DNA re-replication
DNA re-replication (or simply rereplication) is an undesirable and possibly fatal occurrence in eukaryotic cells in which the genome is replicated more than once per cell cycle.[1] Rereplication is believed to lead to genomic instability and has been implicated in the pathologies of a variety of human cancers.[2] To prevent rereplication, eukaryotic cells have evolved multiple, overlapping mechanisms to inhibit chromosomal DNA from being partially or fully rereplicated in a given cell cycle. These control mechanisms rely on cyclin-dependent kinase (CDK) activity.[1] DNA replication control mechanisms cooperate to prevent the relicensing of replication origins and to activate cell cycle and DNA damage checkpoints.[2] DNA rereplication must be strictly regulated to ensure that genomic information is faithfully transmitted through successive generations.
Origin licensing
All known mechanisms that prevent DNA rereplication in eukaryotic organisms inhibit origin licensing.[1] Origin licensing is the preliminary step for normal replication initiation during late G1 and early S phase and involves the recruitment of the pre-replicative complex (pre-RC) to the replication origins. Licensing begins with the binding of the multi-subunit ATPase, the origin recognition complex (ORC), to the DNA at the replication origins.[3] Once bound to chromatin the ORC recruits the AAA+ ATPase Cdc6 and the coiled-coil domain protein Cdt1. Cdt1 binding and the ATPase activity of ORC and Cdc6 facilitate the loading of the minichromosome maintenance (MCM) proteins 2-7 onto the chromatin.[1] The MCM complex is the DNA helicase that opens the helix at the replication origin and unwinds the two strands as the replication forks travel along the DNA.[4] Elevated CDK activity at the end of G1 triggers the firing of the origins and the dismantling of the pre-RCs. High CDK levels, which are maintained until the end of mitosis, inhibit or destroy pre-RC components and prevent the origin from relicensing. A new MCM complex cannot be loaded onto the origin until the pre-RC subunits are reactivated with the decline of CDK activity at the end of mitosis. Thus, CDKs serve a dual role in the regulation of eukaryotic DNA replication: elevated CDK activity initiates replication at the origins and prevents rereplication by inhibiting origin re-licensing.[5][6][7] This ensures that no replication origin fires twice in the same cell cycle.[4]
Two-state model for DNA replication regulation
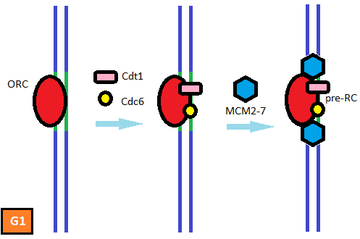

Early experimental evidence on the regulation of DNA replication suggests that replication origins exist in one of two states during the cell cycle: a prereplicative state in G1 and a postreplicative state from the moment of initiation until passage through mitosis.[1] Origins of replication alternate between these two distinct states during the cell cycle.[8] A licensing factor which is required for replication initiation binds to origins in the prereplicative state. At the G1/S transition, the factor is inactivated and cannot be restored until the cell cycle has concluded.[9] The identification and characterization of the ORC, Cdc6, Cdt1, and the MCM complex proteins as the licensing factor gives credence to this model and suggests a means by which the oscillatory nature of CDKs in the cell cycle can regulate rereplication.[1]
Replication regulation
Budding yeast
Rereplication regulation is best understood in budding yeast. Saccharomyces cerevisiae cells prevent rereplication by directly regulating pre-RC assembly through the CDK-mediated phosphorylation of the pre-RC components Cdc6, MCM2-7, and the ORC subunits.[4] The phosphorylation of these components is initiated at the onset of S phase and is maintained throughout the rest of the cell cycle as CDK activity remains high. Phosphorylated Cdc6 is bound by the ubiquitin-protein ligase SCF which leads to its proteolytic degradation. CDK-dependent phosphorylation of the MCM2-7 proteins results in the complex’s export from the nucleus. (Cdt1 which associates with the MCM complex is similarly exported from the nucleus). Phosphorylation of the ORC subunits presumably disrupts the ORC’s ability to bind other pre-RC components.[4] Thus, multiple mechanisms ensure that the pre-RC cannot be reassembled on postreplicative origins.
Note: Since origins fire at different times throughout S phase, it is crucial that the inhibitory mechanisms that prevent new MCM2-7 recruitment do not destabilize existing pre-RCs. Pre-RCs can remain assembled on origins that haven’t fired even though rereplication inhibitory mechanisms are inhibiting or destroying pre-RC components.
Other organisms
Although CDK regulation of pre-RC assembly appears to be highly evolutionarily conserved, some differences across organisms are noted. In multicellular eukaryotes pre-RC assembly is regulated by the anaphase-promoting complex (APC) in addition to CDKs. APC, an E3 enzyme, ubiquitinates the protein geminin and targets it for degradation.[4] Geminin normally prevents origin licensing by binding to and inhibiting Cdt1. In G1, APC activity is adequate to suppress the accumulation of geminin, thereby indirectly promoting pre-RC assembly. At the end of G1, APC is inactivated and geminin can accumulate and prevent origin re-licensing.
Pre-RC regulation in most animals is not well understood.[4]
Consequences of rereplication in eukaryotic cells
Rereplication and mitotic failure are generally not programmed events, but rather result spontaneously from defects in the cell cycle machinery.[1] Rereplication appears to give rise to dsDNA breaks which triggers a DNA damage response and arrests cells in G2.[10] The checkpoint effectively causes a permanent cell cycle arrest and eventual apoptosis.[11]
Rereplication can be experimentally induced by simultaneously disrupting several of the mechanisms that prevent origin re-licensing. For example, deregulation of the ORC, MCM2-7 and Cdc6 mechanisms can induce rereplication in budding yeast cells.[12]
Note: Recent evidence suggests that although overlapping, the multiple replication regulation mechanisms should not be considered as functionally redundant; although a single mechanism may repress rereplication at greater than 99% efficiency, it may not be sufficient to maintain genome stability over many generations.[13] Instead, it is believed that the multiplicative effect of many overlapping mechanisms is what sufficiently prevents rereplication and ensures the faithful transmission of a cell’s genome.
Rereplication in cancer
Rereplication has been implicated in tumorigenesis in model organisms and humans. Replication initiation proteins are overexpressed in tissue samples from several types of human cancers[1][14][15] and experimental overexpression of Cdt1 and Cdc6 can cause tumor development in mouse cells.[16][17][18] Further, these studies indicate that rereplication can result in an increase in aneuploidy, chromosomal fusions, and DNA breaks.[19] A thorough understanding of the regulatory replication mechanisms is important for the development of novel cancer treatments.
Endoreduplication
For the special case of cell cycle-regulated DNA replication in which DNA synthesis is uncoupled from cell cycle progression refer to endoreduplication.
References
- 1 2 3 4 5 6 7 8 Arias, E. E.; Walter, J. C. (2007). "Strength in numbers: Preventing rereplication via multiple mechanisms in eukaryotic cells". Genes & Development. 21 (5): 497–518. PMID 17344412. doi:10.1101/gad.1508907.
- 1 2 Truong, L. N.; Wu, X. (2011). "Prevention of DNA re-replication in eukaryotic cells". Journal of Molecular Cell Biology. 3 (1): 13–22. PMC 3030972
. PMID 21278447. doi:10.1093/jmcb/mjq052.
- ↑ Cvetic, Christin A.; Walter, Johannes C. (2006). "Getting a Grip on Licensing: Mechanism of Stable Mcm2-7 Loading onto Replication Origins". Molecular Cell. 21 (2): 143–4. PMID 16427002. doi:10.1016/j.molcel.2006.01.003.
- 1 2 3 4 5 6 Morgan, David O. (2007). The Cell Cycle: Principles of Control. Oxford University Press. ISBN 0-87893-508-8.
- ↑ Bell, Stephen P.; Dutta, Anindya (2002). "Dna Replication Ineukaryoticcells". Annual Review of Biochemistry. 71: 333–74. PMID 12045100. doi:10.1146/annurev.biochem.71.110601.135425.
- ↑ Broek, D.; Bartlett, R.; Crawford, K.; Nurse, P. (1991). "Involvement of p34cdc2 in establishing the dependency of S phase on mitosis". Nature. 349 (6308): 388–393. PMID 1992340. doi:10.1038/349388a0.
- ↑ Hayles, J.; Fisher, D.; Woollard, A.; Nurse, P. (1994). "Temporal order of S phase and mitosis in fission yeast is determined by the state of the p34cdc2-mitotic B cyclin complex". Cell. 78 (5): 813–822. PMID 8087848. doi:10.1016/S0092-8674(94)90542-8.
- ↑ Diffley, John F.X.; Cocker, Julie H.; Dowell, Simon J.; Rowley, Adele (1994). "Two steps in the assembly of complexes at yeast replication origins in vivo". Cell. 78 (2): 303–16. PMID 8044842. doi:10.1016/0092-8674(94)90299-2.
- ↑ Blow, J. Julian; Laskey, Ronald A. (1988). "A role for the nuclear envelope in controlling DNA replication within the cell cycle". Nature. 332 (6164): 546–8. PMID 3357511. doi:10.1038/332546a0.
- ↑ Green, B. M.; Li, JJ (2004). "Loss of Rereplication Control in Saccharomyces cerevisiae Results in Extensive DNA Damage". Molecular Biology of the Cell. 16 (1): 421–32. PMC 539184
. PMID 15537702. doi:10.1091/mbc.E04-09-0833.
- ↑ Archambault, V.; Ikui, A. E.; Drapkin, B. J.; Cross, F. R. (2005). "Disruption of Mechanisms That Prevent Rereplication Triggers a DNA Damage Response". Molecular and Cellular Biology. 25 (15): 6707–21. PMC 1190345
. PMID 16024805. doi:10.1128/MCB.25.15.6707-6721.2005.
- ↑ Nguyen, Van Q.; Co, Carl; Li, Joachim J. (2001). "Cyclin-dependent kinases prevent DNA re-replication through multiple mechanisms". Nature. 411 (6841): 1068–73. PMID 11429609. doi:10.1038/35082600.
- ↑ Green, B. M.; Morreale, RJ; Ozaydin, B; Derisi, JL; Li, JJ (2006). "Genome-wide Mapping of DNA Synthesis in Saccharomyces cerevisiae Reveals That Mechanisms Preventing Reinitiation of DNA Replication Are Not Redundant". Molecular Biology of the Cell. 17 (5): 2401–14. PMC 1446083
. PMID 16481397. doi:10.1091/mbc.E05-11-1043.
- ↑ Blow, J. Julian; Gillespie, Peter J. (2008). "Replication licensing and cancer — a fatal entanglement?". Nature Reviews Cancer. 8 (10): 799–806. PMC 2577763
. PMID 18756287. doi:10.1038/nrc2500.
- ↑ Gonzalez, Michael A.; Tachibana, Kiku-e K.; Laskey, Ronald A.; Coleman, Nicholas (2005). "Innovation: Control of DNA replication and its potential clinical exploitation". Nature Reviews Cancer. 5 (2): 135–41. PMID 15660109. doi:10.1038/nrc1548.
- ↑ Arentson, Elizabeth; Faloon, Patrick; Seo, Junghee; Moon, Eunpyo; Studts, Joey M; Fremont, Daved H; Choi, Kyunghee (2002). "Oncogenic potential of the DNA replication licensing protein CDT1". Oncogene. 21 (8): 1150–8. PMID 11850834. doi:10.1038/sj.onc.1205175.
- ↑ Liontos, M.; Koutsami, M.; Sideridou, M.; Evangelou, K.; Kletsas, D.; Levy, B.; Kotsinas, A.; Nahum, O.; et al. (2007). "Deregulated Overexpression of hCdt1 and hCdc6 Promotes Malignant Behavior". Cancer Research. 67 (22): 10899–909. PMID 18006835. doi:10.1158/0008-5472.CAN-07-2837.
- ↑ Seo, Junghee; Chung, Yun S; Sharma, Girdhar G; Moon, Eunpyo; Burack, Walter R; Pandita, Tej K; Choi, Kyunghee (2005). "Cdt1 transgenic mice develop lymphoblastic lymphoma in the absence of p53". Oncogene. 24 (55): 8176–86. PMID 16261166. doi:10.1038/sj.onc.1208881.
- ↑ Hook, Sara S; Lin, Jie Jessie; Dutta, Anindya (2007). "Mechanisms to control rereplication and implications for cancer". Current Opinion in Cell Biology. 19 (6): 663–71. PMC 2174913
. PMID 18053699. doi:10.1016/j.ceb.2007.10.007.