. These rocks have a "two pyroxene" composition, although the old Piedras Grandes and Soncor rocks contain hornblende. Other minerals include anhydrite,[51] augite, and plagioclase.[27] Additional minerals are: apatite, ilmenite, magnetite, olivine, orthopyroxene, phyrrotite, quartz, rhyolite in the groundmass, and spinel in inclusions. Dacite has more plagioclase and rhyolite.[58] Component chemicals found at Lascar include: anorthite, augite bordering on diopside, bronzite, fassaite, forsterite, hypersthene, pigeonite, and more.[64]
The rocks of Lascar belong to the calc-alkaline series.[65] SiO
2 contents range from 55.5–67.8% by weight, and the rocks have medium to large concentrations of potassium.[66] The magmas are contaminated by the local crust, but not to the extent noted in the Galan or Purico Complex for example.[67] The chemistry of Lascar's rocks is fairly similar to neighbouring Tumisa.[68]
Magma erupted by Lascar appears to form from the mixing of mafic and more evolved magmas; the 1993 eruption deposits contain bands of different rocks.[51] Specifically, basaltic andesite magma is periodically injected into a magma chamber where crystal fractionation and mixing processes take place.[69] The process happens frequently, thus the magmas are relatively unevolved.[70] Presumably, if the supply of mafic magma is steady the products are andesitic, otherwise dacite forms.[70] This mixture origin of Lascar magmas is reflected in the textures of rocks.[71] The overall magma supply rate of Lascar is 0.02–0.03 cubic metres per second (0.71–1.06 cu ft/s).[72]
The magma chamber of Lascar appears to lie at depths of 10–17 kilometres (6.2–10.6 mi),[73] although the lack of deformation of the edifice during the 1993 eruption indicates it may be deeper, over 25–30 kilometres (16–19 mi) or even over 40 kilometres (25 mi) deep.[74] There appear to be two distinct chamber systems, an andesitic one that is responsible for the frequent andesite lava and pyroclastic flow activity, and a dacitic one that was involved in the Piedras Grandes and Soncor activities.[75]
Temperatures of the magma chamber range 890–970 °C (1,630–1,780 °F), the mafic magmas that are injected in the chamber are about 150–200 °C (302–392 °F) hotter than the extant andesite and dacite. The chamber may be surrounded by skarnic alteration.[76] This alteration gives rise to wollastonite and pyroxene-containing skarn depending on the distance from the magma chamber walls. Metasomatism does further affect rocks derived from magma chamber walls.[77] The conditions at the magma chamber may be comparable to these under which epithermal mineral deposits form.[78] The oxidation conditions in the magma chamber are favourable for the formation of sulfate,[79] but unfavourable for the deposition of sulfide minerals.[80]
A number of xenoliths occur in Lascar's rocks, a large amount of the phenocrysts are ultimately derived from them. Hornfels, skarn and rocks derived from the lava dome ridge Lascar is constructed on form these xenoliths. Minerals encountered in xenoliths include: andradite, anhydrite, anorthite, apatite, biotite, calcite, diopside, fassaite, garnet, gypsum, ilmenite, magnetite, monazite, orthopyroxene, perovskite, plagioclase, prehnite, quartz, sphene, thorite, wilkeite, wollastonite and zircon. A number of such xenoliths formed from carbonate rocks that were influenced by magma[81][70] from Lascar and other volcanoes such as Tumisa.[76]
Gas emissions
Lascar emits plumes of gas. Sometimes, white clouds of vapor are released.[23] The bulk of this gas release occurs over many hundreds of fumarolic vents, which are mostly located in the active crater.[52] In December 2002, two fumaroles had temperatures exceeding 295 °C (563 °F).[82] Total flux is estimated to be 1,312–18,469 kilograms per second (173,500–2,443,000 lb/min).[73]
There are noticeable differences between the high and low temperature fumaroles, for example the latter tend to have far more water than carbon dioxide. Also found are carbon monoxide, hydrogen, hydrogen chloride, hydrogen sulfide and smaller amounts helium. Hydrocarbons and other organic compounds are also found in the low temperature fumaroles.[83] Trace elements include arsenic, boron and titanium, with smaller amounts of barium, chromium, copper, lead, strontium and zinc.[84] Sulfur is released all over the cone, resulting in a noticeable sulfur smell.[62]
Total amounts of SO
2 released amounted to 27 kilograms per second (3,600 lb/min) in 1989,[85] and 28 kilograms per second (3,700 lb/min) in 2003.[86] Overall sulfur output ranges between 200–2,300 tonnes per day (2.3–26.2 long ton/ks).[52][87] This corresponds to about 1% of global volcanic sulfur, and is comparable to Kilauea and Villarica.[88] Lascar is a substantial source of sulfur dioxide for the atmosphere around 30° south, reaching 20–40% over South America and still 10–20% over the South Indian Ocean.[89][90] In 2005, Lascar was the third-largest source of volcanic sulfur dioxide in the world among continuously active volcanoes, behind Etna in Italy and Bagana in Papua-New Guinea.[91] There are temporal variations in the output, for example after a decrease in 2009 sulfur output increased in 2012, probably as a consequence of the arrival of new magma in depth.[92]
Hydrogen chloride and hydrogen fluoride are also released in large amounts, with estimates made in 2003–2004 indicating a mass flux of 340,000,000,000 grams per year (380,000,000 oz/Ms) and 150,000,000,000 grams per year (170,000,000 oz/Ms) respectively.[93] They correspond to about 2 and 5%, respectively, of the global volcanic flux of these compounds.[94]
Emission of other elements:
Element | Output | Source |
---|---|---|
Arsenic | 220 kilograms per day (5.6 lb/ks) | [95] |
Boron | 370 kilograms per day (9.4 lb/ks) | [95] |
Copper | 20 kilograms per day (0.51 lb/ks) | [95] |
Lead | 10 kilograms per day (0.26 lb/ks) | [95] |
Zinc | 70 kilograms per day (1.8 lb/ks) | [95] |
Sulfate dusts are also released in large amounts,[93] about 100000 trillion particles per second.[86]
Gases are partly supplied from shallow magma, considering that the volume of magma erupted is too small to contain all the exhalations.[96] The release of gas by the magma is favoured by strong temperature contrasts between incoming magma and the magma chamber.[79] This may explain the high emission of sulfur dioxide by Lascar.[97] The presence of argon and nitrogen in low-temperature fumaroles indicates that air is involved in their formation,[83] although part of these two gases are non-atmospheric.[98]
Sulfur and chlorine may be derived from the crust, evaporites such as these found at Salar de Atacama, subducted lithosphere or the mantle. Carbon in the gases may come from skarn assimilation.[99] Sulfur isotope data support the notion that evaporite deposits contribute part of Lascar's sulfur.[100] Water appears to be in part magmatic and in part precipitation-derived.[101] The high concentrations of halogens are typical for subduction associated volcanoes; the halogens are supplied to the volcanoes through subduction.[88]
The heat output of Lascar is about 75–765 megawatts (101,000–1,026,000 hp) during regular activity.[102] Electrical conductivity data suggest that a hydrothermal system exists beneath Lascar.[103] The existence of such a system has however been questioned.[104]
Basement
Lascar is constructed on the Atana ignimbrite, a rhyodacitic sheet which was erupted by La Pacana caldera 4.5–3.7 million years ago.[39] The Pampa Chamaca and Tuyajto ignimbrites are somewhat younger, 2.6–2.2 and less than one million years respectively. These ignimbrites form a 3° slope in the area.[30][40] Other basement rocks are the sandstone-containing marine Devonian-Carboniferous Lila formation, and the red-orange Permian Cas formation containing volcanic rocks and granites,[18][31] as well as the volcanic Permian-Triassic Peine formation and Cerro Negro strata, which also contain intruded rocks and lake sediments.[49] These formations are not visible in the Lascar area, but they crop out close to Salar de Atacama.[20] Tertiary sediment and volcanic rocks can also be found.[18] The presence of Mesozoic limestone is indicated by xenoliths in Lascar's lavas; they only crop out farther east in Argentina.[30] This limestone formation has been identified as the Yacoraite formation.[80] Later deposits include the Cenozoic sedimentary Quepe strata. Landforms over this basement include ignimbrites, lava domes, and stratovolcanoes.[49] Exposures of the basement are often limited by faults.[54]
Climate and biota
The area of Lascar belongs to the driest and highest volcanic settings in the world.[105] Precipitation at Lascar is about 50–100 millimetres per year (0.062–0.125 in/Ms) and consists mostly of snow.[62] Persistent snow exists on the western and southern slopes of the volcano, it contributes partly to the fumarole water.[83] In 1993, yearly precipitation at several towns around Lascar ranged from 2.5–20.1 millimetres (0.098–0.791 in). Lascar is located close to the Atacama Desert, one of the world's driest deserts.[106]
During the glacial periods, the volcano most likely featured small glaciers. The equilibrium line at Lascar was at an altitude of 4,700–4,800 metres (15,400–15,700 ft).[30] Traces of glaciation also exist at Cerros de Saltar.[40] The end of glaciation may have accompanied an increase in volcanic activity, a phenomenon that has been noted in other volcanoes in the world.[107] After 8,500 years ago, the climate in the region became much drier and the amount of erosion decreased substantially.[108]
Temperatures in the surrounding region range between −25–40 °C (−13–104 °F).[105] Measurements made on the southwest rim of the main crater in 2009–2012 indicated air temperatures of 10–20 °C (50–68 °F).[14] The present-day snowline in the region lies at an altitude of 6,050 metres (19,850 ft).[109]

Due to the dry climate, there is little vegetation at Lascar. Bunch grass and shrubs grow on slopes. In the deep valleys, groundwater and streams can support more plants.[106]
Volcanic activity at Lascar does affect neighbouring ecosystems such as the Aguas Calientes crater lake and Laguna Lejia; flamingos disappeared from the latter after the 1993 eruption and did not return until 2007.[110] Other reports claim that flamingos remained; other animals like donkeys and llamas were seen around the volcano one day after its eruption.[111]
Eruptive history
Lascar volcano is the most active volcano in the Andean Central Volcanic Zone.[112] A tall plume of water and sulfur dioxide persists over it.[43] Most present-day activity consists in the release of fumarolic gas with additional Vulcanian activity that generate eruption columns several 1 kilometre (0.62 mi) high.[113] The long-term magma supply rate of Lascar is about 0.08 cubic kilometres per millennium (0.00061 cu mi/Gs).[114]
Early activity
The oldest volcanic activity at Lascar occurred less than 50,000,[48] or 220,000 years ago.[14] Activity has alternated between the eastern and western part of the volcano during its history. The eastern edifice formed first ("stage I"), erupting andesite containing pyroxene and eventually forming the Chaile and Saltar pyroclastic flows.[48] Lava flows less than 50 metres (160 ft) issued from the stage I cone and reached lengths of 16 kilometres (9.9 mi). They occur beneath altitudes of 4,100 metres (13,500 ft), their vents buried by later activity.[30] The oldest mafic andesites are less than 43,000 years old, while the Chaile and Saltar pyroclastic flows were erupted over 26,500 years ago.[35] An alternative dating scheme considers Chaile to be 47,000 ± 16,000 years old and Saltar 167,000 ± 9,000 years old.[115] The lavas from the stage I are mostly exposted north and west of Lascar. The Chaile flows are actually formed by two separate units and are found on the southwest flanks of the volcano, up to a distance of 6 kilometres (3.7 mi).[35] They reach thicknesses of 5 metres (16 ft) in the upper unit,[116] and 30 metres (98 ft) in the lower one. The Saltar flow reached widths of 0.7–1.3 kilometres (0.43–0.81 mi) and thicknesses of 5–20 metres (16–66 ft), increasing to 35 metres (115 ft) where the flow had entered valleys. At least nine units form the Saltar deposit, with the northern flows displaying flow welding.[117] These deposits have volumes of 0.1 cubic kilometres (0.024 cu mi) and probably formed when an explosive eruption took place in a lava lake.[72] After the end of stage I, a period of glacial erosion occurred prior to new activity,[62] which created furrows in the Saltar flow. Imprecise argon-argon dating on younger andesites has yielded dates of 14,000 ± 18,000 and 17,000 ± 22,000 years.[117]
Later volcanic activity buried this edifice beneath thin pyroclastic flows. The western edifice formed a complex of lava domes ("stage II").[48] This complex was probably surrounded by a horseshoe-shaped crater open to the west.[118] Possibly, the magma chamber of stage I had almost solidified when the injection of basaltic magma at depths of over 5 kilometres (3.1 mi) triggered a remelting.[119] Andesite-rhyodacite intrusions occurred beneath the volcano,[112] some of these were still hot when the Soncor eruption tore them out of the ground.[120] An ice cap formed over Lascar at that time, feeding two glaciers that extended northeast and southeast away from the volcano.[58]
Piedras Grandes unit
This activity was accompanied by the eruption of block-and-ash flows consisting of andesite, and an eruption whose deposits include blocks with sizes of 15 metres (49 ft). This unit formed by stage II is also known as Piedras Grandes.[48] This unit is exposed on the western slopes below about 4,900 metres (16,100 ft) altitude and is about 2 kilometres (1.2 mi) wide.[118] It consists of large blocks encased in ash.[43] The composition of the Piedras Grandes unit is andesite containing amphibole, basaltic andesite and hornblende.[75] The Piedras Grandes unit is over 26,500 years old,[35] possibly between 100,000 - 63,000 years old.[115] Temperatures have been estimated to be 740–1,060 °C (1,360–1,940 °F) for the andesite and 1,130–1,220 °C (2,070–2,230 °F) for the basaltic andesite.[121] The magmas were formed from a remelted proto-pluton that had been heated and resupplied with volatiles by mafic magmas.[122]
The lava domes interacted with glaciers, resulting in the formation of a glacier run whose deposits are found as far as 10 kilometres (6.2 mi) from the volcano.[75] Blocks with sizes of up to 15 metres (49 ft) were transported by this flow.[118] An alternative theory posits that the Piedras Grandes unit formed when an ice cap on Lascar interacted with a block-and-ash flow erupted by Aguas Calientes.[58]
Soncor eruption
26,450 ± 500 years ago,[112] a major Plinian eruption occurred, erupting 10–15 cubic kilometres (2.4–3.6 cu mi) of ejecta between ash and pyroclastic flows. The deposits left contain both andesite and dacite,[48] with phenocrysts consisting of apatite, augite, biotite, iron-titanium oxides, orthopyroxene and plagioclase in a rhyolite matrix.[123] The Plinian deposit has a colour ranging from white to creamy.[124] Like the Piedras Grandes rocks, they tend towards high potassium quantities and resemble other volcanic rocks of the Central Andes and Lascar in terms of composition.[125] The deposits are formed by a Plinian fallout deposit and an ignimbrite rich in lithics.[43] This Plinian deposit reaches thicknesses of 22 metres (72 ft) and fell from a 22–30 kilometres (14–19 mi) high eruption column.[124]
The Soncor ignimbrite extended as much as 27 kilometres (17 mi) west from the volcano,[43] 10 kilometres (6.2 mi) north and 15 kilometres (9.3 mi) south.[117] It is white, heterogeneous,[75] mostly featureless with only weak sorting,[126] but features a noticeable compositional zonation.[127] The ignimbrite features three facies, one rich in breccia, another rich in pumice, and a normal ignimbrite.[124]
The magma erupted was formed in a magma chamber starting from andesite, which underwent complex petrogenetic processes.[128] This magma chamber existed at a floor depth of 5–6 kilometres (3.1–3.7 mi) (older estimate 12–22 kilometres (7.5–13.7 mi)[129]) and probably had a complex shape given certain chemical properties of the Soncor rocks. At the time preceding the eruption, the magma chamber had a thermal stratification,[130] injections of mafic magmas had heated the magma chamber and induced convection.[122]
Ignimbrite was channeled to the Salar de Atacama by the Quebrada de Chaile, Quebrada de Soncor, Quebrada de Talabre canyons and some smaller valleys, northeastwards by the Quebrada de Morro Blanco, and as far as 11 kilometres (6.8 mi) southeastwards over the Pampa Leija area.[43] In these valleys, the ignimbrite can be as much as 60 metres (200 ft) thick.[126] Pumices are encased in the ignimbrite as lenses and levees and are also found in the terrain above the canyons. Estimated temperatures decreased from 800–900 °C (1,470–1,650 °F) at the vent to 580–600 °C (1,076–1,112 °F) farther down the flows.[131] At the time of emplacement, the ignimbrite was still 200–300 metres (660–980 ft).[132] Magma temperatures have been estimated at 900–1,000 °C (1,650–1,830 °F).[121] The Soncor fallout deposit contains a basal gravelly layer and several layers of andesitic and dacitic pumice which also contain lithics.[133] The total volume of the Soncor eruption products has been estimated to be 5.6 cubic kilometres (1.3 cu mi) dense rock equivalent or 10 cubic kilometres (2.4 cu mi). Lithic rocks derived both from the pre-Soncor volcano and the basement are also represented.[126]
A volatile phase formed inside the magma chamber, containing chlorine and quickly removed most sulfur from the magma. This sulfur extraction was facilitated by the high content of oxygen in the magma which facilitated the formation of sulfur dioxide.[130] Water is a principal volatile and involved in the processes of Plinian eruptions; the water content of the Soncor and Piedras Grandes magmas was about 4–5%.[122] The Soncor magmas were associated with a volatile phase that underwent extensive interaction with the future eruption products.[134]
The previous volcanic edifice was destroyed by this eruption;[75] a caldera may have formed then.[58] The vent was no wider than 2 kilometres (1.2 mi) as it is completely hidden beneath the western cone.[135] Such a vent or caldera is substantially smaller than the volume of rocks erupted, a discrepancy that is also evident in the 1932 eruption of Quizapu. Perhaps the Soncor magma chamber was too deep to collapse when it was emptied.[72]
The Soncor deposit was subsequently affected by glaciation.[58] Subsequently, the stage I edifice was affected by a debris avalanche,[35] which was radiocarbon dated at 22,310 +2700 -2000 years ago in the Quebrada de Chaile.[136] This debris avalanche is 50 metres (160 ft) thick and 25 kilometres (16 mi) long.[135] The Capricorn Lava overlies the Soncor deposits.[134]
Post-Soncor activity
Starting 22,300 years ago,[35] a new stratovolcano grew over the Soncor vent.[48] This volcano was formed by andesite-dacite lava flows ("stage III") and scoria.[43] Lava flows from this stage have thicknesses of 20–60 metres (66–197 ft) and lengths reaching 5 kilometres (3.1 mi). It has a volume of 5–6 cubic kilometres (1.2–1.4 cu mi).[137] The growth of this volcano was preceded by a period of erosion between 20,100–20,800 and 12,500 years ago, coincident with the Lake Minchin humid period.[138] Glaciers in the region reached their maximum size at that time.[139] The deposits left by this erosional period contain no clear evidence of stage III activity; indeed Lascar was probably inactive between 14,000 and 10,500 years ago. However, an eruption of the Cerro Corona lava dome occurred during this period.[137]
Around 7250 BCE,[140] or 9,200 and 9,100 years ago, the Tumbres eruption occurred. This eruption commenced with the eruption of pumice falls that reach thicknesses of less than 1.2 metres (3 ft 11 in). Afterwards, up to four 1–10 metres (3 ft 3 in–32 ft 10 in) thick different units of pyroclastic flows formed deposits up to 10 kilometres (6.2 mi) long.[137] At the end of the eruption, a 1.5 kilometres (0.93 mi) wide caldera formed.[112] The deposits left by this eruption contain basaltic andesite-andesite and have been subject to agglutination and welding.[43] Originally considered part of stage III, it was more recently attributed to stage IV given the considerable (6,000 years) temporal gap between the Tumbres eruption and stage III and the geochemistry of the rocks.[112] The Manquez agglutinate above the Tumbres deposits was formed either by the Tumbres eruption or by the next stage.[137] A pyroclastic cone in the western crater may be associated with this agglutinate.[115]
Activity subsequently shifted to the eastern edifice.[48] Around 5150 ± 1250 BCE as obtained by surface exposure dating,[140] the Tumbres-Talabre lava flow was erupted from the eastern crater.[140][48] This flow extends 8 kilometres (5.0 mi) northwest and is 20–30 metres (66–98 ft) thick.[43][141] The Tumbres-Talabre flow was originally considered to be of late 19th century age.[29] It probably formed when one of the craters filled with andesitic lava to the point of overflow.[137] The three eastern summit craters formed at the time when the Tumbres-Talabre flow was erupted in remains of the stage I cone.[112] Layers of lava and pyroclastics are discernible in the craters.[137] This edifice is the currently active one, with the deepest of its three summit craters being active.[48]
Historical activity
Lascar has erupted about thirty times since the 19th century.[87] Reports of volcanic activity exist since the 16th century, when the Spaniards arrived in the region.[142] Some early reports of volcanic activity at Aguas Calientes probably actually refer to Lascar.[4] Few records exist from before 1848.[29] Volcanic activity recorded after 1848 chiefly consists of fumarolic emissions and occasional explosive activity.[51] For some eruptions it is not clear whether they occurred at Lascar or Aguas Calientes, e.g. the January 1854 eruption. Recorded eruptions occurred in 1858, 1875, 1883–1885, 1898–1900 (?) and 1902, ranging from a VEI (volcanic explosivity index) of 0 to VEI 2.[140] The 1933 eruption was seen as far as Chuquicamata.[143] Another series of eruptions occurred between November 1951 and January 1952; one eruption is recorded from 1940.[140][144] Eruptions were observed in March 1960, which were accompanied by earthquakes felt in Toconao, as well as in September 1964 when ash fell in Socaire.[29] Yet another eruption sequence occurred between 1959 and 1968 and 1969. Eruptions in 1972 and 1974 are uncertain.[140]
In 1984 Lascar awakened to new activity,[51] when satellite images noted the presence of hot spots on the volcano.[113] Landsat images taken during this time indicate that a lava lake may have existed in the central crater[145] generating a plume of volcanic gases and, in September 1986, a vulcanian eruption that dropped ash in Salta, Argentina.[51] This eruption was first noticed when ash fell on Salta and was accompanied by anomalies in the heat emission from the volcano recorded by satellite.[23] The eruption was also observed by geologists in Toconao,[146] where the explosion was violent enough to wake people who were sleeping. Observers noted the formation of a cauliflower-shaped cloud that eventually developed into a mushroom cloud with a maximum height of 9.4 kilometres (5.8 mi) above the volcano.[147] The eruption itself lasted only about five minutes and consisted of two pulses. Ash fall in Salta occurred about one hour after the eruption.[146] This eruption was the most significant of the two decades,[145] having a VEI of 3. Preceding historical eruptions do not exceed 2.[28]
A 200 metres (660 ft) wide and 50 metres (160 ft) high lava dome formed in early 1989. This dome began to shrink in October 1989 and in December 1989 white clouds rose 2 kilometres (1.2 mi) above Lascar's crater. On the 20 February 1990, an eruption column rose 8–14 kilometres (5.0–8.7 mi) above the crater,[51] resulting in ash fall over 100 kilometres (62 mi) away from the volcano.[113] In March 1990, the lava dome had a temperature of 100–200 °C (212–392 °F) with some parts exceeding 900 °C (1,650 °F).[113] Lava bombs with diameters of up to 1.5 metres (4 ft 11 in) were hurled as far as 4 kilometres (2.5 mi) from the crater, presumably as a consequence of the lava dome exploding. Some of the material came from the conduit walls. The lava dome had disappeared, but in early 1992 another lava dome formed, eventually reaching a size of 180–190 metres (590–620 ft) width and 40 metres (130 ft) height and was accompanied by explosions. It probably started shrinking in April 1992, although the shrinkage was directly visible only in November. Small explosions accompanied the shrinkage until by March 1993 the dome had disappeared again.[148]
An alternating cycle of fumarolic activity, an accumulation of fumarolic gases in the conduit and lava dome, explosive activity followed by renewed fumarolic activity has characterized Lascar's activity since 1984. Explosive activity presumably occurs when gases can no longer escape.[65] This occurs because as the magma loses its gas content, the amount of pores in it and thus the permeability to gas decrease. Further, fractures permitting gas passage are obstructed when the magma contracts.[149] During most of the time, numerous fumaroles within the crater form a plume that reaches an altitude of 1,000 metres (3,300 ft). During minor explosive eruptions, eruption columns reach heights of no more than 5,000 metres (16,000 ft).[150] The temperatures of the lava dome can reach 148–367 °C (298–693 °F).[151] This cycle ended after 1993, probably because the April 1993 eruption modified the conditions in the volcanic system.[96] Alternatively, the cycle may have continued, to reach another lava dome collapse stage in early 2003.[152] While eruptions before 1993 had always been preceded by a reduction in heat radiated from the volcano, such a reduction in 1999–2000 did not lead to an eruption, and when an eruption took place in July 2000, it was preceded by only a brief drop in heat radiation.[153]
1993 eruption
Vulcanian explosions occurred 18 April 1993, and on the 19–20 April 1993, a major eruption occurred.[148] A phreatic eruption around 14:30 of 18 April formed the prelude to the eruption.[154] The eruption commenced with two explosions at 6:28 and 9:20 local time, forming eruption columns 10 kilometres (6.2 mi) high. Another explosion at 13:02 sent a column 8.5 kilometres (5.3 mi) high.[28] At least ten different pulses were observed, which generated columns of various height.[155] Eruption columns formed mushroom clouds.[156] The 1993 eruption lasted 23 hours. The eruption column underwent several collapses, creating pyroclastic flows at least nine[157] or seven times. The strongest pulse occurred on 20 April between 6.28-9:20 and sent flows towards the northwest. This pulse generated an eruption column 23 kilometres (14 mi) high.[158] The total mass flux of the eruption was about 10,000,000–100,000,000 kilograms per second (1.3×109–1.32×1010 lb/min), comparable to the 1982 eruption of El Chichon.[159] The lava dome in the crater was destroyed and was probably the source of the lava bombs that were thrown as far as 4 kilometres (2.5 mi) away from the vent.[156]
The first pyroclastic flow was observed around 10:12.[154] Other flows occurred on 19 April at 12:05, after 13:37, 17:25, 21:35–21:48, 23:40–23:50 and on 20 April at 9:20.[160] After being discharged through gaps in the crater rim,[158] pyroclastic flows on the northwestern and the eastern sides reached lengths of 8.5 kilometres (5.3 mi),[161] and 4 kilometres (2.5 mi) on the southern side.[162] These flows reached a thickness of about 5–10 metres (16–33 ft) and advanced through the Quebrada de Talabre on the northern flank, which had intercepted the northern flows. On the southeastern flank, the pyroclastic flows formed a fan extending several hundred 1 metre (3 ft 3 in) into Pampa Leija. Pyroclastic flows reached a speed of 55 metres per second (180 ft/s),[157] and themselves generated ash surges that partly rose above the flows.[163] Hot pyroclastic flows on the southeastern flank covered a surface area of 13–18.5 kilometres (8.1–11.5 mi).[164] The southern flank flows at first proceeded along a gully before spreading out.[165] The total area covered by the flows is about 14.2 square kilometres (5.5 sq mi) on the northern slopes and 4.3 square kilometres (1.7 sq mi) on the southern slopes.[166] The speed of these flows has been estimated at 100–700 kilometres per hour (62–435 mph).[28]
About 30% of these flows was formed by ash and 70% by blocks.[156] The pyroclastic flow deposits contain lithics from several sources and pumice.[167] Pumice mostly accumulated on the surface of the flows and individual stones are up to 30 centimetres (12 in) wide.[158] Lithic blocks are up to 3 metres (9.8 ft) thick.[55] The total volume of these pyroclastic flows is about 0.06 cubic kilometres (0.014 cu mi).[168]
There is a pronounced morphology characterized by a channel upslope and snouts downslope.[169] Flow surfaces display pronounced fractures with a V profile,[170] which developed a year after the eruption.[171] The pyroclastic flow surfaces subsided after the eruption, with pulses of faster subsidence coinciding with the 1995 Antofagasta earthquake and the 2007 Tocopilla earthquake.[172]
The flows were strongly erosive, extracting rocks and material from the bedrock even far away from the vent.[41] A noticeable erosion occurred in the areas where pyroclastic flows had passed over, forming abrasion surfaces and removing loose detritus from the ground.[173]
These flows took a long time to cool down, in the Quebrada Tumbres they had not cooled down completely in December 1993.[174] Additional surfaces were covered by ash cloud surges, reaching thicknesses of no more than 5 centimetres (2.0 in) on the sides of the pyroclastic flows.[55] In some parts of the edifice, ejecta formed layers thick enough to undergo landsliding.[175]
The ash from the volcano was carried by western wind towards Argentina and the Atlantic Ocean.[156] Ash fall in Tucuman and Santiago del Estero was intense enough that traffic ground to a halt.[176] Tephra fall from this eruption was recorded in Argentina including the capital Buenos Aires 1,500 kilometres (930 mi) away, Brazil, Paraguay and Uruguay.[161] Ash from this eruption was identified in ice cores from Illimani.[177] Over 0.1 millimetres (0.0039 in) of ash fell over a surface area of over 850,000 square kilometres (330,000 sq mi).[25] Larger particles fell closer to the volcano, while smaller particles were carried to larger distances.[178]
This eruption is the most significant eruption of Lascar in the last 9,000 years, with a volcanic explosivity index of 4.[28][113] It caused noticeable changes in the morphology of the volcano, including the formation of a new fracture along the summit craters.[179] The eruption released about 400,000 tonnes (390,000 long tons; 440,000 short tons) of sulfur dioxide, about half the quantity released by the 1980 eruption of Mount St. Helens.[180] This amount of sulfur dioxide was sufficient to cause a noticeable increase in atmospheric opacity.[181] The Quebrada Tumbre was blocked and its water chemistry noticeably altered by the eruption.[111] About 900,000 tonnes (890,000 long tons; 990,000 short tons) of gypsum were deposited in the drainages around the volcano, forming a significant supply of sulfur in the region.[182]
The eruption led to water pollution issues in the region, including increases in cadmium, copper and lead content in local rivers.[183] An increase of mercury from the eruption was detected as far as Laguna del Plata, Argentina.[184] The 1993 eruption was followed by a significant increase in fluorine content of plants covered by the ash. Limits on concentrations of other elements in water were exceeded, although only temporarily.[111]
Post-1993 activity
The eruption record at Lascar becomes more irregular after the 1993 eruption.[185] During April 1993 a new lava dome formed in the crater, reaching a diameter of 380 metres (1,250 ft). It started to shrink again by May. On 17 December 1993 another explosion created an eruption column 8–10 kilometres (5.0–6.2 mi) high. By 28 December the dome had subsided completely in the centre, leaving only its margins. Subsequently, a number of fumaroles were active around the crater.[161] Explosive eruptions accompanied by the formation of eruption columns reaching heights of several 1 kilometre (0.62 mi), sometimes leading to ash fall in Jujuy, Argentina, occurred on 27 February 1994, July 1994, November 1994, March 1995, 10 May 1995, 20 July 1995, and 18 October 1996.[150] During the July 1995 eruption, subsidence was noted inside the central crater from satellite images.[186] The collapse structures during this activity were enlarged compared to these of previous activity, possibly because the April 1993 eruption had emptied part of the system.[96] Otherwise, activity between 1993 and 2000 was not accompanied by deformation of the edifice.[187][188]
An eruption in July 2000 was seen from Chuquicamata and the noise was audible as far as San Antonio de los Cobres 160 kilometres (99 mi) away. The eruption lasted for two hours and formed a 10–11 kilometres (6.2–6.8 mi) high eruption column.[189] An ash plume was carried 660 kilometres (410 mi) east.[52] Three eruptions in October 2002 formed ash columns that rose 500–2,500 metres (1,600–8,200 ft), while an explosion in December 2003 created a column 400–500 metres (1,300–1,600 ft) high.[190] No lava domes were recorded during that period in the crater.[191]

Further activity occurred in May 2005 with a 8–10 kilometres (5.0–6.2 mi) high ash cloud and in April 2006. [52] An eruption commenced at 11:35 local time, 18 April 2006.[192] This explosion was strong enough to rattle windows in the school at Talabre.[193] The 18 April eruption was seen from the El Abra copper mine 220 kilometres (140 mi) away and resulted in ash fall north-northeast from the volcano. Four eruptions occurred at 15:20, 17:22, 19:00, and 21:00 UTC forming eruption columns reaching altitudes of 10 kilometres (6.2 mi). The next day, additional explosions occurred at 15:04, 15:05, and 17:39 UTC, with a maximum column height of 7 kilometres (4.3 mi).[194] A video taken by the Chilean Air Force on 20 April showed a 50 metres (160 ft) wide pit in the floor of the main crater.[195] The following days additional explosions generated columns up to 3 kilometres (1.9 mi) high, with little ash production.[196] The eruption ended around 15:32 on 20 April,[193] although some explosions occurred in the following days.[197] Other eruptions were recorded in November 2006 and July 2007.[190]
Weak eruptions, characterized by earthquakes and the release of plumes, occurred in February–March 2012 and March–April 2013.[105] Between April and June 2013 glow was observed at the summit, accompanied by the occasional release of gray clouds. Glowing was also reported in October and November 2013.[198] The last eruption in 30 October 2015 created a 2,500 metres (8,200 ft) high column of ash that prompted a raise in the local volcano alert level.[2]
Monitoring and threats
Because of the volcano's remote location, much information on its activity comes from remote sensing.[162] In addition, occasional reconnaissance flights, seismographic monitoring, and infrequent visits to the volcano occur.[113] The Observatorio Volcanológico de los Andes del Sur in Temuco also employs webcams to watch Lascar.[105]
Lascar's activity has been monitored by Thematic Mapper, which has been used to monitor volcanic activity since 1985, when hot spots were observed on Lascar.[199] The eruptions of April 1993 and September 1986 were both preceded by a reduction of thermal radiation observed by Thematic Mapper.[113]
Seismic activity occurs at Lascar. Research has indicated peculiar patterns including so called "rapid-fire" events on a background of continuous activity.[200] Harmonic tremor has been recorded at Lascar,[142] perhaps caused by a hydrothermal system.[74] Such tremors may be produced by the movement of liquid materials in the volcano.[201] A number of earthquakes were recorded in early February 2012.[105] Between January 2014 and June 2016, about 2–4 volcano-tectonic earthquakes per month were recorded. Long-period earthquakes with magnitudes not exceeding 1.3 were also recorded, a maximum noted in May 2015 with 209 events.[2]
Explosive eruptions and ash falls are the major threat from Lascar volcano.[139] The towns of Tumbres and Talabre may be affected by pyroclastic flows, and ash falls can occur east of the volcano.[105] Such ash falls could potentially affect the towns of San Pedro de Atacama, Talabre, Toconao as well as the Llano de Chajnantor Observatory and the San Pedro de Atacama-Paso de Jama-Jujuy international road.[202] Past eruptions caused ash fall in Argentina and disruption of air travel.[203] The town of Talabre was moved for safety reasons.[47] Sector collapse and lahars did occur in the past, but are unlikely to be present-day hazards.[139] The National Geology and Mining Service of Chile publishes a "volcano alert level" for Lascar.[204]
Heavy metal exposure is a problem for the region. High quantities of arsenic have been observed in local crops.[205] Thallium from the volcano is a pollution hazard in the Talabre area.[206] High nickel concentrations in crops from Talabre appear to depend on volcanic activity as well.[33]
See also
Notes
- 1 2 3 4 "Láscar". Global Volcanism Program. Smithsonian Institution. Retrieved 8 December 2016.
- 1 2 3 Global Volcanism Program, 2016. Report on Lascar (Chile). In: Venzke, E (ed.), Bulletin of the Global Volcanism Network, 41:7. Smithsonian Institution.
- 1 2 3 Casertano & Barozzi 2015, p. 309.
- 1 2 Casertano & Barozzi 2015, p. 312.
- ↑ Le Paige, Gustavo (1978-01-01). "Vestigios arqueológicos incaicos en las cumbres de la zona atacameña". Estudios Atacameños (6): 36–52.
- ↑ Moyano, Ricardo (26 July 2011). "Sub-tropical astronomy in the southern Andes: the ceque system in Socaire, Atacama, northern Chile". Proceedings of the International Astronomical Union. 7 (S278): 99. doi:10.1017/S1743921311012518.
- ↑ Patricia Erfurt-Cooper (9 August 2014). Volcanic Tourist Destinations. Springer Science & Business Media. p. 4. ISBN 978-3-642-16191-9.
- 1 2 3 Matthews, Jones & Gardeweg 1994, p. 401.
- 1 2 Tilling 2009, p. 126.
- ↑ Tilling 2009, p. 127.
- ↑ Nur & Ben-Avraham 1981, p. 730.
- ↑ Nur & Ben-Avraham 1981, p. 731.
- ↑ Nur & Ben-Avraham 1981, p. 738.
- 1 2 3 4 5 Menard et al. 2014, p. 53.
- ↑ Díaz, Brasse & Ticona 2012, p. 21.
- ↑ Díaz, Brasse & Ticona 2012, p. 22.
- ↑ Tilling 2009, p. 128.
- 1 2 3 4 5 6 Calder, Sparks & Gardeweg 2000, p. 202.
- ↑ Mather et al. 2004, p. 1.
- 1 2 3 Matthews et al. 1996, p. 510.
- 1 2 Francis & Rothery 1987, p. 614.
- ↑ Matthews, Jones & Gardeweg 1994, p. 401,403.
- 1 2 3 4 Glaze et al. 1989, p. 151.
- ↑ Francis & Rothery 1987, p. 616.
- 1 2 3 4 Tassi et al. 2008, p. 172.
- ↑ Pritchard & Simons 2004, p. 2.
- 1 2 Glaze et al. 1989, p. 149.
- 1 2 3 4 5 6 Denniss et al. 1998, p. 802.
- 1 2 3 4 5 6 7 8 Francis & Rothery 1987, p. 615.
- 1 2 3 4 5 6 7 8 9 10 Gardeweg, Sparks & Matthews 1998, p. 92.
- 1 2 Gardeweg, Sparks & Matthews 1998, p. 90.
- ↑ Déruelle et al. 1996, p. 191.
- 1 2 Stegen, Susana; Queirolo, Fabrizio; Carrasco, Carmen; Ostaoczuk, Peter; Sschwuger, Milan J. (September 2002). "Concentrations of Ni and Co in crop plants cultivated in northern Chile". Boletín de la Sociedad Chilena de Química. 47 (3). doi:10.4067/S0366-16442002000300012.
- 1 2 3 National Imagery and Mapping Agency. "Salar de Atacama, Chile" (Map). Latin America, Joint Operations Graphic (jpg) (1 ed.). 1:250,000. 1501.
- 1 2 3 4 5 6 Calder, Sparks & Gardeweg 2000, p. 204.
- ↑ Demergasso, Cecilia; Dorador, Cristina; Meneses, Daniela; Blamey, Jenny; Cabrol, Nathalie; Escudero, Lorena; Chong, Guillermo (June 2010). "Prokaryotic diversity pattern in high-altitude ecosystems of the Chilean Altiplano". Journal of Geophysical Research: Biogeosciences. 115 (G2): 11. doi:10.1029/2008JG000836.
- ↑ Gardeweg, Sparks & Matthews 1998, p. 91.
- ↑ Cabrol et al. 2009, p. 3.
- 1 2 3 4 Calder, Sparks & Gardeweg 2000, p. 203.
- 1 2 3 4 5 Donoso, Aguilera & Medina 2005, p. 231.
- 1 2 Calder, Sparks & Gardeweg 2000, p. 223.
- ↑ Díaz, Brasse & Ticona 2012, p. 27.
- 1 2 3 4 5 6 7 8 9 Calder, Sparks & Gardeweg 2000, p. 205.
- 1 2 Donoso, Aguilera & Medina 2005, p. 230.
- ↑ Donoso, Aguilera & Medina 2005, p. 233.
- 1 2 Matthews, Jones & Gardeweg 1994, p. 402.
- 1 2 Fernández, Álvarez & Salinas 2011, p. 748.
- 1 2 3 4 5 6 7 8 9 10 11 Matthews, Gardeweg & Sparks 1997, p. 73.
- 1 2 3 4 5 6 7 8 9 Matthews, Jones & Gardeweg 1994, p. 403.
- ↑ Gardeweg, Sparks & Matthews 1998, p. 89.
- 1 2 3 4 5 6 7 Matthews, Gardeweg & Sparks 1997, p. 74.
- 1 2 3 4 5 Tassi et al. 2008, p. 173.
- ↑ Casertano & Barozzi 2015, p. 311.
- 1 2 3 Zellmer et al. 2014, p. 189.
- 1 2 3 4 Sparks et al. 1997, p. 559.
- ↑ Matthews, Jones & Gardeweg 1994, pp. 409–411.
- ↑ Matthews, Jones & Gardeweg 1994, p. 404.
- 1 2 3 4 5 6 Matthews, Jones & Gardeweg 1994, p. 405.
- ↑ Sparks et al. 1997, p. 562.
- ↑ Sparks et al. 1997, p. 560.
- ↑ Matthews, S.; Vita-Finzi, C. (1993-01-01). "Neotectonics at Laguna Lejia, Atacama desert, Northern Chile": 115.
- 1 2 3 4 Matthews, Jones & Gardeweg 1994, p. 428.
- ↑ Casertano & Barozzi 2015, p. 308.
- ↑ Matthews, Jones & Gardeweg 1994, pp. 406–407.
- 1 2 Matthews, Gardeweg & Sparks 1997, p. 72.
- ↑ Matthews, Jones & Gardeweg 1994, p. 414.
- ↑ Matthews, Jones & Gardeweg 1994, p. 421.
- ↑ Matthews, Jones & Gardeweg 1994, p. 422.
- ↑ Matthews, Sparks & Gardeweg 1999, pp. 1892–1893.
- 1 2 3 Matthews, Jones & Gardeweg 1994, p. 411.
- ↑ Matthews, Jones & Gardeweg 1994, p. 406.
- 1 2 3 Gardeweg, Sparks & Matthews 1998, p. 102.
- 1 2 González et al. 2015, p. 288.
- 1 2 Pritchard & Simons 2004, p. 26.
- 1 2 3 4 5 Matthews, Sparks & Gardeweg 1999, p. 1893.
- 1 2 Matthews, Jones & Gardeweg 1994, p. 412.
- ↑ Matthews et al. 1996, p. 516.
- ↑ Laznicka, Peter (2010-01-01). Giant Metallic Deposits. Springer Berlin Heidelberg. pp. 109–168. ISBN 978-3-642-12404-4. doi:10.1007/978-3-642-12405-1_6.
- 1 2 Matthews, Jones & Gardeweg 1994, p. 413.
- 1 2 Matthews et al. 1996, p. 528.
- ↑ Matthews et al. 1996, p. 513.
- ↑ Tassi et al. 2008, p. 173,175.
- 1 2 3 Tassi et al. 2008, p. 175.
- ↑ Menard et al. 2014, p. 55.
- ↑ Mather et al. 2004, p. 7.
- 1 2 Mather et al. 2004, p. 18.
- 1 2 Menard et al. 2014, p. 52.
- 1 2 Menard et al. 2014, p. 58.
- ↑ Fatima, Hashmi; Upadhyaya, H. C.; Tripathi, S. N.; Sharma, O. P.; Yu, Fangqun (3 May 2011). "On radiative forcing of sulphate aerosol produced from ion-promoted nucleation mechanisms in an atmospheric global model". Meteorology and Atmospheric Physics. 112 (3–4): 108. doi:10.1007/s00703-011-0138-8.
- ↑ Lucas, D. D.; Akimoto, H. (4 June 2007). "Contributions of anthropogenic and natural sources of sulfur to SO2, H2SO4(g) and nanoparticle formation". Atmospheric Chemistry and Physics Discussions. 7 (3): 7693–7694. doi:10.5194/acpd-7-7679-2007.
- ↑ Roberta L. Rudnick (2005). The Crust. Gulf Professional Publishing. p. 146. ISBN 978-0-08-044847-3.
- ↑ Menard et al. 2014, p. 63.
- 1 2 Mather et al. 2004, p. 8.
- ↑ Menard et al. 2014, p. 59.
- 1 2 3 4 5 Menard et al. 2014, p. 60.
- 1 2 3 Matthews, Gardeweg & Sparks 1997, p. 81.
- ↑ Matthews, Jones & Gardeweg 1994, p. 426.
- ↑ Tassi et al. 2008, p. 176,178.
- ↑ Matthews, Jones & Gardeweg 1994, pp. 428–429.
- ↑ Risacher & Alonso 2001, p. 327.
- ↑ Tassi et al. 2008, p. 176.
- ↑ Inostroza, M.; González, C.; Aguilera, F. (2014-12-01). "Eruptive and magma circulation patterns determined by satellite imagery: The case of Lascar volcano, Northern Chile". AGU Fall Meeting Abstracts. 41.
- ↑ Díaz, Brasse & Ticona 2012, p. 28.
- ↑ Tassi, F.; Aguilera, F.; Medina, E.; Vaselli, O.; Tedesco, D.; Poreda, R.J. (January 2007). "First geochemical survey of fumarolic gases from Lascar volcano (Central Andes, Chile)" (PDF). Geophysical Research Abstracts. 9. Retrieved 11 December 2016.
- 1 2 3 4 5 6 Global Volcanism Program, 2013. Report on Lascar (Chile). In: Venzke, E (ed.), Bulletin of the Global Volcanism Network, 38:7. Smithsonian Institution.
- 1 2 Risacher & Alonso 2001, p. 321.
- ↑ Tuffen, H. (19 April 2010). "How will melting of ice affect volcanic hazards in the twenty-first century?". Philosophical Transactions of the Royal Society A: Mathematical, Physical and Engineering Sciences. 368 (1919): 2544. doi:10.1098/rsta.2010.0063.
- ↑ Gardeweg, Sparks & Matthews 1998, pp. 101–102.
- ↑ Ram Bali Singh (1992). Dynamics of Mountain Geosystems. APH Publishing. p. 165. ISBN 978-81-7024-472-1.
- ↑ Cabrol et al. 2009, p. 3,4.
- 1 2 3 Gardeweg & Medina 1994, p. 303.
- 1 2 3 4 5 6 Matthews, Sparks & Gardeweg 1999, p. 1892.
- 1 2 3 4 5 6 7 Wooster & Rothery 1997, p. 567.
- ↑ Samaniego, Pablo; Rivera, Marco; Mariño, Jersy; Guillou, Hervé; Liorzou, Céline; Zerathe, Swann; Delgado, Rosmery; Valderrama, Patricio; Scao, Vincent (September 2016). "The eruptive chronology of the Ampato–Sabancaya volcanic complex (Southern Peru)". Journal of Volcanology and Geothermal Research. 323: 110–128. doi:10.1016/j.jvolgeores.2016.04.038.
- 1 2 3 González et al. 2015, p. 278.
- ↑ Gardeweg, Sparks & Matthews 1998, p. 95.
- 1 2 3 Gardeweg, Sparks & Matthews 1998, p. 94.
- 1 2 3 Gardeweg, Sparks & Matthews 1998, p. 96.
- ↑ Matthews, Sparks & Gardeweg 1999, p. 1913.
- ↑ Matthews, Sparks & Gardeweg 1999, p. 1897.
- 1 2 Matthews, Sparks & Gardeweg 1999, p. 1900.
- 1 2 3 Matthews, Sparks & Gardeweg 1999, p. 1917.
- ↑ Matthews, Sparks & Gardeweg 1999, p. 1895.
- 1 2 3 Gardeweg, Sparks & Matthews 1998, p. 97.
- ↑ Matthews, Sparks & Gardeweg 1999, p. 1901.
- 1 2 3 Calder, Sparks & Gardeweg 2000, p. 207.
- ↑ Matthews, Sparks & Gardeweg 1999, p. 1903.
- ↑ Matthews, Sparks & Gardeweg 1999, p. 1914.
- ↑ Pritchard & Simons 2004, p. 28.
- 1 2 Matthews, Sparks & Gardeweg 1999, p. 1915.
- ↑ Calder, Sparks & Gardeweg 2000, p. 211.
- ↑ Gardeweg, Sparks & Matthews 1998, p. 98.
- ↑ Calder, Sparks & Gardeweg 2000, p. 210.
- 1 2 Matthews, Jones & Gardeweg 1994, p. 409.
- 1 2 Gardeweg, Sparks & Matthews 1998, p. 99.
- ↑ Gardeweg, Sparks & Matthews 1998, p. 94,97.
- 1 2 3 4 5 6 Gardeweg, Sparks & Matthews 1998, p. 100.
- ↑ Gardeweg, Sparks & Matthews 1998, p. 99,100.
- 1 2 3 Gardeweg, Sparks & Matthews 1998, p. 103.
- 1 2 3 4 5 6 "Lascar, Eruptive History". Global Volcanism Program. Smithsonian Institution. Retrieved 11 December 2016.
- ↑ Gardeweg, Sparks & Matthews 1998, p. 101.
- 1 2 Hellweg 1999, p. 452.
- ↑ Casertano & Barozzi 2015, p. 313.
- ↑ Rudolph, William E. (October 1952). "Sulphur in Chile". Geographical Review. 42 (4): 568. doi:10.2307/211839.
- 1 2 Francis & Rothery 1987, p. 617.
- 1 2 Glaze et al. 1989, p. 152.
- ↑ Glaze et al. 1989, p. 153.
- 1 2 Matthews, Gardeweg & Sparks 1997, p. 75.
- ↑ Wooster & Rothery 1997, p. 568.
- 1 2 Matthews, Gardeweg & Sparks 1997, p. 77.
- ↑ Wright, Robert; Flynn, Luke P. (2003). "On the retrieval of lava-flow surface temperatures from infrared satellite data". Geology. 31 (10): 893. doi:10.1130/G19645.1.
- ↑ Mather et al. 2004, p. 2.
- ↑ Wooster 2001, p. 848.
- 1 2 Gardeweg & Medina 1994, p. 299.
- ↑ Gardeweg & Medina 1994, p. 300.
- 1 2 3 4 Déruelle et al. 1996, p. 192.
- 1 2 Calder, Sparks & Gardeweg 2000, p. 217.
- 1 2 3 Sparks et al. 1997, p. 558.
- ↑ Déruelle et al. 1996, p. 194.
- ↑ Gardeweg & Medina 1994, pp. 299–300.
- 1 2 3 Matthews, Gardeweg & Sparks 1997, p. 76.
- 1 2 Harris et al. 1997, p. 55.
- ↑ Calder, Sparks & Gardeweg 2000, p. 219.
- ↑ Harris et al. 1997, p. 56.
- ↑ Jessop et al. 2012, p. 82.
- ↑ Denniss et al. 1998, p. 808.
- ↑ Calder, Sparks & Gardeweg 2000, p. 221.
- ↑ Calder, Sparks & Gardeweg 2000, p. 228.
- ↑ Jessop et al. 2012, p. 94.
- ↑ Whelley et al. 2011, p. 514.
- ↑ Whelley et al. 2011, p. 515.
- ↑ Whelley et al. 2011, p. 521,522.
- ↑ Sparks et al. 1997, p. 557.
- ↑ Wooster 2001, p. 849.
- ↑ Gardeweg & Medina 1994, p. 302.
- ↑ Gardeweg & Medina 1994, p. 301.
- ↑ Delmonte, B.; Andersson, P.S.; Schöberg, H.; Hansson, M.; Petit, J.R.; Delmas, R.; Gaiero, D.M.; Maggi, V.; Frezzotti, M. (January 2010). "Geographic provenance of aeolian dust in East Antarctica during Pleistocene glaciations: preliminary results from Talos Dome and comparison with East Antarctic and new Andean ice core data". Quaternary Science Reviews. 29 (1–2): 261. doi:10.1016/j.quascirev.2009.05.010.
- ↑ Fernández, Álvarez & Salinas 2011, p. 749.
- ↑ Pavez et al. 2006, p. 308.
- ↑ Deshler, Terry; Anderson-Sprecher, Richard; Jäger, Horst; Barnes, John; Hofmann, David J.; Clemesha, Barclay; Simonich, Dale; Osborn, M.; Grainger, R. G.; Godin-Beekmann, Sophie (2006). "Trends in the nonvolcanic component of stratospheric aerosol over the period 1971–2004". Journal of Geophysical Research. 111 (D1): 2. doi:10.1029/2005JD006089.
- ↑ Crowley, T. J.; Unterman, M. B. (23 May 2013). "Technical details concerning development of a 1200 yr proxy index for global volcanism". Earth System Science Data. 5 (1): 189. doi:10.5194/essd-5-187-2013.
- ↑ Risacher & Alonso 2001, p. 333.
- ↑ Queirolo, F (8 June 2000). "Total arsenic, lead, cadmium, copper, and zinc in some salt rivers in the northern Andes of Antofagasta, Chile". The Science of The Total Environment. 255 (1–3): 90. doi:10.1016/S0048-9697(00)00451-4.
- ↑ Stupar, Yohana Vanesa; García, María Gabriela; Schäfer, Jörg; Schmidt, Sabine; Piovano, Eduardo; Blanc, Gérard; Huneau, Frédéric; Le Coustumer, Phillipe (2014-04-01). "Identificación de fases portadoras y flujos de mercurio en el registro sedimentario de la Laguna del Plata, región central de Argentina". Revista mexicana de ciencias geológicas. 31 (1): 104–115. ISSN 1026-8774.
- ↑ González et al. 2015, p. 277.
- ↑ Pavez et al. 2006, p. 315.
- ↑ Pavez et al. 2006, p. 313.
- ↑ Pritchard & Simons 2004, p. 10.
- ↑ González et al. 2015, p. 278,279.
- 1 2 González et al. 2015, p. 279.
- ↑ González et al. 2015, p. 285.
- ↑ Clavero, Naranjo & Cayupi 2006, p. 435.
- 1 2 Clavero, Naranjo & Cayupi 2006, p. 436.
- ↑ Aguilera et al. 2006, p. 394.
- ↑ Clavero, Naranjo & Cayupi 2006, pp. 436–437.
- ↑ Aguilera et al. 2006, p. 395.
- ↑ Clavero, Naranjo & Cayupi 2006, p. 437.
- ↑ Global Volcanism Program, 2015. Report on Lascar (Chile). In: Venzke, E (ed.), Bulletin of the Global Volcanism Network, 40:6. Smithsonian Institution.
- ↑ Harris et al. 1997, p. 49.
- ↑ Asch et al. 1996, p. 282.
- ↑ Hellweg 1999, p. 463.
- ↑ Aguilera et al. 2006, p. 396.
- ↑ Perucca, Laura P.; Moreiras, Stella M. (2009). "Seismic and Volcanic Hazards in Argentina". Developments in Earth Surface Processes. 13: 288–289. doi:10.1016/S0928-2025(08)10014-1.
- ↑ "Red Nacional de Vigilancia Volcánica de Chile". sernageomin.cl (in Spanish). National Geology and Mining Service. Retrieved 11 December 2016.
- ↑ Queirolo, F (8 June 2000). "Total arsenic, lead, and cadmium levels in vegetables cultivated at the Andean villages of northern Chile". The Science of The Total Environment. 255 (1–3): 75–84. doi:10.1016/S0048-9697(00)00450-2.
- ↑ Queirolo, Fabrizio; Stegen, Susana; Contreras-Ortega, Carlos; Ostapczuk, Peter; Queirolo, Alessandro; Paredes, Betty (2009-12-01). "Thallium Levels and Bioaccumulation in Environmental Samples of Northern Chile: Human Health Risks". Journal of the Chilean Chemical Society. 54 (4): 464–469. ISSN 0717-9707. doi:10.4067/S0717-97072009000400031.
Sources
- Aguilera, F.; Viramonte, J.; Medina, E.; Guzmán, K.; Becchio, R.; Delgado, H.; Arnosio, M. (August 2006). "Recent eruptive activity from Lascar volcano (2006)" (PDF). SERNAGEOMIN. Antofagasta: 11th Chilean Geological Congress. Retrieved 5 December 2016.
- Asch, G.; Wylegalla, K.; Hellweg, M.; Seidl, D.; Rademacher, H. (March 1996). "Observations of rapid-fire event tremor at Lascar volcano, Chile". Annals of Geophysics. 39 (2): 273–282. doi:10.4401/ag-3972.
- Calder, E.S.; Sparks, R.S.J.; Gardeweg, M.C. (December 2000). "Erosion, transport and segregation of pumice and lithic clasts in pyroclastic flows inferred from ignimbrite at Lascar Volcano, Chile". Journal of Volcanology and Geothermal Research. 104 (1–4): 201–235. doi:10.1016/S0377-0273(00)00207-9.
- Casertano, L.; Barozzi, R. (2015). "Informe sobre el sistema volcánico de Lascar" (PDF). Anales de la Facultad de Ciencias Físicas y Matemáticas (in Spanish). Universidad de Chile. 18 (18): 303–315. Retrieved 7 December 2016.
- Denniss, A. M.; Harris, A. J. L.; Rothery, D. A.; Francis, P. W.; Carlton, R. W. (January 1998). "Satellite observations of the April 1993 eruption of Lascar volcano". International Journal of Remote Sensing. 19 (5): 801–821. doi:10.1080/014311698215739.
- Déruelle, Bernard; Oscar Figueroa, A.; Eduardo Medina, T.; Jose Viramonte, G.; Mario Maragaño, C. (March 1996). "Petrology of pumices of April 1993 eruption of Lascar (Atacama, Chile)". Terra Nova. 8 (2): 191–199. doi:10.1111/j.1365-3121.1996.tb00744.x.
- Cabrol, Nathalie A.; Grin, Edmond A.; Chong, Guillermo; Minkley, Edwin; Hock, Andrew N.; Yu, Youngseob; Bebout, Leslie; Fleming, Erich; Häder, Donat P.; Demergasso, Cecilia; Gibson, John; Escudero, Lorena; Dorador, Cristina; Lim, Darlene; Woosley, Clayton; Morris, Robert L.; Tambley, Cristian; Gaete, Victor; Galvez, Matthieu E.; Smith, Eric; Uskin-Peate, Ingrid; Salazar, Carlos; Dawidowicz, G.; Majerowicz, J. (June 2009). "The High-Lakes Project". Journal of Geophysical Research: Biogeosciences. 114 (G2): 1–20. doi:10.1029/2008JG000818.
- Clavero, Jorge; Naranjo, José A; Cayupi, Juan (August 2006). "El ciclo eruptivo del 18 al 25 de Abril de 2006 del volcan Lascar, Andes Centrales" (PDF). biblioserver.sernageomin.cl (in Spanish). Antofagasta: 9th Chilean Geological Congress. pp. 435–438. Retrieved 11 December 2016.
- Díaz, Daniel; Brasse, Heinrich; Ticona, Faustino (March 2012). "Conductivity distribution beneath Lascar volcano (Northern Chile) and the Puna, inferred from magnetotelluric data". Journal of Volcanology and Geothermal Research. 217–218: 21–29. doi:10.1016/j.jvolgeores.2011.12.007.
- Donoso, C.L.; Aguilera, F.A.; Medina, Eduardo T. (2005-01-01). "Geology and petrology of the Corona and Cerros de Saltar domes near the Lascar volcano system, northern Chile".
- Fernández, Luis; Álvarez, Gabriel; Salinas, Renato (25 November 2011). "Impact area determination of pyroclastic deposits in Lascar volcano, eruptive process in the year 1993". Natural Hazards. 60 (2): 747–760. doi:10.1007/s11069-011-0048-6.
- Francis, P. W.; Rothery, D. A. (1987). "Using the Landsat Thematic Mapper to detect and monitor active volcanoes: An example from Lascar volcano, northern Chile". Geology. 15 (7): 614. doi:10.1130/0091-7613(1987)15<614:UTLTMT>2.0.CO;2.
- Gardeweg, P. Moyra; Medina, Eduardo (1994). "La erupcion subpliniana del 19-20 de Abril de 1993 del volcan Lascar, N de Chile" (PDF). 7th Chilean Geological Congress (in Spanish). University of Concepcion. Retrieved 11 December 2016.
- Gardeweg, M. C.; Sparks, R. S. J.; Matthews, S. J. (1 February 1998). "Evolution of Lascar Volcano, Northern Chile". Journal of the Geological Society. 155 (1): 89–104. doi:10.1144/gsjgs.155.1.0089.
- Glaze, L. S.; Francis, P. W.; Self, S.; Rothery, D. A. (May 1989). "The 16 September 1986 eruption of Lascar volcano, north Chile: Satellite investigations". Bulletin of Volcanology. 51 (3): 149–160. doi:10.1007/BF01067952.
- González, C.; Inostroza, M.; Aguilera, F.; González, R.; Viramonte, J.; Menzies, A. (August 2015). "Heat and mass flux measurements using Landsat images from the 2000–2004 period, Lascar volcano, northern Chile". Journal of Volcanology and Geothermal Research. 301: 277–292. doi:10.1016/j.jvolgeores.2015.05.009.
- Harris, Andrew J. L.; Butterworth, Anna L.; Carlton, Richard W.; Downey, Ian; Miller, Peter; Navarro, Pedro; Rothery, David A. (23 September 1997). "Low-cost volcano surveillance from space: case studies from Etna, Krafla, Cerro Negro, Fogo, Lascar and Erebus". Bulletin of Volcanology. 59 (1): 49–64. doi:10.1007/s004450050174.
- Hellweg, Margaret (June 1999). "Listening carefully: unique observations of harmonic tremor at Lascar volcano, Chile". Annals of Geophysics. 42 (3): 451–464. doi:10.4401/ag-3729.
- Jessop, D.E.; Kelfoun, K.; Labazuy, P.; Mangeney, A.; Roche, O.; Tillier, J.-L.; Trouillet, M.; Thibault, G. (November 2012). "LiDAR derived morphology of the 1993 Lascar pyroclastic flow deposits, and implication for flow dynamics and rheology". Journal of Volcanology and Geothermal Research. 245–246: 81–97. doi:10.1016/j.jvolgeores.2012.06.030.
- Mather, T. A.; Tsanev, V. I.; Pyle, D. M.; McGonigle, A. J. S.; Oppenheimer, C.; Allen, A. G. (16 November 2004). "Characterization and evolution of tropospheric plumes from Lascar and Villarrica volcanoes, Chile". Journal of Geophysical Research: Atmospheres. 109 (D21): n/a–n/a. doi:10.1029/2004JD004934.
- Matthews, S. J.; Jones, A. P.; Gardeweg, M. C. (1 April 1994). "Lascar Volcano, Northern Chile; Evidence for Steady-State Disequilibrium". Journal of Petrology. 35 (2): 401–432. doi:10.1093/petrology/35.2.401.
- Matthews, S. J.; Marquillas, R. A.; Kemp, A. J.; Grange, F. K.; Gardeweg, M. C. (July 1996). "Active skarn formation beneath Lascar Volcano, northern Chile: a petrographic and geochemical study of xenoliths in eruption products". Journal of Metamorphic Geology. 14 (4): 509–530. doi:10.1046/j.1525-1314.1996.00359.x.
- Matthews, S. J.; Sparks, R. S. J.; Gardeweg, M. C. (1 December 1999). "The Piedras Grandes-Soncor Eruptions, Lascar Volcano, Chile; Evolution of a Zoned Magma Chamber in the Central Andean Upper Crust". Journal of Petrology. 40 (12): 1891–1919. doi:10.1093/petroj/40.12.1891.
- Matthews, Stephen J.; Gardeweg, Moyra C.; Sparks, R. Stephen J. (23 September 1997). "The 1984 to 1996 cyclic activity of Lascar Volcano, northern Chile: cycles of dome growth, dome subsidence, degassing and explosive eruptions". Bulletin of Volcanology. 59 (1): 72–82. doi:10.1007/s004450050176.
- Menard, G.; Moune, S.; Vlastélic, I.; Aguilera, F.; Valade, S.; Bontemps, M.; González, R. (October 2014). "Gas and aerosol emissions from Lascar volcano (Northern Chile): Insights into the origin of gases and their links with the volcanic activity". Journal of Volcanology and Geothermal Research. 287: 51–67. doi:10.1016/j.jvolgeores.2014.09.004.
- Nur, Amos; Ben-Avraham, Zvi (1981). "Volcanic gaps and the consumption of aseismic ridges in South America". GSA Memoirs. 154: 729–740. doi:10.1130/MEM154-p729.
- Pavez, A.; Remy, D.; Bonvalot, S.; Diament, M.; Gabalda, G.; Froger, J-L.; Julien, P.; Legrand, D.; Moisset, D. (February 2006). "Insight into ground deformations at Lascar volcano (Chile) from SAR interferometry, photogrammetry and GPS data: Implications on volcano dynamics and future space monitoring". Remote Sensing of Environment. 100 (3): 307–320. doi:10.1016/j.rse.2005.10.013.
- Pritchard, M. E.; Simons, M. (February 2004). "An InSAR-based survey of volcanic deformation in the central Andes". Geochemistry, Geophysics, Geosystems. 5 (2): 1–42. doi:10.1029/2003GC000610.
- Risacher, François; Alonso, Hugo (September 2001). "Geochemistry of ash leachates from the 1993 Lascar eruption, northern Chile. Implication for recycling of ancient evaporites". Journal of Volcanology and Geothermal Research. 109 (4): 319–337. doi:10.1016/S0377-0273(01)00198-6.
- Sparks, R. S. J.; Gardeweg, M. C.; Calder, E. S.; Matthews, S. J. (7 May 1997). "Erosion by pyroclastic flows on Lascar Volcano, Chile". Bulletin of Volcanology. 58 (7): 557–565. doi:10.1007/s004450050162.
- Tassi, F.; Aguilera, F.; Vaselli, O.; Medina, E.; Tedesco, D.; Delgado Huertas, A.; Poreda, R.; Kojima, S. (3 June 2008). "The magmatic- and hydrothermal-dominated fumarolic system at the Active Crater of Lascar volcano, northern Chile". Bulletin of Volcanology. 71 (2): 171–183. doi:10.1007/s00445-008-0216-z.
- Tilling, R. I. (14 December 2009). "Volcanism and associated hazards: the Andean perspective". Advances in Geosciences. 22: 125–137. doi:10.5194/adgeo-22-125-2009.
- Whelley, Patrick L.; Jay, J.; Calder, E. S.; Pritchard, M. E.; Cassidy, N. J.; Alcaraz, S.; Pavez, A. (27 September 2011). "Post-depositional fracturing and subsidence of pumice flow deposits: Lascar Volcano, Chile". Bulletin of Volcanology. 74 (2): 511–531. doi:10.1007/s00445-011-0545-1.
- Wooster, M. J.; Rothery, D. A. (7 May 1997). "Thermal monitoring of Lascar Volcano, Chile, using infrared data from the along-track scanning radiometer: a 1992–1995 time series". Bulletin of Volcanology. 58 (7): 566–579. doi:10.1007/s004450050163.
- Wooster, Martin J. (1 March 2001). "Long-term infrared surveillance of Lascar Volcano: Contrasting activity cycles and cooling pyroclastics". Geophysical Research Letters. 28 (5): 847–850. doi:10.1029/2000GL011904.
- Zellmer, Georg F.; Freymuth, Heye; Cembrano, José M.; Clavero, Jorge E.; Veloso, Eugenio A. E.; Sielfeld, Gerd G. (2014). "Altered mineral uptake into fresh arc magmas: insights from U–Th isotopes of samples from Andean volcanoes under differential crustal stress regimes". Geological Society, London, Special Publications. 385 (1): 185–208. doi:10.1144/SP385.9.
Further reading
- González-Ferrán, Oscar (1995). Volcanes de Chile (in Spanish). Santiago, Chile: Instituto Geográfico Militar. ISBN 956-202-054-1.
External links
![]() |
Wikimedia Commons has media related to Lascar Volcano. |
- Lascar at sernageomin.cl
- Ascención al Volcán Lascar(5590msnm.) at the Wayback Machine (archived 13 July 2011)
- Atacama Photo Gallery - panoramic view into the crater of Lascar
- SI Google Earth Placemarks - Smithsonian Institution Global Volcanism Program: download placemarks with SI Holocene volcano-data.