Choline
![]() | |
![]() | |
Names | |
---|---|
Preferred IUPAC name
2-Hydroxy-N,N,N-trimethylethan-1-aminium | |
Other names
2-Hydroxy-N,N,N-trimethylethanaminium Bilineurine (2-Hydroxyethyl)trimethylammonium | |
Identifiers | |
3D model (JSmol) |
|
1736748 | |
ChEBI | |
ChemSpider | |
DrugBank | |
ECHA InfoCard | 100.000.487 |
EC Number | 200–655–4 |
324597 | |
KEGG | |
PubChem CID |
|
UNII | |
| |
| |
Properties | |
C5H14NO+ | |
Molar mass | 104.17080 |
Density | 1.09 g/ml |
Boiling point | 305 °C (581 °F; 578 K) |
500 mg/ml | |
Except where otherwise noted, data are given for materials in their standard state (at 25 °C [77 °F], 100 kPa). | |
![]() ![]() ![]() | |
Infobox references | |
Choline /ˈkoʊliːn/[1][2] is a water-soluble vitamin-like essential nutrient.[3][4][5] The term cholines refers to the class of quaternary ammonium salts containing the N,N,N-trimethylethanolammonium cation (X− on the right denotes an undefined counteranion).[3]
The cation appears in the head groups of phosphatidylcholine and sphingomyelin, two classes of phospholipid that are abundant in cell membranes. Choline is the precursor molecule for the neurotransmitter acetylcholine, which is involved in many functions including memory and muscle control.
Some animals cannot produce choline, but must consume it through their diet to remain healthy. Humans make choline in the liver. Whether dietary or supplemental choline is beneficial or harmful to humans has not been determined.[6] Possible benefits include reducing the risk of neural tube defects and fatty liver disease. It has also been found that intake of choline during pregnancy can have long-term beneficial effects on memory for the child.[5]
According to the US Institute of Medicine, there is not enough evidence to establish a Recommended Daily Intake for choline. The Australian and New Zealand national nutrition bodies note that while deficiency has been seen during experiments, there have been no reports of choline deficiency in the general population.[7] All three have published an "Adequate Intake" value, discussed below. The European Union's food safety authority says there are no Recommended Daily Intakes in the EU and "no indications of inadequate choline intakes available in the EU".[8]
Methionine and folate are known to interact with choline while homocysteine is undergoing methylation to produce methionine. Recent studies have shown that choline deficiency may have adverse effects, even when sufficient amounts of methionine and folate are present.[5]
History
Choline was first isolated by Adolph Strecker from pig and ox bile (Greek: χολή, chole) in 1862.[9] When it was first chemically synthesized by Oscar Liebreich in 1865,[9] it was known as neurine until 1898 when it was shown to be chemically identical to choline.[10] In 1998, choline was classified as an essential nutrient by the Food and Nutrition Board of the Institute of Medicine (USA).[11]
Chemistry
Choline is a quaternary ammonium salt with the chemical formula (CH3)3N+(CH2)2OHX−, where X− is a counterion such as chloride (see choline chloride), hydroxide or tartrate. Choline chloride can form a low-melting deep eutectic solvent mixture with urea with unusual properties.[12] The salicylate salt is used topically for pain relief of aphthous ulcers.[13][14]
Choline hydroxide
Choline hydroxide is one of the class of phase transfer catalysts that are used to carry the hydroxide ion into organic systems, and, therefore, is considered a strong base. It is the least-costly phase transfer catalyst, and is used as an effective method of stripping photoresists in circuit boards.[15] Choline hydroxide is not completely stable, and it slowly breaks down into trimethylamine.[16]
In humans
Physiology
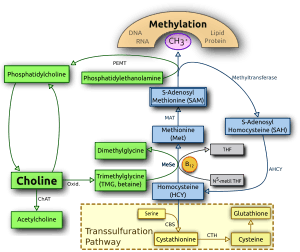
Choline and its metabolites are needed for three main physiological purposes: structural integrity and signaling roles for cell membranes, cholinergic neurotransmission (acetylcholine synthesis), and a major source for methyl groups via its metabolite, trimethylglycine (betaine), which participates in the S-adenosylmethionine (SAMe) synthesis pathways.[17][18]
Fish odor syndrome
Choline is a precursor to trimethylamine, which some persons are not able to break down due to a genetic disorder called trimethylaminuria. Persons suffering from this disorder may suffer from a strong fishy or otherwise unpleasant body odor, due to the body's release of odorous trimethylamine. A body odor will occur even on a normal diet – i.e., one that is not particularly high in choline. Persons with trimethylaminuria are advised to restrict the intake of foods high in choline; this may help to reduce the sufferer's body odor.[19]
Food sources of choline
The following are choline values for a selection of foods in quantities that people may consume in a day.
Animal and plant foods | Food amount (imperial) | Food amount (metric) | Choline (mg) | Choline (mg) in 100g food | Calories | % of diet to meet AI (smaller is better)[a 1] |
---|---|---|---|---|---|---|
Raw beef liver | 5 ounces | 142g | 473 | 333 | 192 [nb 1] | 9 |
Cauliflower | 1 pound | 454g | 177 | 39 | 104 [nb 2] | 13 |
Large egg | 1 | 50g | 147 | 294 | 78 [nb 3] | 12 |
Broccoli | 1 pound | 454g | 182 | 40 | 158 [nb 4] | 19 |
Cod fish | 0.5 pound | 227g | 190 | 84 | 238 [nb 5] | 28 |
Spinach | 1 pound | 454g | 113 | 25 | 154 [nb 6] | 30 |
Wheat germ | 1 cup | 113g | 202 | 179 | 432 [nb 7] | 47 |
Soybeans, mature, raw | 1 cup | 186g | 216 | 116 | 86 [nb 8] | 51 |
Milk, 1% fat | 1 quart | 946mL (976g) | 173 | 18 | 410 [nb 9] | 52 |
Firm tofu | 2 cups | 504g | 142 | 28 | 353 [nb 10] | 55 |
Chicken | 0.5 pound | 227g | 150 | 66 | 543 [nb 11] | 80 |
Cooked kidney beans | 2 cups | 354g | 108 | 31 | 450 [nb 12] | 92 |
Uncooked quinoa | 1 cup | 170g | 119 | 70 | 626 [nb 13] | 116 |
Peanuts | 1 cup | 146g | 77 | 53 | 828 [nb 14] | 237 |
Almonds | 1 cup | 143g | 74 | 52 | 822 [nb 15] | 244 |
Besides cauliflower, other cruciferous vegetables may also be good sources of choline.[20]
The USDA Nutrients Database has choline content for many foods.
Adequate Intake instead of Recommended Dietary Allowance
The Australian, New Zealand, and European Union national nutrition bodies note there have been no reports of choline deficiency in the general population.[21][8] The USA joins these three in not publishing a Recommended Dietary Allowance (RDA), due to lack of consensus evidence. They have, however, published Adequate Intake (AI) values. The US Institute of Medicine (IOM) notes that these figures are based on just one study and that there was little data and the choline made by the body (assuming a dietary intake of zero) may be enough for some groups.[22] Australia, New Zealand and Canada use the figures published by the US IOM. The European Food Safety Authority (EFSA) published its own Adequate Intake values, most recently in 2016.[8][23] For U.S. dietary supplement labeling purposes the amount in a serving is expressed as a percent of Daily Value (%DV) based on the AI. For choline, as shown in Reference Daily Intake, 100% of the Daily Value is 550 mg.
Group | EFSA Adequate Intake | US IOM Adequate Intake | US IOM Upper Limit |
---|---|---|---|
Infants | (mg/day) | (mg/day) | (mg/day) |
0–6 months | - | 125 | ND |
7–12 months | 160 | 150 | ND |
Children | |||
1–3 yrs | 140 | 200 | 1000 |
4–6 yrs | 170 | 250 | 1000 |
7–8 yrs | 250 | 250 | 1000 |
9–10 yrs | 250 | 375 | 1000 |
11–13 yrs | 340 | 375 | 2000 |
Males | |||
14 yrs | 340 | 550 | 3000 |
15+ yrs | 400 | 550 | 3500 (3000 for 15–18 yrs) |
Females | |||
14 yrs | 340 | 400 | 3000 |
15–18 yrs | 400 | 400 | 3000 |
19+ yrs | 400 | 425 | 3500 |
If pregnant | 480 | 450 | 3500 (3000 if ≤ 18 yrs) |
If lactating | 520 | 550 | 3500 (3000 if ≤ 18 yrs) |
Average or common intakes
Studies on a number of different populations have found that the average intake of choline was below the Adequate Intake.[5][24][25] In the United States, a recently published government survey on food consumption reported that for men and women ages 20 and older the average choline intakes were, respectively, 402 and 278 mg/day. For post-menopausal women, 280 mg/day[26]
Health effects of dietary choline
Choline deficiency may play a role in liver disease, atherosclerosis, and possibly neurological disorders.[5] One sign of choline deficiency is an elevated level of the liver enzyme ALT.[4] If low choline intake causes an elevated homocysteine level, it raises the risk for preeclampsia, premature birth, and very low birth weight.[5]
Involvement of choline in long-term health and development of clinical disorders, such as cardiovascular diseases, cognitive decline in aging and regulation of blood lipid levels, has not been well-defined.[27]
As a dietary supplement
The US Food and Drug Administration requires that infant formula not made from cow's milk be supplemented with choline.[28] Choline-providing dietary supplement ingredients include: choline chloride, choline bitartrate, citicoline (CDP-choline), L-alpha-glycerophosphocholine (Alpha-GPC), lecithin, phosphatidylcholine.
Medical imaging
Because of its involvement in biologic processes, several medical imaging techniques have been developed to monitor relative concentration and kinetics of choline in different diseases. Radiolabeling one of the nitrogen methyl groups on choline with carbon-11 allows it to be used as a tracer in PET imaging studies. Carbon-11 is radioactive with a half-life of 20.38 minutes.[29] By monitoring the gamma radiation resulting from the decay of carbon-11, the uptake and retention of carbon-11 choline in different parts of the body can be measured.
One of the first uses of carbon-11 choline in PET imaging was with Alzheimer's disease patients.[30] Choline is the precursor to neurotransmitter acetylcholine whose cholinergic activity is impaired in many neurodegenerative diseases including Alzheimer’s.[31] While there was uptake of the tracer in the brain, no pharmacokinetic pattern was found.
Carbon-11 choline has found more success in cancer systems imaging. Choline is a precursor for the synthesis of phospholipids in our cells.[32] When a cell is about to divide, it synthesizes these phospholipids to generate enough material to build the cell membranes of the two daughter cells. Thus it was hypothesized that highly proliferative tumors would uptake more choline than the surrounding healthy tissue. This was first tested in brain tumors after successful demonstration of choline uptake in the brain.[33] It was found that these brain tumors had over 10x the uptake of carbon-11 choline than the surrounding brain tissue. Furthermore, because of the low choline uptake in healthy brain tissue, carbon-11 choline was found to be a superior PET tracer than fluorine-18 Fludeoxyglucose (FDG) when delineating brain tumors.[34] Carbon-11 choline has also been used to detect tumors in the colon[35] and esophagus[36] and lung metastases.[37]
Prostate cancer is another disease where carbon-11 choline PET imaging has found success. As with the brain, there is too much signal from the surrounding tissue, especially the bladder, to accurately measure tumor uptake with fluorine-18 FDG. While it was shown carbon-11 choline could be used to detect the initiation of prostate cancer,[38] its value was found in detecting prostate cancer recurrence when it is the most deadly.[39][40][41][42] In 2012, the U.S. Food and Drug Administration approved choline carbon-11 choline as an imaging agent to be used during a PET scan to detect recurrent prostate cancer.[43][44]
Pregnancy and brain development
The human body can produce choline by methylation of phosphatidylethanolamine by N-methyltranferase (PEMT) to form phosphatidylcholine in the liver, or it may be consumed from the diet. It has been demonstrated that both de novo production and dietary consumption are necessary, as humans eating diets lacking choline develop fatty liver, liver damage, and muscle damage. However, because of the close interplay between choline, folate, methionine, and vitamin B12, (whose pathways overlap), the function of choline can be complex.
To begin with, methionine can be formed two ways, either from methyl groups derived from folate, or from methyl groups derived from betaine (which gets its methyl groups from choline). Changes in one of these pathways is compensated for by the other, and if these pathways do not adequately supply methyl groups to produce methionine, the precursor to methionine, homocysteine, rises.
Choline in food exists in either a free or esterified form (choline bound within another compound, such as phosphatidylcholine, through an ester linkage). Although all forms are most likely usable, some evidence indicates they are unequally bioavailable (able to be used by the body). Lipid-soluble forms (such as phosphytidylcholine) bypass the liver once absorbed, while water-soluble forms (such as free choline) enter the liver portal circulation and are generally absorbed by the liver.[45] Both pregnancy and lactation increase demand for choline dramatically. This demand may be met by upregulation of PEMT via increasing estrogen levels to produce more choline de novo, but even with increased PEMT activity, the demand for choline is still so high that bodily stores are generally depleted. This is exemplified by the observation that Pemt -/- mice (mice lacking functional PEMT) will abort at 9–10 days unless fed supplemental choline.[46]
While maternal stores of choline are depleted during pregnancy and lactation, the placenta accumulates choline by pumping choline against the concentration gradient into the tissue, where it is then stored in various forms, most interestingly as acetylcholine, (an uncommon occurrence outside of neural tissue). The fetus itself is exposed to a very high choline environment as a result, and choline concentrations in amniotic fluid can be ten times higher than in maternal blood. This high concentration is assumed to allow choline to be abundantly available to tissues and cross the blood-brain barrier effectively.[46]
Functions in the fetus
Choline is in high demand during pregnancy as a substrate for building cellular membranes, (rapid fetal and mother tissue expansion), increased need for one-carbon moieties (a substrate for addition of methylation to DNA and other functions), raising choline stores in fetal and placental tissues, and for increased production of lipoproteins (proteins containing "fat" portions).[47][48][49] In particular, there is interest in the impact of choline consumption on the brain. This stems from choline's use as a material for making cellular membranes, (particularly in making phosphatidylcholine). Human brain growth is most rapid during the third trimester of pregnancy and continues to be rapid to approximately five years of age.[50] During this time, the demand is high for sphingomyelin, which is made from phosphytidyl choline (and thus from choline), because this material is used to myelinate (insulate) nerve fibers.[51] Choline is also in demand for the production of the neurotransmitter acetylcholine, which can influence the structure and organization of brain regions, neurogenesis, myelination, and synapse formation. Acetylcholine is even present in the placenta and may help control cell proliferation/differentiation (increases in cell number and changes of multiuse cells into dedicated cellular functions) and parturition.[52][53]
Choline uptake into the brain is controlled by a low-affinity transporter located at the blood-brain barrier.[54] Transport occurs when arterial plasma choline concentrations increase above 14 μmol/l, which can occur during a spike in choline concentration after consuming choline-rich foods. Neurons, conversely, acquire choline by both high- and low-affinity transporters. Choline is stored as membrane-bound phosphytidylcholine, which can then be used for acetylcholine neurotransmitter synthesis later. Acetylcholine is formed as needed, travels across the synapse, and transmits the signal to the following neuron. Afterwards, acetylcholinesterase degrades it, and the free choline is taken up by a high-affinity transporter into the neuron again.[55]
Neural tube closure
While folate is most well known for preventing neural tube nonclosure (the basis for its addition to prenatal vitamins), folate and choline metabolism are interrelated. Both choline and folate (with the help of vitamin B12) can act as methyl donors to homocysteine to form methionine, which can then go on to form SAM (S-Adenosyl methionine) and act as a methyl donor for methylation of DNA. Dietary choline deficiency alone without concurrent folate deficiency can decrease SAM concentration, suggesting that both folate and choline are important sources of methyl groups for SAM production.[46] Inhibition of choline absorption and use is associated with neural-tube defects in mice, and this may also occur in humans.[45] A retrospective case control study (a study that collects data after the fact, from cases occurring without the investigator causing them to occur) of 400 cases and 400 controls indicated that women with the lowest daily choline intake had a four-fold greater risk of having a child with a neural-tube defect than women in the highest quartile of intake.[25]
Choline and long-term memory
In humans, the brain continues to develop after birth, and does not become similar to its adult structure until around four years of age. By feeding infants formula instead of milk, and presumably through differences in choline amount in the breast milk of mothers consuming different choline levels, the still-developing brain of an infant may be impacted, which may, in part, contribute to the differences seen between individual adult humans in memory and recall.[46]
Choline and lactation
The human mammary gland is composed of several cell types, including adipose (fat cells), muscle, ductal epithelium, and mammary epithelium (referred to sometimes as lactocytes). The mammary epithelium is the site for excretion of raw materials into the milk supply, including choline. This occurs, for the fat portion of the milk, by apocrine secretion, where vacuoles containing materials bud off the cell into the lumen (storage) of the alveolus (milk secretion gland). From here the milk will be released upon stimulation with oxytocin via suckling.[56]
Choline in milk
Choline can be found in milk as free choline, phosphocholine, glycerophosphocholine, sphingomyelin, and phosphatidylcholine, and choline levels within breast milk are correlated with choline levels in maternal blood.[57][58] Choline consumed via breast milk has been shown to impact blood levels of choline in breast-fed infants – indicating that choline consumed in breast milk is entering the neonatal system.[58] Choline may enter the milk supply either directly from the maternal blood supply, or choline-containing nutrients may be produced within the mammary epithelium.[59] Choline reaches the milk through a transporter specific for choline from the maternal blood supply (against a concentration gradient) into the mammary epithelial cells.[60] At high concentrations (greater than that typically seen in humans), choline can diffuse across the cell membrane into the mammary epithelium cell. At more normal concentrations, it passes via what is believed to be a calcium/sodium-dependent, phosphorylation-related, active transporter into the cell.[61]
Differences between breast milk and formula
Human milk is rich in choline, but formulas derived from other sources, particularly soy, have lower total choline concentrations than human milk.[46][62][63] Bovine milk and bovine-derived formulas had similar or higher glycerophosphocholine compared to human milk, and soy-derived formulas had lower glycerophosphocholine content. Phosphatidylcholine and sphingomyelin concentrations were similar between bovine formulas and human milk, but soy-derived infant formulas had more phosphatidylcholine than human or bovine sources. Soy-derived formulas had less sphingomyelin than human milk, which is a concern, since sphingomyelin is used for producing myelin, which insulates neurons. Free choline concentrations in mature human milk were 30–80% lower than those found in bovine milk or formulas. Mature human milk also has lower free choline than colostrum-transitional human milk. Phosphocholine is particularly abundant in human milk.[64]
Additional images
- Choline (C5H14NO+)
- Choline hydroxide
See also
Notes
- ↑ Percentage of diet calculated using 2500 daily calories for an average adult male and 550mg for Adequate Intake (AI) using the figures from the table. As an example, even though peanuts contain some choline, they are a poor source given their 237% of diet to meet AI requirement, which means that a person who ate nothing but peanuts would have less than half of AI. Nearly identical figures are obtained for an average adult female when using 2000 calories and 425mg as assumptions; therefore, separate columns for male and female are not necessary.
References
- ↑ Oxford Dictionaries definition for Choline
- ↑ Merriam-Webster definition of Choline
- 1 2 "Choline". Human Metabolome Database. The Metabolomics Innovation Centre, University of Alberta, Edmonton, Canada. 17 August 2016. Retrieved 13 September 2016.
- 1 2 "Choline". Micronutrient Information Center, Linus Pauling Institute, Oregon State University, Corvallis, Oregon. February 2015. Retrieved 10 January 2017.
- 1 2 3 4 5 6 Zeisel SH; da Costa KA (November 2009). "Choline: an essential nutrient for public health". Nutrition Reviews. 67 (11): 615–23. PMC 2782876
. PMID 19906248. doi:10.1111/j.1753-4887.2009.00246.x.
- ↑ Leermakers, E. T.; Moreira, E. M.; Kiefte-De Jong, J. C.; Darweesh, S. K.; Visser, T; Voortman, T; Bautista, P. K.; Chowdhury, R; Gorman, D; Bramer, W. M.; Felix, J. F.; Franco, O. H. (2015). "Effects of choline on health across the life course: A systematic review". Nutrition Reviews. 73 (8): 500–22. PMID 26108618. doi:10.1093/nutrit/nuv010.
- ↑ Nutrient Reference Values for Australia and New Zealand
- 1 2 3 "Scientific Opinion on the substantiation of health claims related to choline and contribution to normal lipid metabolism (ID 3186), maintenance of normal liver function (ID 1501), contribution to normal homocysteine metabolism (ID 3090), maintenance of normal neurological function (ID 1502), contribution to normal cognitive function (ID 1502), and brain and neurological development (ID 1503) pursuant to Article 13(1) of Regulation (EC) No 1924/2006". EFSA Journal. 9 (4): 2056. 2011. doi:10.2903/j.efsa.2011.2056.
The Panel notes that no dietary reference values for choline have been established in the EU. There are no reliable intake data and there are no indications of inadequate choline intakes available in the EU
- 1 2 Zeisel, Steven H. (2012). "A Brief History of Choline". Annals of Nutrition and Metabolism. 61 (3): 254–258. doi:10.1159/000343120.
- ↑ Boldyrev, A. A. (14 April 2012). "Carnosine: New concept for the function of an old molecule". Biochemistry (Moscow). 77 (4): 313–326. doi:10.1134/S0006297912040013.
- ↑ "Choline Overview". CholineInfo.org. Retrieved 6 January 2012.
- ↑ Andrew P. Abbott; Glen Capper; David L. Davies; Raymond K. Rasheed; Vasuki Tambyrajah (2003). "Novel solvent properties of choline chloride/urea mixtures". Chemical Communications (1): 70–71. doi:10.1039/b210714g.
- ↑ Gastroenterology eu.elsevierhealth.com. Retrieved 15 November 2012. Gastroenterology. Chapter 6. page 128
- ↑ Choline salicylate/magnesium salicylate - oral, Trilisate medicinenet.com. Retrieved 15 November 2012
- ↑ Sedlak, Rudy (2009). "The Technology of Photoresist Stripping". Retrieved 27 November 2013.
- ↑ GuideChem http://www.guidechem.com/products/123-41-1.html
- ↑ Glier, Melissa B.; Green, Timothy J.; Devlin, Angela M. (2014). "Methyl nutrients, DNA methylation, and cardiovascular disease". Molecular Nutrition & Food Research. 58: 172–182. doi:10.1002/mnfr.201200636.
- ↑ "Dietary Betaine Promotes Generation of Hepatic S-Adenosylmethionine and Protects the Liver from Ethanol-Induced Fatty Infiltration" (June 1993) Alcoholism: Clinical and Experimental Research Volume 17, Issue 3, Pages: 552–555, Anthony J. Barak, Harriet C. Beckenhauer, Matti Junnila and Dean J. Tuma
- ↑ Mitchell SC, Smith RL (2001). "Trimethylaminuria: the fish malodor syndrome". Drug Metab Dispos. 29 (4 Pt 2): 517–21. PMID 11259343.
- ↑ Gossell-Williams M, Fletcher H, McFarlane-Anderson N, Jacob A, Patel J, Zeisel S (December 2005). "Dietary intake of choline and plasma choline concentrations in pregnant women in Jamaica". The West Indian Medical Journal. 54 (6): 355–9. PMC 2438604
. PMID 16642650. doi:10.1590/s0043-31442005000600002.
- ↑ Nutrient Reference Values for Australia and New Zealand,
Although choline is essential, there appear to have been no reports of deficiency in the general population. Deficiencies have been seen in experimental situations and also in total parenteral nutrition (Buchman et al. 1992, 1993, 1995, Chalwa et al. 1989, Shapira et al. 1986, Sheard et al. 1986).
- ↑ Choline. IN: Dietary Reference Intakes for Thiamin, Riboflavin, Niacin, Vitamin B6, Folate, Vitamin B12, Pantothenic Acid, Biotin, and Choline. National Academy Press. 2001, PP. 390-422.
- ↑ "Dietary reference values: EFSA publishes advice on choline". European Food Safety Authority, Parma, Italy. 17 August 2016. Retrieved 10 January 2017.
- ↑ Bidulescu A, Chambless LE, Siega-Riz AM, Zeisel SH, Heiss G (2009). "Repeatability and measurement error in the assessment of choline and betaine dietary intake: the Atherosclerosis Risk in Communities (ARIC) study". Nutrition Journal. 8 (1): 14. PMC 2654540
. PMID 19232103. doi:10.1186/1475-2891-8-14.
- 1 2 Shaw, GM; Carmicheal, SL; Yang, W; Selvin, S; Schaffer, DM (2004). "Periconceptional dietary intake of choline and betain and neural tube defects in offspring". Am J Epidemiol. 160 (2): 102–9. PMID 15234930. doi:10.1093/aje/kwh187.
- ↑ What We Eat In America, NHANES 2013-2014.
- ↑ Leermakers, Elisabeth T.M.; Moreira, Eduardo M.; Kiefte-De Jong, Jessica C.; Darweesh, Sirwan K.L.; Visser, Thirsa; Voortman, Trudy; Bautista, Paula K.; Chowdhury, Rajiv; Gorman, Donal; Bramer, Wichor M.; Felix, Janine F.; Franco, Oscar H. (2015). "Effects of choline on health across the life course: A systematic review". Nutrition Reviews. 73 (8): 500. PMID 26108618. doi:10.1093/nutrit/nuv010.
- ↑ "21 CFR 107.100: Infant formula; Nutrient requirements; Nutrient specifications; Choline content". Code of Federal Regulations, Title 21; Food and Drug Administration. 1 April 2016. Retrieved 20 December 2016.
- ↑ "appendix C - Decay Characteristics of Some Medically Important Radionuclides". Physics in Nuclear Medicine (Fourth Edition) (PDF). W.B. Saunders. pp. 449–475. ISBN 9781416051985.
- ↑ "XX Canadian Congress of Neurological Sciences Abstracts of the Scientific Program". Canadian Journal of Neurological Sciences. 12 (2): 169–220. 1 May 1985. ISSN 0317-1671. doi:10.1017/S031716710004703X.
- ↑ Schliebs, R; Arendt, T (10 August 2011). "The cholinergic system in aging and neuronal degeneration.". Behavioural Brain Research. 221 (2): 555–63. PMID 21145918. doi:10.1016/j.bbr.2010.11.058.
- ↑ Zeisel, SH (1981). "Dietary choline: biochemistry, physiology, and pharmacology.". Annual review of nutrition. 1: 95–121. PMID 6764726. doi:10.1146/annurev.nu.01.070181.000523.
- ↑ Hara, T; Kosaka, N; Shinoura, N; Kondo, T (June 1997). "PET imaging of brain tumor with [methyl-11C]choline.". Journal of nuclear medicine : official publication, Society of Nuclear Medicine. 38 (6): 842–7. PMID 9189127.
- ↑ Ohtani, T; Kurihara, H; Ishiuchi, S; Saito, N; Oriuchi, N; Inoue, T; Sasaki, T (November 2001). "Brain tumour imaging with carbon-11 choline: comparison with FDG PET and gadolinium-enhanced MR imaging.". European journal of nuclear medicine. 28 (11): 1664–70. PMID 11702108. doi:10.1007/s002590100620.
- ↑ Terauchi, T; Tateishi, U; Maeda, T; Kanou, D; Daisaki, H; Moriya, Y; Moriyama, N; Kakizoe, T (October 2007). "A case of colon cancer detected by carbon-11 choline positron emission tomography/computed tomography: an initial report.". Japanese journal of clinical oncology. 37 (10): 797–800. PMID 17989097. doi:10.1093/jjco/hym102.
- ↑ Kobori, O; Kirihara, Y; Kosaka, N; Hara, T (1 November 1999). "Positron emission tomography of esophageal carcinoma using (11)C-choline and (18)F-fluorodeoxyglucose: a novel method of preoperative lymph node staging.". Cancer. 86 (9): 1638–48. PMID 10547535. doi:10.1002/(sici)1097-0142(19991101)86:9<1638::aid-cncr4>3.0.co;2-u.
- ↑ Hara, T; Inagaki, K; Kosaka, N; Morita, T (September 2000). "Sensitive detection of mediastinal lymph node metastasis of lung cancer with 11C-choline PET.". Journal of nuclear medicine : official publication, Society of Nuclear Medicine. 41 (9): 1507–13. PMID 10994730.
- ↑ Hara, T; Kosaka, N; Kishi, H (June 1998). "PET imaging of prostate cancer using carbon-11-choline.". Journal of nuclear medicine : official publication, Society of Nuclear Medicine. 39 (6): 990–5. PMID 9627331.
- ↑ Scattoni, V; Picchio, M; Suardi, N; Messa, C; Freschi, M; Roscigno, M; Da Pozzo, L; Bocciardi, A; Rigatti, P; Fazio, F (August 2007). "Detection of lymph-node metastases with integrated [11C]choline PET/CT in patients with PSA failure after radical retropubic prostatectomy: results confirmed by open pelvic-retroperitoneal lymphadenectomy.". European Urology. 52 (2): 423–9. PMID 17397992. doi:10.1016/j.eururo.2007.03.032.
- ↑ Rinnab, L; Mottaghy, FM; Simon, J; Volkmer, BG; de Petriconi, R; Hautmann, RE; Wittbrodt, M; Egghart, G; Moeller, P; Blumstein, N; Reske, S; Kuefer, R (2008). "[11C]Choline PET/CT for targeted salvage lymph node dissection in patients with biochemical recurrence after primary curative therapy for prostate cancer. Preliminary results of a prospective study.". Urologia internationalis. 81 (2): 191–7. PMID 18758218. doi:10.1159/000144059.
- ↑ Reske, SN; Blumstein, NM; Glatting, G (January 2008). "[11C]choline PET/CT imaging in occult local relapse of prostate cancer after radical prostatectomy.". European journal of nuclear medicine and molecular imaging. 35 (1): 9–17. PMID 17828534. doi:10.1007/s00259-007-0530-2.
- ↑ Mitchell, Christopher; Kwon, Eugene; Lowe, Val; Hung, Joseph; Rangel, Laureano; Karnes, R. Jeffrey (April 2012). "2039 IMPACT OF 11C-CHOLINE PET/CT SCAN ON DETECTION OF RECURRENT PROSTATE CANCER IN MEN WITH BIOCHEMICAL RECURRENCE FOLLOWING FAILED INITIAL TREATMENT". The Journal of Urology. 187 (4): e823. doi:10.1016/j.juro.2012.02.2203.
- ↑ "FDA approves production of imaging agent that helps detect prostate cancer". U.S. Food and Drug Administration, Silver Spring, MD. 12 September 2012. Retrieved 9 January 2017.
- ↑ "Choline C-11". Drugs.com. 3 November 2016. Retrieved 9 January 2017.
- 1 2 Zeisel, SH (2006). "The fetal origins of memory: the role of dietary choline in optimal brain development". J Pediatr. 149 (5): S131–S136. PMC 2430654
. PMID 17212955. doi:10.1016/j.jpeds.2006.06.065.
- 1 2 3 4 5 Zeisel, SH (2006). "Choline: critical role during fetal development and dietary requirements in adults". Annu. Rev. Nutr. 26: 229–50. PMC 2441939
. PMID 16848706. doi:10.1146/annurev.nutr.26.061505.111156.
- ↑ Institute of Medicine, Food and Nutrition Board. Dietary reference intakes for Thiamine, Riboflavin, Niacin, Bitamin B6, Folate, Vitamin B12, Pantothenic Acid, Biotin and Choline. Washington, DC: National Academies Press;1998
- ↑ Allen LH. Pregnancy and lactation In: Bowman BA, Russle RM, eds. Present Knowledge in Nutrition. Washington DC: ILSI Press; 2006: 529–543
- ↑ King, JC (2000). "Physiology of pregnancy and nutrient metabolism". AM J Clin Nutr. 71: 1218S–1225S.
- ↑ Morgane, PJ; Mokler, DJ; Galler, JR (2002). "Effects of prenatal protein malnutrition on the hippocampal formation". Neurosci Biobehav Rev. 26 (4): 471–483. doi:10.1016/s0149-7634(02)00012-x.
- ↑ Oshida, K; Shimizu, T; Takase, M; Tamura, Y; Shimizu, T; Yamashiro, Y (2003). "Effects of dietary sphingomyelin on central nervous system myelination in developing rats". Peditr Res. 53: 589–593.
- ↑ Sastry, BV (1997). "Human placental cholinergic system". Biochem Pharmacol. 53: 1577–1586.
- ↑ Sastry, BV; Sadavongvivad, C (1978). "Cholinergic systems in non-nervous tissues". Pharmacol Rev. 30: 65–132.
- ↑ Lockman, P. R.; Allen, D. D. (2002). "The transport of choline". Drug Development and Industrial Pharmacy. 28 (7): 749–71. PMID 12236062. doi:10.1081/DDC-120005622.
- ↑ Caudill, M (2010). "Pre and Postnatal Health: evidence of increased choline needs". American Dietetic Association. 110 (8): 1198–1206. doi:10.1016/j.jada.2010.05.009.
- ↑ Hale T & Hartmann P. Textbook of Human Lactation. Hale Publishing, 2007; p. 35-44
- ↑ Holmes-McNarry, MQ; Cheng, WL; Mar, MH; Fussell, S; Zeisel, SH (1996). "Choline and choline esters in human and rat milk in infant formulas". Am J Clin Nutr. 64 (4): 572–6. PMID 8839502.
- 1 2 Ilcol, Y.O.; et al. (2005). "Choline status in newborns, infants, children, breast-feeding women, breast-fed infants, and human breast milk". Journal of Nutritional Biochemsitry. 16 (8): 489–499. doi:10.1016/j.jnutbio.2005.01.011.
- ↑ James AR. Hormone Regulation of Choline Uptake and Incorporation in Mouse mammary Gland Explants. Exp Biol Med (Maywood). 2004 Apr;229(4):323–6.
- ↑ Chao, CK; Pomfret, EA; Zeisel, SH (1988). "Uptake of choline by rat mammary gland epithelial cells". Biochem J. 254 (1): 33–8. PMC 1135035
. PMID 3178755. doi:10.1042/bj2540033.
- ↑ Chiao-Kang et al. Uptake of choline by rat mammary-gland epithelial cells. Biochem J. (1988) 254, 33–38.
- ↑ Banapurmath, CR; et al. (1996). "Developing brain and breastfeeding". Indian Pediatrics. 33: 235–38.
- ↑ Tram, TH; et al. (1997). "Sialic acid content of infant saliva: comparison of breast fed with formula fed infants". Archives of Disease in Childhood. 77 (4): 315–318. doi:10.1136/adc.77.4.315.
- ↑ Holmes-McNary, M; Cheng, WL; Mar, MH; Fussel, S; Zeisel, SH. "Choline and choline esters in human and rat milk and infant formulas". Am J Clin Nutr. 1996 (64): 572–6.
References for nutritional data
- ↑ Entry for "Beef, variety meats and by-products, liver, raw"in the USDA Nutrients database Archived 3 March 2015 at the Wayback Machine.
- ↑ Entry for "Cauliflower, cooked, boiled, drained, with salt" in the USDA Nutrients database Archived 3 March 2015 at the Wayback Machine.
- ↑ Entry for one large "Egg, whole, cooked, hard-boiled" in the USDA Nutrients database Archived 3 March 2015 at the Wayback Machine.
- ↑ Entry for "Broccoli, cooked, boiled, drained, with salt" in the Nutritiondata database
- ↑ Entry for "Fish, cod, Atlantic, cooked, dry heat" in the USDA Nutrients database Archived 3 March 2015 at the Wayback Machine.
- ↑ Entry for "Spinach, frozen, chopped or leaf, cooked, boiled, drained, without salt" in the USDA Nutrients database Archived 3 March 2015 at the Wayback Machine.
- ↑ Entry for "Cereals ready-to-eat, wheat germ, toasted, plain" in the USDA Nutrients database Archived 3 March 2015 at the Wayback Machine.
- ↑ Entry for "Soybeans, mature seeds, sprouted, raw" in the USDA Nutrients database Archived 3 March 2015 at the Wayback Machine.
- ↑ Entry for "Milk, lowfat, fluid, 1% milkfat, with added vitamin A and vitamin D" in the USDA Nutrients database Archived 3 March 2015 at the Wayback Machine.
- ↑ Entry for "Tofu, firm, prepared with calcium sulfate and magnesium chloride (nigari) (1)" in the USDA Nutrients database Archived 3 March 2015 at the Wayback Machine.
- ↑ Entry for "Chicken, broilers or fryers, meat and skin, cooked, roasted" in the USDA Nutrients database Archived 3 March 2015 at the Wayback Machine.
- ↑ Entry for "Beans, kidney, all types, mature seeds, cooked, boiled, without salt" in the USDA Nutrients database Archived 3 March 2015 at the Wayback Machine.
- ↑ Entry for "Quinoa, uncooked" in the USDA Nutrients database Archived 3 March 2015 at the Wayback Machine.
- ↑ Entry for "Peanuts, all types, raw" in the USDA Nutrients database Archived 3 March 2015 at the Wayback Machine.
- ↑ Entry for 1 cup whole "Nuts, almonds" in the USDA Nutrients database Archived 3 March 2015 at the Wayback Machine.