Heusler compound
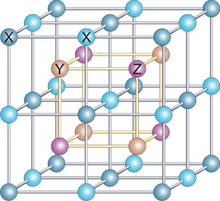

Heusler compounds are magnetic intermetallics with face-centered cubic crystal structure and a composition of XYZ (half-Heuslers) or X2YZ (full-Heuslers), where X and Y are transition metals and Z is in the p-block. Many of these compounds exhibit properties relevant to spintronics, such as magnetoresistance, variations of the Hall effect, ferro-, antiferro-, and ferrimagnetism, half- and semimetallicity, semiconductivity with spin filter ability, superconductivity, and topological insulation. Their magnetism results from a double-exchange mechanism between neighboring magnetic ions. Manganese, which sits at the body centers of the cubic structure, was the magnetic ion in the first Heusler compound discovered. (See the Bethe-Slater curve for more info on why this happens.)
Discovery and properties
The term is named after a German mining engineer and chemist Friedrich Heusler, who studied such a compound in 1903.[1] It contained two parts copper, one part manganese, and one part tin, that is Cu2MnSn, and has the following properties. Its magnetism varies considerably with heat treatment and composition.[2] It has a room-temperature saturation induction of around 8,000 gauss, which exceeds that of the element nickel (around 6100 gauss) but is smaller than that of iron (around 21500 gauss). For early studies see.[1][3][4] In 1934, Bradley and Rogers showed that the room-temperature ferromagnetic phase was a fully ordered structure of the L21 type.[5] This has a primitive cubic lattice of copper atoms with alternate cells body-centered by manganese and aluminium. The lattice parameter is 5.95 Ångströms. The molten alloy has a solidus temperature of about 910 °C. As it is cooled below this temperature, it transforms into disordered, solid, body-centered cubic beta-phase. Below 750 °C, a B2 ordered lattice forms with a primitive cubic copper lattice, which is body-centered by a disordered manganese-aluminium sublattice.[2][6] Cooling below 610 °C causes further ordering of the manganese and aluminium sub-lattice to the L21 form.[2][7] In non-stoichiometric alloys, the temperatures of ordering decrease, and the range of anealing temperatures, where the alloy does not form microprecipitates, becomes smaller than for the stoichiometric material.[8][9][2]
Oxley found a value of 357 °C for the Curie temperature, below which the compound becomes ferromagnetic.[10] Neutron diffraction and other techniques have shown that a magnetic moment of around 3.7 Bohr magnetons resides almost solely on the manganese atoms.[2][11] As these atoms are 4.2 Angstroms apart, the exchange interaction, which aligns the spins, is likely indirect and is mediated through conduction electrons or the aluminium and copper atoms.[10][12]
Electron microscopy studies demonstrated that thermal antiphase boundaries (APBs) form during cooling through the ordering temperatures, as ordered domains nucleate at different centers within the crystal lattice and are often out of step with each other where they meet.[2][6] The anti-phase domains grow as the alloy is annealed. There are two types of APBs corresponding to the B2 and L21 types of ordering. APBs also form between dislocations if the alloy is deformed. At the APB the manganese atoms will be closer than in the bulk of the alloy and, for non-stoichiometric alloys with an excess of copper (e.g. Cu2.2MnAl0.8), an antiferromagnetic layer forms on every thermal APB.[13] These antiferromagnetic layers completely supersede the normal magnetic domain structure and stay with the APBs if they are grown by annealing the alloy. This significantly modifies the magnetic properties of the non-stoichiometric alloy relative to the stoichiometric alloy which has a normal domain structure. Presumably this phenomenon is related to the fact that pure manganese is an antiferromagnet although it is not clear why the effect is not observed in the stoichiometric alloy. Similar effects occur at APBs in the ferromagnetic alloy MnAl at its stoichiometric composition.
Some Heusler compounds also exhibit properties of materials known as ferromagnetic shape-memory alloys. These are generally composed of nickel, manganese and gallium and can change their length by up to 10% in a magnetic field.
List of notable Heusler compounds
- Cu2MnAl, Cu2MnIn, Cu2MnSn
- Ni2MnAl, Ni2MnIn, Ni2MnSn, Ni2MnSb, Ni2MnGa
- Co2MnAl, Co2MnSi, Co2MnGa, Co2MnGe, Co2NiGa
- Pd2MnAl, Pd2MnIn, Pd2MnSn, Pd2MnSb
- Co2FeSi, Co2FeAl
- Fe2VAl
- Mn2VGa, Co2FeGe [14][15]
References
- 1 2 Heusler F. (1903). "Über magnetische Manganlegierungen". Verhandlungen der Deutschen Physikalischen Gesellschaft (in German). 12: 219.
- 1 2 3 4 5 6 Bouchard M. (1970). "Electron metallography and magnetic properties Cu-Mn-Al heusler alloys". Ph.D. Thesis, Imperial College London.
- ↑ Knowlton A.A.; Clifford O.C. (1912). "The Heusler alloys". Trans. Faraday Soc. 8: 195–206. doi:10.1039/TF9120800195.
- ↑ Bozorth, Richard M. (1993). Ferromagnetism. Wiley-VCH. p. 201. ISBN 0-7803-1032-2.
- ↑ Bradley A.J.; Rogers J.W. (1934). "The Crystal Structure of the Heusler Alloys". Proc. Roy. Soc. A144 (852): 340–359. Bibcode:1934RSPSA.144..340B. doi:10.1098/rspa.1934.0053.
- 1 2 Nesterenko Y.H.G.; Osipenko I.A.; Firstov S.A. (1969). Fiz. Metal. Metalloved. (in Russian). 27: 135. Missing or empty
|title=
(help) - ↑ Ohoyama T.; Webster P.J.; Williams K.C. (1968). "The ordering temperature of Cu2MnAl". J. Phys. D. 1 (7): 951. Bibcode:1968JPhD....1..951O. doi:10.1088/0022-3727/1/7/421.
- ↑ West D.R.F.; Lloyd Thomas D. (1956). "The constitution of copper rich alloys of the copper-manganese-aluminum system". Journal of Industrial Metals. 85: 97.
- ↑ Johnston G.B.; Hall E.O. (1968). "Studies on the Heusler alloys—I. Cu2MnAl and associated structures". J. Phys. Chem. Solids. 29 (2): 193, 201. Bibcode:1968JPCS...29..193J. doi:10.1016/0022-3697(68)90062-0.
- 1 2 Oxley D.P.; Tebble R.S.; Williams K.C. (1963). "Heusler Alloys". J. Appl. Phys. 34 (4): 1362. Bibcode:1963JAP....34.1362O. doi:10.1063/1.1729511.
- ↑ Endo K.; Ohoyama T.; Kimura R. (1964). "On the Magnetic Moment of Mn in Aluminum Heusler Alloy". J. Phys. Soc. Japan. 19 (8): 1494–1495. Bibcode:1964JPSJ...19.1494E. doi:10.1143/JPSJ.19.1494.
- ↑ Geldart D.J.W.; Ganguly P. (1970). "Hyperfine Fields and Curie Temperatures of the Heusler Alloys Cu2MnAl, Cu2MnIn, and Cu2MnSn". Physical Review. B1 (7): 3101. Bibcode:1970PhRvB...1.3101G. doi:10.1103/PhysRevB.1.3101.
- ↑ Lapworth A.J.; Jakubovics J.P. (1974). "Effect of antiphase boundaries on the magnetic properties of Cu-Mn-Al Heusler alloys". Phil. Mag. 29 (2): 253. Bibcode:1974PMag...29..253L. doi:10.1080/14786437408213271.
- ↑ . doi:10.1016/j.jmmm.2008.003. Missing or empty
|title=
(help) - ↑ Kumar, K. Ramesh; Bharathi, K. Kamala; Chelvane, J. Arout; Venkatesh, S.; Markandeyulu, G.; Harishkumar, N. (2009). "First-Principles Calculation and Experimental Investigations on Full-Heusler Alloy Co2FeGe". IEEE Transactions on Magnetics. 45 (10): 3997. Bibcode:2009ITM....45.3997K. doi:10.1109/TMAG.2009.2022748.
Further reading
- G. Sauthoff: Intermetallics, Wiley-VCH, Weinheim 1995, S. 83 u. 90.
- T. Block; M. J. Carey; B. A. Gurney; O. Jepsen (2004). "Band-structure calculations of the half-metallic ferromagnetism and structural stability of full- and half-Heusler phases". Physical Review. 70 (20): 205114. Bibcode:2004PhRvB..70t5114B. doi:10.1103/PhysRevB.70.205114.
- PJ Webster (1969). "Heusler alloys". Contemporary Physics. 10 (6): 559–577. Bibcode:1969ConPh..10..559W. doi:10.1080/00107516908204800.