Histone methyltransferase
histone-lysine N-methyltransferase | |||||||||
---|---|---|---|---|---|---|---|---|---|
Identifiers | |||||||||
EC number | 2.1.1.43 | ||||||||
CAS number | 9055-08-7 | ||||||||
Databases | |||||||||
IntEnz | IntEnz view | ||||||||
BRENDA | BRENDA entry | ||||||||
ExPASy | NiceZyme view | ||||||||
KEGG | KEGG entry | ||||||||
MetaCyc | metabolic pathway | ||||||||
PRIAM | profile | ||||||||
PDB structures | RCSB PDB PDBe PDBsum | ||||||||
Gene Ontology | AmiGO / EGO | ||||||||
|
Histone methyltransferases (HMT) are histone-modifying enzymes (e.g., histone-lysine N-methyltransferases and histone-arginine N-methyltransferases), that catalyze the transfer of one, two, or three methyl groups to lysine and arginine residues of histone proteins. The attachment of methyl groups occurs predominantly at specific lysine or arginine residues on histones H3 and H4.[1] Two major types of histone methyltranferases exist, lysine-specific (which can be SET (Su(var)3-9, Enhancer of Zeste, Trithorax) domain containing or non-SET domain containing) and arginine-specific.[2][3][4] In both types of histone methyltransferases, cofactor S-Adenosyl methionine (SAM) serves as a cofactor and methyl donor group.[1][5][6][7] In eukaryotic cells, the genome is tightly condensed into chromatin (composed of DNA and histone proteins[8]), so enzymes, such as histone methyltransferases, must overcome this inaccessibility.[9] Histone methyltransferase does so by modifying histones at certain sites through methylation. Methylation of histones is important biologically because it is the principal epigenetic modification of chromatin that determines gene expression, genomic stability, stem cell maturation, cell lineage development, genetic imprinting, DNA methylation, and cell mitosis.[2]
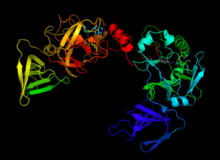
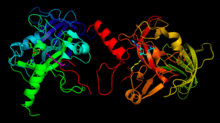
Types
The class of lysine-specific histone methyltransferases is subdivided into SET domain-containing and non-SET domain-containing. As indicated by their monikers, these differ in the presence of a SET domain, which is a type of protein domain.
Human genes encoding proteins with histone methyltransferase activity include:
- ASH1L
- DOT1L
- EHMT1, EHMT2, EZH1, EZH2
- MLL, MLL2, MLL3, MLL4, MLL5
- NSD1
- PRDM2
- SET, SETBP1, SETD1A, SETD1B, SETD2, SETD3, SETD4, SETD5, SETD6, SETD7, SETD8, SETD9, SETDB1, SETDB2
- SETMAR, SMYD1, SMYD2, SMYD3, SMYD4, SMYD5, SUV39H1, SUV39H2, SUV420H1, SUV420H2
SET domain-containing lysine-specific
Structure
The structures involved in methyltransferase activity are the SET domain (composed of approximately 130 amino acids), the pre-SET, and the post-SET domains. The pre-SET and post-SET domains flank the SET domain on either side. The pre-SET region contains cysteine residues that form triangular zinc clusters, tightly binding the zinc atoms and stabilizing the structure. The SET domain itself contains a catalytic core rich in β-strands that, in turn, make up several regions of β-sheets. Often, the β-strands found in the pre-SET domain will form β-sheets with the β-strands of the SET domain, leading to slight variations to the SET domain structure. These small changes alter the target residue site specificity for methylation and allow the SET domain methyltransferases to target many different residues. This interplay between the pre-SET domain and the catalytic core is critical for enzyme function.[1]
Catalytic mechanism
In order for the reaction to proceed, S-Adenosyl methionine (SAM) and the lysine residue of the substrate histone tail must first be bound and properly oriented in the catalytic pocket of the SET domain. Next, a nearby tyrosine residue deprotonates the ε-amino group of the lysine residue.[10] The lysine chain then makes a nucleophilic attack on the methyl group on the sulfur atom of the SAM molecule, transferring the methyl group to the lysine side chain.
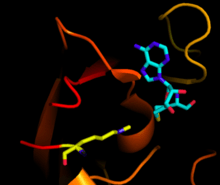
Non-SET domain-containing lysine-specific
Instead of SET, non-SET domain-containing histone methyltransferase utilizes the enzyme Dot1. Unlike the SET domain, which targets the lysine tail region of the histone, Dot1 methylates a lysine residue in the globular core of the histone, and is the only enzyme known to do so.[1] A possible homolog of Dot1 was found in archaea which shows the ability to methylate archaeal histone-like protein in recent studies.
Structure
The N terminal of Dot1 contains the active site. A loop serving as the binding site for SAM links the N-terminal and the C-terminal domains of the Dot1 catalytic domain. The C-terminal is important for the substrate specificity and binding of Dot1 because the region carries a positive charge, allowing for a favorable interaction with the negatively charged backbone of DNA.[11] Due to structural constraints, Dot1 is only able to methylate histone H3.
Arginine-specific
There are two different types of protein arginine methyltransferases (PRMTs) and three types of methylation that can occur at arginine residues on histone tails. The first type of PRMTs (PRMT1, PRMT3, CARM1⧸PRMT4, and Rmt1⧸Hmt1) produce monomethylarginine and asymmetric dimethylarginine.[12][13][14] The second type (JBP1⧸PRMT5) produces monomethyl or symmetric dimethylarginine.[5] The differences in the two types of PRMTs arise from restrictions in the arginine binding pocket.[5]
Structure
The catalytic domain of PRMTs consists of a SAM binding domain and substrate binding domain (about 310 amino acids in total).[5][6][7] Each PRMT has a unique N-terminal region and a catalytic core. The arginine residue and SAM must be correctly oriented within the binding pocket. SAM is secured inside the pocket by a hydrophobic interaction between an adenine ring and a phenyl ring of a phenylalanine.[7]
Catalytic mechanism
A glutamate on a nearby loop interacts with nitrogens on the target arginine residue. This interaction redistributes the positive charge and leads to the deprotonation of one nitrogen group,[15] which can then make a nucleophilic attack on the methyl group of SAM. Differences between the two types of PRMTs determine the next methylation step: either catalyzing the dimethylation of one nitrogen or allowing the symmetric methylation of both groups.[5] However, in both cases the proton stripped from the nitrogen is dispersed through a histidine–aspartate proton relay system and released into the surrounding matrix.[16]
Role in gene regulation
Histone methylation plays an important role in epigenetic gene regulation. Methylated histones can either repress or activate transcription as different experimental findings suggest. For example, it is likely that the methylation of lysine 9 on histone H3 (H3K9me3) in the promoter region of genes prevents excessive expression of these genes and, therefore, delays cell cycle transition and/or proliferation.[17] See Histone#Chromatin regulation.
Disease relevance
Abnormal expression or activity of methylation-regulating enzymes has been noted in some types of human cancers, suggesting associations between histone methylation and malignant transformation of cells or formation of tumors.[17] In recent years, epigenetic modification of the histone proteins, especially the methylation of the histone H3, in cancer development has been an area of emerging research. It is now generally accepted that in addition to genetic aberrations, cancer can be initiated by epigenetic changes in which gene expression is altered without genomic abnormalities. These epigenetic changes include loss or gain of methylations in both DNA and histone proteins.[17]
There is not yet compelling evidence that suggests cancers develop purely by abnormalities in histone methylation or its signaling pathways, however they may be a contributing factor. For example, down-regulation of methylation of lysine 9 on histone 3 (H3K9me3) has been observed in several types of human cancer (such as colorectal cancer, ovarian cancer, and lung cancer), which arise from either the deficiency of H3K9 methyltransferases or elevated activity or expression of H3K9 demethylases.[17][18][19]
Further research
Histone methyltransferase may be able to be used as biomarkers for the diagnosis and prognosis of cancers. Additionally, many questions still remain about the function and regulation of histone methyltransferases in malignant transformation of cells, carcinogenesis of the tissue, and tumorigenesis.[17]
See also
- Histone-Modifying Enzymes
- Histone acetyltransferase (HAT)
- Histone deacetylase (HDAC)
- RNA polymerase control by chromatin structure
- Histone methylation
References
- 1 2 3 4 Wood A (2004). "Posttranslational Modifications of Histones by Methylation". In Conaway JW, Conaway RC. Proteins in eukaryotic transcription. Advances in Protein Chemistry. 67. Amsterdam: Elsevier Academic Press. pp. 201–222. ISBN 0-12-034267-7. doi:10.1016/S0065-3233(04)67008-2.
- 1 2 Sawan C, Herceg Z (2010). "Histone Modifications and Cancer". In Ushijima T, Herceg Z. Epigenetics and Cancer, Part A, Volume 70. Advances in Genetics. 70. Boston: Academic Press. pp. 57–85. ISBN 0-12-380866-9. doi:10.1016/B978-0-12-380866-0.60003-4.
- ↑ Feng Q, Wang H, Ng HH, Erdjument-Bromage H, Tempst P, Struhl K, Zhang Y (June 2002). "Methylation of H3-lysine 79 is mediated by a new family of HMTases without a SET domain". Curr. Biol. 12 (12): 1052–8. PMID 12123582. doi:10.1016/S0960-9822(02)00901-6.
- ↑ Ng HH, Feng Q, Wang H, Erdjument-Bromage H, Tempst P, Zhang Y, Struhl K (June 2002). "Lysine methylation within the globular domain of histone H3 by Dot1 is important for telomeric silencing and Sir protein association". Genes Dev. 16 (12): 1518–27. PMC 186335
. PMID 12080090. doi:10.1101/gad.1001502.
- 1 2 3 4 5 Branscombe TL, Frankel A, Lee JH, Cook JR, Yang Z, Pestka S, Clarke S (August 2001). "PRMT5 (Janus kinase-binding protein 1) catalyzes the formation of symmetric dimethylarginine residues in proteins". J. Biol. Chem. 276 (35): 32971–6. PMID 11413150. doi:10.1074/jbc.M105412200.
- 1 2 Weiss VH, McBride AE, Soriano MA, Filman DJ, Silver PA, Hogle JM (December 2000). "The structure and oligomerization of the yeast arginine methyltransferase, Hmt1". Nat. Struct. Biol. 7 (12): 1165–71. PMID 11101900. doi:10.1038/82028.
- 1 2 3 Zhang X, Zhou L, Cheng X (July 2000). "Crystal structure of the conserved core of protein arginine methyltransferase PRMT3". EMBO J. 19 (14): 3509–19. PMC 313989
. PMID 10899106. doi:10.1093/emboj/19.14.3509.
- ↑ "Chromatin Network". Retrieved 1 March 2012.
- ↑ Kouzarides T (February 2007). "Chromatin modifications and their function". Cell. 128 (4): 693–705. PMID 17320507. doi:10.1016/j.cell.2007.02.005.
- ↑ Trievel RC, Beach BM, Dirk LM, Houtz RL, Hurley JH (October 2002). "Structure and catalytic mechanism of a SET domain protein methyltransferase". Cell. 111 (1): 91–103. PMID 12372303. doi:10.1016/S0092-8674(02)01000-0.
- ↑ Min J, Feng Q, Li Z, Zhang Y, Xu RM (March 2003). "Structure of the catalytic domain of human DOT1L, a non-SET domain nucleosomal histone methyltransferase". Cell. 112 (5): 711–23. PMID 12628190. doi:10.1016/S0092-8674(03)00114-4.
- ↑ Chen D, Ma H, Hong H, Koh SS, Huang SM, Schurter BT, Aswad DW, Stallcup MR (June 1999). "Regulation of transcription by a protein methyltransferase". Science. 284 (5423): 2174–7. PMID 10381882. doi:10.1126/science.284.5423.2174.
- ↑ Gary JD, Lin WJ, Yang MC, Herschman HR, Clarke S (May 1996). "The predominant protein-arginine methyltransferase from Saccharomyces cerevisiae". J. Biol. Chem. 271 (21): 12585–94. PMID 8647869. doi:10.1074/jbc.271.21.12585.
- ↑ McBride AE, Weiss VH, Kim HK, Hogle JM, Silver PA (February 2000). "Analysis of the yeast arginine methyltransferase Hmt1p/Rmt1p and its in vivo function. Cofactor binding and substrate interactions". J. Biol. Chem. 275 (5): 3128–36. PMID 10652296. doi:10.1074/jbc.275.5.3128.
- ↑ McBride AE, Silver PA (July 2001). "State of the arg: protein methylation at arginine comes of age". Cell. 106 (1): 5–8. PMID 11461695. doi:10.1016/S0092-8674(01)00423-8.
- ↑ Fersht AR, Sperling J (February 1973). "The charge relay system in chymotrypsin and chymotrypsinogen". J. Mol. Biol. 74 (2): 137–49. PMID 4689953. doi:10.1016/0022-2836(73)90103-4.
- 1 2 3 4 5 Chen F, Kan H, Castranova V (2010). "Methylation of Lysine 9 of Histone H3: Role of Heterochromatin Modulation and Tumorigenesis". In Tollefsbol TO. Handbook of Epigenetics: The New Molecular and Medical Genetics. Boston: Academic Press. pp. 149–157. ISBN 0-12-375709-6. doi:10.1016/B978-0-12-375709-8.00010-1.
- ↑ Espino PS, Drobic B, Dunn KL, Davie JR (April 2005). "Histone modifications as a platform for cancer therapy". J. Cell. Biochem. 94 (6): 1088–102. PMID 15723344. doi:10.1002/jcb.20387.
- ↑ Hamamoto R, Furukawa Y, Morita M, Iimura Y, Silva FP, Li M, Yagyu R, Nakamura Y (August 2004). "SMYD3 encodes a histone methyltransferase involved in the proliferation of cancer cells". Nat. Cell Biol. 6 (8): 731–40. PMID 15235609. doi:10.1038/ncb1151.
Further reading
- Trievel RC (2004). "Structure and function of histone methyltransferases". Crit. Rev. Eukaryot. Gene Expr. 14 (3): 147–69. PMID 15248813. doi:10.1615/CritRevEukaryotGeneExpr.v14.i3.10.
- Conde F, Refolio E, Cordón-Preciado V, Cortés-Ledesma F, Aragón L, Aguilera A, San-Segundo PA (June 2009). "The Dot1 histone methyltransferase and the Rad9 checkpoint adaptor contribute to cohesin-dependent double-strand break repair by sister chromatid recombination in Saccharomyces cerevisiae". Genetics. 182 (2): 437–46. PMC 2691753
. PMID 19332880. doi:10.1534/genetics.109.101899.
External links
- GeneReviews/NCBI/NIH/UW entry on Kleefstra Syndrome
- Histone-Lysine N-Methyltransferase at the US National Library of Medicine Medical Subject Headings (MeSH)
- Protein-Arginine N-Methyltransferase at the US National Library of Medicine Medical Subject Headings (MeSH)