Energy efficiency in transport

Part of a series on |
Transport |
---|
Modes |
Topics |
![]() |
The energy efficiency in transport, is the useful travelled distance, of passengers, goods or any type of load; divided by the total energy put into the transport propulsion means. The energy input might be rendered in several different types depending on the type of propulsion, and normally such energy is presented in liquid fuels, electrical energy or food-energy.[1][2] The energy efficiency is also occasionally known as energy intensity.[3] The inverse of the energy efficiency in transport, is the energy consumption in transport.
Energy efficiency in transport is often, and confusingly, described in terms of fuel consumption, being fuel consumption the reciprocal of fuel economy.[2] Nonetheless, fuel consumption is linked with a means of propulsion which uses liquid fuels, whilst energy efficiency is applicable to any sort of propulsion. To avoid said confusion, and to be able to compare the energy efficiency in any type of vehicle, experts tend to measure the energy in the International System of Units, i.e., Joules.
Therefore, in the International System of Units, the energy efficiency in transport is measured in terms of metre per Joule, or m/J, whilst the energy consumption in transport is measured in terms of Joules per meter, or J/m. As more efficient is the vehicle, more metres it covers with one Joule (more efficiency), or less Joules it uses to travel over one meter (less consumption). The energy efficiency in transport largely varies by means of transport. Different types of transport range from some hundred kilojoules per kilometre (kJ/km) for a bicycle to tens of megajoules per kilometer (MJ/km) for a helicopter.
Units of measurement
In the International System of Units, the energy efficiency in transport is measured in terms of metre per Joule, or m/J. Nonetheless, several conversions are applicable, depending on the unit of distance and on the unit of energy. For liquid fuels, normally the quantity of energy input is measured in terms of the liquid's volume, such as litres or gallons. For propulsion which runs on electricity, normally kW·h is used, whilst for any type of human-propelled vehicle, the energy input is measured in terms of Calories. Conversions between different types of energy and units, are very well known in the art.
For passenger transport, the energy efficiency is normally measured in terms of passengers times distance per unit of energy, in the SI, passengers metres per Joule (pax.m/J); whilst for cargo transport the energy efficiency is normally measured in terms of mass of transported cargo times distance per unit of energy, in the SI, kilograms metres per Joule (kg.m/J).
Typical conversions into SI unit
Joules | |
---|---|
Litre of gasoline | 0.3x10⁸ |
US gallon of gasoline[4] | 1.3x10⁸ |
Imp. gallon of gasoline | 1.6x10⁸ |
kilocalorie[5][6] | 4.2x10³ |
kW.h[4] | 3.6x10⁶ |
BTU[4] | 1.1x10³ |
Liquid fuels
Energy efficiency is expressed in terms of fuel economy:[2]
- distance per vehicle per unit fuel volume; e.g., km/L or miles per gallon (US or imperial).
- distance per vehicle per unit fuel mass; e.g., km/kg.[7]
- distance per vehicle per unit energy; e.g., miles per gallon equivalent (mpg-e).
Energy consumption (reciprocal efficiency)[3] is expressed terms of fuel consumption:[2]
- volume of fuel (or total energy) consumed per unit distance per vehicle; e.g. L/100 km or MJ/100 km.
- volume of fuel (or total energy) consumed per unit distance per passenger; e.g., L/(100 passenger·km).
- volume of fuel (or total energy) consumed per unit distance per unit mass of cargo transported; e.g., L/100 kg·km or MJ/t·km.
Electricity
Energy consumption:
- electrical energy used per vehicle per unit distance; e.g., kW·h/100 km.
Food energy
Energy consumption:
- calories burnt by the body's metabolism per kilometre; e.g., Cal/km.
- calories burnt by the body's metabolism per mile; e.g., Cal/miles.[8]
Land transport means
Walking
A 68 kg (150 lb) person walking at 4 km/h (2.5 mph) requires approximately 210 kilocalories (880 kJ) of food energy per hour, which is equivalent to 4.55 km/MJ.[9] 1 US gal (3.8 L) of gasoline contains about 114,000 British thermal units (120 MJ)[10] of energy, so this is approximately equivalent to 360 miles per US gallon (0.65 L/100 km).
Velomobile
Velomobiles have the highest energy efficiency of any known mode of personal transport. At a speed of 50 km/h (31 mph), the velomobile manufacturer WAW claims that only 0.5 kW·h of food energy per 100 km is needed to transport the passenger, which is around 1⁄5 (20%) of what is needed to power a standard upright bicycle, and 1⁄50 (2%) of that which is consumed by an average fossil fuel or electric car. This corresponds to 4700 miles per US gallon (2000 km/L, 0.05 L/100 km).[11] Other sources give a figure of 1⁄3.4 (29.5%) of the energy use of a normal bicycle.[12]
Bicycling

A lightweight and moderate-speed vehicle with low-friction tires and an efficient chain-driven drivetrain, the bicycle is one of the most energy-efficient forms of transport. Compared with walking, a 64 kg (140 lb) cyclist riding at 16 km/h (10 mph) requires about half the food energy per unit distance: 43 kcal/mi, 27 kcal/km or 3.1 kW·h (11 MJ) per 100 km.[9] This converts to about 732 mpg‑US (0.321 L/100 km; 879 mpg‑imp).[13] This means that a bicycle will use between 10-25 times less energy per distance traveled than a personal car, depending on fuel source and size of the car. This figure does depend on the speed and mass of the rider: greater speeds give higher air drag and heavier riders consume more energy per unit distance. In addition, because bicycles are very lightweight (usually between 7–15 kg) this means they consume very low amounts of materials and energy to manufacture, especially in comparison to an automobile weighing 1500 kg or more. This means that a bicycle typically requires 100-200 times less energy to produce than an automobile, which for an automobile can make up a large portion of its total energy use (as discussed below in the automobile section).
A motorized bicycle allows human power and the assistance of a 49 cm3 (3.0 cu in) engine, giving a range of 160 to 200 mpg‑US (1.5–1.2 L/100 km; 190–240 mpg‑imp). Electric pedal-assisted bikes run on as little as 1.0 kW·h (3.6 MJ) per 100 km, while maintaining speeds in excess of 30 km/h (19 mph). These best-case figures rely on a human doing 70% of the work, with around 3.6 MJ (1.0 kW·h) per 100 km coming from the motor. This makes an electric bicycle one of the most efficient possible motorized vehicles, behind only a motorized velomobile.
Human power
To be thorough, a comparison must also consider the energy costs of producing, transporting and packaging of fuel (food or fossil fuel), the energy incurred in disposing of exhaust waste, and the energy costs of manufacturing the vehicle. This last can be significant given that walking requires little or no special equipment, while automobiles, for example, require a great deal of energy to produce and have relatively short lifespans. In addition, any comparison of electric vehicles and liquid-fuelled vehicles must include the fuel consumed in the power station to generate the electricity. In the UK for instance the efficiency of the electricity generation and distribution system is around 0.40.
Automobiles
%2C_5._April_2012%2C_D%C3%BCsseldorf.jpg)
Automobile fuel efficiency is most commonly expressed in terms of the volume of fuel consumed per one hundred kilometres (L/100 km), but in some countries (including the USA, UK and India) it is more commonly expressed in terms of the distance per volume fuel consumed (km/L or miles per US or imperial gallon). This is complicated by the different energy content of fuels such as petrol and diesel. The Oak Ridge National Laboratory (ORNL) states that the energy content of unleaded gasoline is 115,000 British thermal unit (BTU) per US gallon (32 MJ/L) compared to 130,500 BTU per US gallon (36.4 MJ/L) for diesel.[14]
A second important consideration is the energy costs of producing energy. Bio-fuels, electricity and hydrogen, for instance, have significant energy inputs in their production. Because of this, the efficiency of 50-70% (from natural gas) or 10-15% (from electricity) of hydrogen production, as well as the vast amounts of energy required to store and transport hydrogen must to be combined with the vehicle efficiency to yield net efficiency.[15] Because of this, hydrogen automobiles are one of the least efficient means of passenger transport, generally around 50 times as much energy must be put into the production of hydrogen compared to how much is used to move the car.
A third consideration to take into account is the occupancy rate of the vehicle. As the number of passengers per vehicle increases the consumption per unit distance per vehicle increases. However this increase is slight compared to the reduction in consumption per unit distance per passenger. We can compare, for instance, the estimated average occupancy rate of about 1.3 passengers per car in the San Francisco Bay Area[16] to the 2006 UK estimated average of 1.58.[17]
Fourth, the energy needed to build and maintain roads is an important consideration, as is the energy returned on energy invested (EROEI). Between these two factors, roughly 20% must be added to the energy of the fuel consumed, to accurately account for the total energy used.
Finally, vehicle energy efficiency calculations would be misleading without factoring the energy cost of producing the vehicle itself. This initial energy cost can of course be depreciated over the life of the vehicle to calculate an average energy efficiency over its effective life span. In other words, vehicles that take a lot of energy to produce and are used for relatively short periods will require a great deal more energy over their effective lifespan than those that do not, and are therefore much less energy efficient than they may otherwise seem. Hybrid and electric cars use less energy in their operation than comparable petroleum-fueled cars but more energy is used to manufacture them, so the overall difference would be less than immediately apparent. Compare, for example, walking, which requires no special equipment at all, and an automobile, produced in and shipped from another country, and made from parts manufactured around the world from raw materials and minerals mined and processed elsewhere again, and used for a limited number of years. According to the French energy and environment agency ADEME,[18] an average motor car has an embodied energy content of 20,800 kWh and an average electric vehicle amounts to 34,700 kWh. The electric car requires nearly twice as much energy to produce, primarily due to the large amount of mining and purification necessary for the rare earth metals and other materials used in lithium-ion batteries and in the electric drive motors. This represents a significant portion of the energy used over the life of the car (in some cases nearly as much as energy that is used through the fuel that is consumed, effectively doubling the car's per-distance energy consumption), and cannot be ignored when comparing automobiles to other transport modes. It is important to note, also, that as these are average numbers for French automobiles and they are likely to be significantly larger in more auto-centric countries like the United States and Canada, where much larger and heavier cars are more common.
Driving practices and vehicles can be modified to improve their energy efficiency by about 15%.[19][20]
On a percentage basis, if there is one occupant in an automobile, between 0.4-0.6% of the total energy used is used to move the person in the car, while 99.4-99.6% (about 165 to 250 times more) is used to move the car.[21]
Example consumption figures

- Solar cars use no externally supplied fuel other than sunlight, charging the batteries entirely from built-in solar panels, and typically use less than 3 kW·h per 100 miles (67 kJ/km or 1.86 kW·h/100 km). These cars are not designed for passenger or utility use and would not be practical as such due to speed, payload, and inherent design.[22]
- The four passenger GEM NER uses 169 Wh/mi (203 mpg‑e; 10.5 kW·h/100 km),[23] which equates to 2.6 kW·h/100 km per person when fully occupied, albeit at only 24 mph (39 km/h).
- The General Motors EV1 was rated in a test with a charging efficiency of 373 Wh-AC/mile or 23 kWh/100 km[24] approximately equivalent to 2.6 L/100 km (110 mpg‑imp; 90 mpg‑US) for petroleum-fuelled vehicles.
- Chevrolet Volt in full electric mode uses 36 kilowatt-hours per 100 miles (810 kJ/km; 96 mpg‑e), meaning it may approach or exceed the energy efficiency of walking if the car is fully occupied with 4 or more passengers, although the relative emissions produced may not follow the same trends if analyzing environmental impacts.
- The Daihatsu Charade 993cc turbo diesel (1987–1993) won the most fuel efficient vehicle award for going round the United Kingdom consuming an average of 2.82 L/100 km (100 mpg‑imp). It was surpassed only recently by the VW Lupo 3 L which consumes about 2.77 L/100 km (102 mpg‑imp). Both cars are rare to find on the popular market. The Daihatsu had major problems with rust and structural safety which contributes to its rarity and the quite short production run.
- The Volkswagen Polo 1.4 TDI Bluemotion and the SEAT Ibiza 1.4 TDI Ecomotion, both rated at 3.8 L/100 km (74 mpg‑imp; 62 mpg‑US) (combined) were the most fuel efficient petroleum-fueled cars on sale in the UK as of 22 March 2008.[25][26][27]
- Honda Insight – achieves 48 mpg‑US (4.9 L/100 km; 58 mpg‑imp) under real-world conditions.[28]
- Honda Civic Hybrid- regularly averages around 45 mpg‑US (5.2 L/100 km; 54 mpg‑imp).
- Toyota Prius – According to the US EPA's revised estimates, the combined fuel consumption for the 2008 Prius is 46 mpg‑US (5.1 L/100 km; 55 mpg‑imp),[29] making it the most fuel efficient United States car of 2008.[30] In the UK, the official fuel consumption figure (combined) for the Prius is 4.3 L/100 km (66 mpg‑imp; 55 mpg‑US).[31]
- 2012 Cadillac CTS-V Wagon 6.2 L Supercharged, 14 mpg‑US (17 L/100 km; 17 mpg‑imp).[32]
- 2012 Bugatti Veyron, 10 mpg‑US (24 L/100 km; 12 mpg‑imp).[32]
Trains
Trains are in general one of the most efficient means of transport for freight and passengers. Efficiency varies significantly with passenger loads, and losses incurred in electricity generation and supply (for electrified systems),[33][34] and, importantly, end-to-end delivery, where stations are not the originating final destinations of a journey.
Actual consumption depends on gradients, maximum speeds, and loading and stopping patterns. Data produced for the European MEET project (Methodologies for Estimating Air Pollutant Emissions) illustrate the different consumption patterns over several track sections. The results show the consumption for a German ICE high-speed train varied from around 19 to 33 kW·h/km (68–119 MJ/km; 31–53 kW·h/mi). The data also reflects the weight of the train per passenger. For example, TGV double-deck Duplex trains use lightweight materials, which keep axle loads down and reduce damage to track and also save energy.[35]
Freight
Energy consumption estimates for rail freight vary widely, and many are provided by interested parties. Some are tabulated below.
Country | Year | Fuel economy (weight of goods) | Energy Intensity |
---|---|---|---|
USA[36] | 2007 | 185.363 km/L (1 short ton) | energy/mass-distance |
UK[37] | — | 87 t·km/L | 0.41 MJ/t·km (LHV) |
Passenger
Country | Year | Train efficiency | Per passenger-km (kJ) |
---|---|---|---|
Japan[38] | 2004 | 17.9 MJ (5.0 kWh)/car-km | 350 kJ/passenger-km |
EC[39][40] | 1997 | 18 kW·h/km (65 MJ/km) | |
USA[41][42] | 1.125 mpg‑US (209.1 L/100 km; 1.351 mpg‑imp) | 468 passenger-miles/US gallon (0.503 L/100 passenger-km) | |
Switzerland[43] | 2011 | 2300 GWhr/yr | 470 kJ/passenger-km |
Basel, Switzerland[44][45] | 1.53 kWh/vehicle-km (5.51 MJ/vehicle-km) | 85 kJ/passenger-km (150 kJ/passenger-km at 80% average load) | |
USA[46] | 2009 | 2,435 BTU/mi (1.60 MJ/km) | |
Portugal[47] | 2011 | 8.5 kW·h/km (31 MJ/km; 13.7 kW·h/mi) | 77 kJ/passenger-km |
Considering only the energy spent to move the train, and taking as example the urban area of Lisbon, train seems to be on average 7 times more efficient than automobile for transport of passengers, if we consider energy spent per passenger-km.[47] Considering an automobile which has a consumptions of around 6 l/100 km (47 mpg‑imp; 39 mpg‑US) of gasoline, the fact the on average cars in Europe have an occupation ratio of around 1.2 passengers per automobile and that one litre of gasoline amounts for about 8826 Wh, one gets on average 441 Wh (1,590 kJ) per passenger-km. On the other hand, a modern urban train with an average occupation of 20% of total capacity, which has a consumption of about 8.5 kW·h/km (31 MJ/km; 13.7 kW·h/mi), after accounting for energy lost in making the electricity it uses, requires 61.5 Wh of energy per passenger-km, 1/7th of that of the automobile.
Braking losses
Stopping is a considerable source of inefficiency. Modern electric trains like the Shinkansen (the Bullet Train) use regenerative braking to return current into the catenary while they brake. A Siemens study indicated that regenerative braking might recover 41.6% of the total energy consumed. The Passenger Rail (Urban and Intercity) and Scheduled Intercity and All Charter Bus Industries Technological and Operational Improvements – FINAL REPORT states that "Commuter operations can dissipate more than half of their total traction energy in braking for stops." and that "We estimate head-end power to be 35 percent (but it could possibly be as high as 45 percent) of total energy consumed by commuter railways."[48] Having to accelerate and decelerate a heavy train load of people at every stop is inefficient despite regenerative braking which can recover typically around 20% of the energy wasted in braking. Weight is a determinant of braking losses.
Other references
AEA study of road and rail for the United Kingdom Department for Transport: Final report
Buses
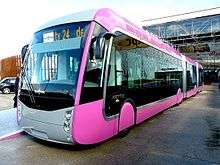
- In July 2005, the average occupancy for buses in the UK was stated to be 9.[50]
- The fleet of 244 40-foot (12 m) 1982 New Flyer trolley buses in local service with BC Transit in Vancouver, Canada, in 1994/95 used 35,454,170 kWh for 12,966,285 vehicle km, or 9.84 MJ/vehicle km. Exact ridership on trolleybuses is not known, but with all 34 seats filled this equates to 0.32 MJ/passenger km. It is quite common to see people standing on Vancouver trolleybuses. This is a service with many stops per kilometre; part of the reason for the efficiency is the use of regenerative braking.
- A commuter service in Santa Barbara, California, USA, found average diesel bus efficiency of 6.0 mpg‑US (39 L/100 km; 7.2 mpg‑imp) (using MCI 102DL3 buses). With all 55 seats filled this equates to 330 passenger mpg; with 70% filled, 231 passenger mpg.[51]
- In 2011 the fleet of 752 buses in the city of Lisbon had an average speed of 14.4 km/h and an average occupancy of 20.1 passengers per vehicle.[52]
Other
- NASA's Crawler-Transporter is used to move the Shuttle from storage to the launch pad. It uses diesel and has one of the highest fuel consumption rates on record, 150 US gallons per mile (350 l/km; 120 imp gal/mi).[53]
Air transport means
Aircraft
A principal determinant of energy consumption in aircraft is drag, which must be opposed by thrust for the aircraft to progress.
- Drag is proportional to the lift required for flight,[54] which is equal to the weight of the aircraft. As induced drag increases with weight, mass reduction, with improvements in engine efficiency and reductions in aerodynamic drag, has been a principal source of efficiency gains in aircraft, with a rule-of-thumb being that a 1% weight reduction corresponds to around a 0.75% reduction in fuel consumption.[54]
- Flight altitude affects engine efficiency. Jet-engine efficiency increases at altitude up to the tropopause, the temperature minimum of the atmosphere; at lower temperatures, the Carnot efficiency is higher.[54] Jet engine efficiency is also increased at high speeds, but above about Mach 0.85 the airframe aerodynamic losses increase faster.
- Compressibility effects: beginning at transonic speeds of around Mach 0.85, shockwaves form increasing drag.
- For supersonic flight, it is difficult to achieve a lift to drag ratio greater than 5, and fuel consumption is increased in proportion.
Aircraft | Concorde[55] | Boeing 747-400[56] |
---|---|---|
Passenger-miles/imperial gallon | 17 | 109 |
Passenger-miles/US gallon | 14 | 91 |
Litres/100 passenger-km | 16.6 | 3.1 |
Passenger airplanes averaged 4.8 l/100 km per passenger (1.4 MJ/passenger-km) (49 passenger-miles per gallon) in 1998. Note that on average 20% of seats are left unoccupied. Jet aircraft efficiencies are improving: Between 1960 and 2000 there was a 55% overall fuel efficiency gain (if one were to exclude the inefficient and limited fleet of the DH Comet 4 and to consider the Boeing 707 as the base case).[57] Most of the improvements in efficiency were gained in the first decade when jet craft first came into widespread commercial use. Compared to advanced piston engine airliners of the 1950s, current jet airliners are only marginally more efficient per passenger-mile.[58] Between 1971 and 1998 the fleet-average annual improvement per available seat-kilometre was estimated at 2.4%. Concorde the supersonic transport managed about 17 passenger-miles to the Imperial gallon; similar to a business jet, but much worse than a subsonic turbofan aircraft. Airbus puts the fuel rate consumption of their A380 at less than 3 l/100 km per passenger (78 passenger-miles per US gallon).[59]

The mass of an aircraft can be reduced by using light-weight materials such as titanium, carbon fiber and other composite plastics. Expensive materials may be used, if the reduction of mass justifies the price of materials through improved fuel efficiency. The improvements achieved in fuel efficiency by mass reduction, reduces the amount of fuel that needs to be carried. This further reduces the mass of the aircraft and therefore enables further gains in fuel efficiency. For example, the Airbus A380 design includes multiple light-weight materials.
Airbus has showcased wingtip devices (sharklets or winglets) that can achieve 3.5 percent reduction in fuel consumption.[60][61] There are wingtip devices on the Airbus A380. Further developed Minix winglets have been said to offer 6 percent reduction in fuel consumption.[62] Winglets at the tip of an aircraft wing smooth out the wing-tip vortex (reducing the aircraft's wing drag) and can be retrofitted to any airplane.[62]
NASA and Boeing are conducting tests on a 500 lb (230 kg) "blended wing" aircraft. This design allows for greater fuel efficiency since the whole craft produces lift, not just the wings.[63] The blended wing body (BWB) concept offers advantages in structural, aerodynamic and operating efficiencies over today's more conventional fuselage-and-wing designs. These features translate into greater range, fuel economy, reliability and life cycle savings, as well as lower manufacturing costs.[64][65] NASA has created a cruise efficient STOL (CESTOL) concept.
Fraunhofer Institute for Manufacturing Engineering and Applied Materials Research (IFAM) have researched a shark skin imitating paint that would reduce drag through a riblet effect.[66] Aircraft are a major potential application for new technologies such as aluminium metal foam and nanotechnology such as the shark skin imitating paint.
Propfan propulsors are a more fuel efficient technology than jets or turboprops, but turboprops have an optimum speed below about 450 mph (700 km/h).[67] This speed is less than used with jets by major airlines today. However, the decrease in speed reduces drag. With the current high price for jet fuel and the emphasis on engine/airframe efficiency to reduce emissions, there is renewed interest in the propfan concept for jetliners that might come into service beyond the Boeing 787 and Airbus A350XWB. For instance, Airbus has patented aircraft designs with twin rear-mounted counter-rotating propfans.[68] NASA has conducted an Advanced Turboprop Project (ATP), where they researched a variable pitch propfan that produced less noise and achieved high speeds.
Related to fuel efficiency is the impact of aviation emissions on climate.
Small aircraft

- Motor-gliders made of composite materials can reach an extremely low fuel consumption for cross-country flights, using thermal air currents and winds.
- At 160 km/h, a diesel powered two-seater Dieselis burns 6 liters of fuel per hour, 1.9 liters per 100 passenger km.[69]
- at 220 km/h, a four-seater 100 hp MCR-4S burns 20 liters of gas per hour, 2.2 liters per 100 passenger km.
- Under continuous motorised flight at 225 km/h, a Pipistrel Sinus burns 11 liters of fuel per flight hour. Carrying 2 people aboard, it operates at 2.4 liters per 100 passenger km.
- Ultralight aircraft Tecnam P92 Echo Classic at cruise speed of 185 km/h burns 17 liters of fuel per flight hour, 4.6 liters per 100 passenger km (2 people).[70] Other modern ultralight aircraft have increased efficiency; Tecnam P2002 Sierra RG at cruise speed of 237 km/h burns 17 liters of fuel per flight hour, 3.6 liters per 100 passenger km (2 people).[71]
- Two-seater and four-seater flying at 250 km/h with old generation engines can burn 25 to 40 liters per flight hour, 3 to 5 liters per 100 passenger km.
- The Sikorsky S-76C++ twin turbine helicopter gets about 1.65 mpg‑US (143 L/100 km; 1.98 mpg‑imp) at 140 knots (260 km/h; 160 mph) and carries 12 for about 19.8 passenger-miles per gallon (11.9 L per 100 passenger km).
Water transport means
Ships
Queen Elizabeth
Cunard stated that Queen Elizabeth 2 travelled 49.5 feet per imperial gallon of diesel oil (3.32 m/l or 41.2 ft/US gal), and that it had a passenger capacity of 1777.[72] Thus carrying 1777 passengers we can calculate an efficiency of 16.7 passenger miles per imperial gallon (16.9 l/100 p·km or 13.9 p·mpg–US).
Cruise ships
MS Oasis of the Seas has a capacity of 6,296 passengers and a fuel efficiency of 14.4 passenger miles per US gallon. Voyager-class cruise ships have a capacity of 3,114 passengers and a fuel efficiency of 12.8 passenger miles per US gallon.[73]
Emma Maersk
Emma Maersk uses a Wärtsilä-Sulzer RTA96-C, which consumes 163 g/kW·h and 13,000 kg/h. If it carries 13,000 containers then 1 kg fuel transports one container for one hour over a distance of 45 km. The ship takes 18 days from Tanjung (Singapore) to Rotterdam (Netherlands), 11 from Tanjung to Suez, and 7 from Suez to Rotterdam,[74] which is roughly 430 hours, and has 80 MW, +30 MW. 18 days at a mean speed of 25 knots (46 km/h) gives a total distance of 10,800 nautical miles (20,000 km).
Sailboats
A sailboat, much like a solar car, can locomote without consuming any fuel. A sail boat such as a Dinghy using just wind power requires no input energy in terms of fuel. However some manual energy is required by the crew to steer the boat and adjust the sails using ropes. In addition energy will be needed for demands other than propulsion, such as cooking, heating or lighting. The fuel efficiency of a single-occupancy boat is highly dependent on the size of its engine, the speed at which it travels, and its displacement. Due to the high viscosity of water, with a single passenger, the equivalent energy efficiency will be lower than in a car, train, or plane.
International transport comparisons
European Public transport
Rail and bus are generally required to serve 'off peak' and rural services, which by their nature have lower loads than city bus routes and inter city train lines. Moreover, due to their 'walk on' ticketing it is much harder to match daily demand and passenger numbers. As a consequence, the overall load factor on UK railways is 35% or 90 people per train:[75]
Conversely, airline services generally work on point-to-point networks between large population centres and are 'pre-book' in nature. Using yield management, overall load factors can be raised to around 70–90%. Intercity train operators have begun to use similar techniques, with loads reaching typically 71% overall for TGV services in France and a similar figure for the UK's Virgin Trains services.[76]
For emissions, the electricity generating source needs to be taken into account.[77][78] [79]
US Passenger transport
The US transport Energy Data Book states the following figures for passenger transport in 2009:[46]
Transport mode | Average passengers per vehicle |
BTU per passenger-mile |
MJ per passenger-kilometre |
---|---|---|---|
Rail (intercity Amtrak) | 20.9 | 2,435 | 1.596 |
Motorcycles | 1.16 | 2,460 | 1.61 |
Rail (transit light & heavy) | 24.5 | 2,516 | 1.649 |
Rail (commuter) | 32.7 | 2,812 | 1.843 |
Air | 99.3 | 2,826 | 1.853 |
Cars | 1.55 | 3,538 | 2.319 |
Personal trucks | 1.84 | 3,663 | 2.401 |
Buses (transit) | 9.2 | 4,242 | 2.781 |
Taxi | 1.55 | 15,645 | 10.257 |
US Freight transport
The US transport Energy book states the following figures for freight transport in 2010:[46][80][81][82]
transport mode | Fuel consumption | |
---|---|---|
BTU per short ton-mile | kJ per tonne-kilometre | |
Domestic waterborne | 217 | 160 |
Class 1 railroads | 289 | 209 |
Heavy trucks | 3,357 | 2,426 |
Air freight (approx.) | 9,600 | 6,900 |
From 1960 to 2010 the efficiency of air freight has increased 75%, mostly due to more efficient jet engines.[83]
1 gal-US (3.785 l, 0.833 gal-imp) of fuel can move a ton of cargo 857 km or 462 nmi by barge, or 337 km (209 mi) by rail, or 98 km (61 mi) by truck.[84]
Compare:
- Space Shuttle used to transport freight to the other side of the Earth (see above): 40 megajoules per tonne-kilometre.
- Net energy for lifting: 10 megajoules per tonne-kilometre.
Canadian transport
Natural Resources Canada's Office of Energy Efficiency publishes annual statistics regarding the efficiency of the entire Canadian fleet. For researchers, these fuel consumption estimates are more realistic than the fuel consumption ratings of new vehicles, as they represent the real world driving conditions, including extreme weather and traffic. The annual report is called Energy Efficiency Trends Analysis. There are dozens of tables illustrating trends in energy consumption expressed in energy per passenger km (passengers) or energy per tonne km (freight).[85]
French environmental calculator
The environmental calculator of the French environment and energy agency (ADEME) published in 2007 using data from 2005[86] enables one to compare the different means of transport as regards the CO2 emissions (in terms of carbon dioxide equivalent) as well as the consumption of primary energy. In the case of an electric vehicle, the ADEME makes the assumption that 2.58 toe as primary energy are necessary for producing one toe of electricity as end energy in France (see Embodied energy#Embodied energy in the energy field).
This computer tool devised by the ADEME shows the importance of public transport from an environmental point of view. It highlights the primary energy consumption as well as the CO2 emissions due to transport. Due to the relatively low environmental impact of radioactive waste, compared to that of fossil fuel combustion emissions, this is not a factor in the tool. Moreover, intermodal passenger transport is probably a key to sustainable transport, by allowing people to use less polluting means of transport.
German environmental costs
Deutsche Bahn calculates their energy consumption for their various routes they take.
Type | 2015 |
---|---|
Regional rail passenger transport (MJ/pkm) | 0.98 |
Long-distance rail passenger transport (MJ/pkm) | 0.38 |
Bus service (MJ/Pkm) | 1.22 |
Rail freight transport (MJ/tkm) | 0.35 |
Road freight transport (MJ/tkm) | 1.31 |
Air freight (MJ/tkm) | 10.46 |
Oceanfreight (MJ/tkm) | 0.11 |
Footnotes
- ↑ "Efficiency". Retrieved 2016-09-18.
- 1 2 3 4 "Assessment of Fuel Economy Technologies for Light-duty Vehicles". The National Academies Press. 2011. ISBN 978-0-309-15607-3. Retrieved 2016-09-18.
- 1 2 "Glossary of energy-related terms". U.S. Department of Energy. Retrieved 2016-09-20.
- 1 2 3 "Energy Content of Fuels (in Joules)" (PDF).
- ↑ "Calories - Joules units conversion". www.unitsconversion.com.ar. Retrieved 2017-06-24.
- ↑ "Energy Units". www.aps.org. Retrieved 2017-06-24.
- ↑ Aeroplane Efficiency, Fédération Aéronautique Internationale, "FAI – The World Air Sports Federation"
- ↑ "Calories Burned Calculator". Runner's World. 2016-08-05. Retrieved 2017-06-23.
- 1 2 Mackenzie, Brian. "Energy expenditure for walking and running".
- ↑ EPA (2007). "Appendix B, Transportation Energy Data Book". Retrieved 16 November 2010.
- ↑ "Waw:: a practical sports car :: - mobilitylab.be".
- ↑ "The velomobile: high-tech bike or low-tech car?".
- ↑ "Calculation of conversion from dietary calories per mile to miles per gallon gasoline, using the energy density of gasoline listed by Wolfram Alpha". 2011. Retrieved 19 Jul 2011.
- ↑ "Oak Ridge National Laboratory (ORNL)". Archived from the original on 27 September 2011.
- ↑ "Vehicle Technologies Office - Department of Energy" (PDF).
- ↑ Maps and Data – Metropolitan Transportation Commission for the nine-county San Francisco Bay Area, California
- ↑ "Transport trends: current edition". UK Department for Transport. 8 January 2008. Archived from the original on 22 April 2008. Retrieved 23 March 2008.
- ↑ (fr) Life cycle assessment website www.ademe.fr see page 9
- ↑ Beusen; et al. (2009). "Using on-board logging devices to study the long-term impact of an eco-driving course". Transportation Research D. 14: 514–520.
- ↑ "Do lower speed limits on motorways reduce fuel consumption and pollutant emissions?". Retrieved 2013-08-12.
- ↑ "GreatestPlanet,, August 2007". www.greatestplanet.org. 10 August 2007. Retrieved 10 August 2007.
- ↑ "MIT Unveils 90 MPH Solar Race Car".
- ↑ "Vehicle Technologies Office - Department of Energy" (PDF).
- ↑ "Vehicle Technologies Office - Department of Energy" (PDF).
- ↑ "Best on CO2 rankings". UK Department for Transport. Archived from the original on 12 March 2008. Retrieved 2008-03-22.
- ↑ "Vehicle details for Polo 3 / 5 Door (from Nov 06 Wk 45>) 1.4 TDI (80PS) (without A/C) with DPF BLUEMOTION M5". UK Vehicle Certification Agency. Archived from the original on 10 February 2009. Retrieved 22 March 2008.
- ↑ "Vehicle details for Ibiza ( from NOV 06 Wk 45 > ) 1.4 TDI 80PS Ecomotion M5". UK Vehicle Certification Agency. Archived from the original on 10 February 2009. Retrieved 22 March 2008.
- ↑ Jerry Garrett (2006-08-27). "The Once and Future Mileage King". The New York Times.
- ↑ "2008 Toyota Prius". EPA. Retrieved 2007-12-25.
- ↑ "2008 Most and Least Fuel Efficient cars (ranked by city mpg)". United States Environmental Protection Agency and United States Department of Energy. Retrieved 2007-12-25.
- ↑ "Vehicle details for Prius 1.5 VVT-i Hybrid E-CVT". UK Vehicle Certification Agency. Archived from the original on 5 June 2008. Retrieved 2008-03-22.
- 1 2 "2016 Best and Worst Fuel Economy Vehicles".
- ↑ "Fuel-Efficiency of Travel in the 20th Century: Appendix-Notes".
- ↑ Fuel Efficiency of Travel in the 20th Century)
- ↑ "Commission for integrated transport, Short haul air v High speed rail".
- ↑ "Rail News August 2016 - For Railroad Career Professionals From Progressive Railroading magazine".
- ↑ freightonrail.co.uk
- ↑ Environmental Goals and Results, JR-East Sustainability Report 2005
- ↑ TGV Duplex assuming 3 intermediate stops between Paris and Lyon.
- ↑ Estimating Emissions from Railway Traffic, page 74
- ↑ Colorado Railcar double-deck DMU hauling two Bombardier Bi-level coaches
- ↑ Colorado Railcar: "DMU Performs Flawlessly on Tri-Rail Service Test"
- ↑ SBB Facts and Figures Traffic
- ↑ European Environment Agency Occupancy Rates, page 3
- ↑ Combino – Low Floor Light Rail Vehicles Tests, Trials and Tangible Results
- 1 2 3 Davis, Stacy C.; Susan W. Diegel; Robert G. Boundy (2011). Transportation Energy Data Book: Edition 30. US Department of Energy. pp. Table 2.14. ORNL-6986 (Edition 30 of ORNL-5198). Retrieved 2012-02-22.
- 1 2 "Eficiência energética: carro ou comboio?".
- ↑ Bus and Rail Final Report
- ↑ "Van Hool presents the ExquiCity Design Mettis.". Archived from the original on 5 June 2013. Retrieved 5 June 2012.
- ↑ "Passenger Transport (Fuel Consumption)". Hansard. UK House of Commons. 2005-07-20. Retrieved 2008-03-25.
- ↑ Demonstration of Caterpillar C-10 Duel-Fuel Engines in MCI 102DL3 Commuter Buses
- ↑ Seara.com. "Indicadores de Atividade".
- ↑ "Crawler-Transporter System".
- 1 2 3 Barney L. Capehart (2007). Encyclopedia of Energy Engineering and Technology, Volume 1. CRC Press. ISBN 0-8493-3653-8, ISBN 978-0-8493-3653-9.
- ↑ "Powerplant." concordesst.com. Retrieved: 2 December 2009.
- ↑ "Technical Specifications: Boeing 747-400". Boeing. Retrieved 11 January 2010.
- ↑ National Aerospace Laboratory
- ↑ Peeters P.M., Middel J., Hoolhorst A. (2005). Fuel efficiency of commercial aircraft An overview of historical and future trends. National Aerospace Laboratory, The Netherlands.
- ↑ "The A380: The future of flying". Airbus. Archived from the original on 14 December 2007. Retrieved 2008-03-22.
- ↑ Bradley, Grant (17 November 2009). "'Shark fin' wings give airline chiefs something to smile about" – via New Zealand Herald.
- ↑ "A320 plane shark fins small winglets successfully completed the first flight test".
- 1 2 "Minix wing tip device promises 6% gain in fuel efficiency for airliners".
- ↑ Ecogeek Article
- ↑ "Boeing to Begin Ground Testing of X-48B Blended Wing Body Concept." Archived 19 August 2012 at the Wayback Machine. Boeing, October 27, 2006. Retrieved: April 10, 2012.
- ↑ Lorenz III, Phillip. "AEDC testing brings unique blended wing aircraft closer to flight." Archived 14 July 2014 at the Wayback Machine. AEDC, U.S. Air Force, July 3, 2007. Retrieved: April 10, 2012.
- ↑ Mahony, Melissa. "A sharkskin coating for ships, planes and blades - ZDNet".
- ↑ Spakovszky, Zoltan (2009). "Unified Propulsion Lecture 1". Unified Engineering Lecture Notes. MIT. Retrieved 2009-04-03.
- ↑ US application 2009020643, Airbus & Christophe Cros, "Aircraft having reduced environmental impact", published 2009-01-22
- ↑ Contact, Experimental Aircraft and Powerplant Newsforum for Designers and Builders, Issue 55, March–April 2000
- ↑ "Tecnam P92 Echo Classic". Tecnam costruzioni aeronautiche s.r.l. Archived from the original on 29 May 2012. Retrieved 22 May 2012.
- ↑ "Tecnam P2002 Sierra De Luxe". Tecnam costruzioni aeronautiche s.r.l. Archived from the original on 8 June 2012. Retrieved 22 May 2012.
- ↑ "Queen Elizabeth 2: Technical Information" (PDF). Cunard Line. Archived from the original (PDF) on 18 March 2009. Retrieved 2008-03-31.
- ↑ "Cruise Ship Gas Mileage".
- ↑ Emma Mærsk schedules Mærsk, 5 December 2011.
- ↑ "ATOC".
- ↑ "Delivering a sustainable railway - Publications - GOV.UK".
- ↑ "Energy & Emissions Statement" (PDF).
- ↑ Defra 2008 Guidelines to Defra’s GHG Conversion Factors
- ↑ Kilograms of CO2 per passenger kilometre for different modes of transport within the UK
- ↑ US Environmental protection, 2006
- ↑ Energy Efficiency – Transportation sector (from the United States Department of Energy's Energy Information Administration)
- ↑ Energy Table 2.15
- ↑ "Trends in Fuel Efficiency, Selected Passenger Jet Planes".
- ↑ Rodrigue, Dr. Jean-Paul. "Transportation and Energy".
- ↑ 2010 data
- ↑ (fr) ADEME environmental calculator which informs about the CO2 emissions and the primary energy consumption
- ↑ Deutsche Bahn
See also
- ACEA agreement
- Alternative propulsion
- Corporate Average Fuel Economy (CAFE)
- Carbon dioxide equivalent and emission standard
- Gasoline gallon equivalent
- Gas-guzzler
- Life cycle assessment
External links
- transport Research at the National Renewable Energy Laboratory
- ECCM Study for rail, road and air journeys between main UK cities
- Transport Energy Consumption Discussion Paper 2004 – Prof. Roger Kemp
- Traction Summary Report 2007- Prof. Roger Kemp
- transport Energy Data Book (US)
- Fuel Consumption Ratings
- Energy Efficiency of different modes of transport by James Strickland, M.Sc. Standford, 2009