Cardiac glycoside
Cardiac glycoside | |
---|---|
Drug class | |
Class identifiers | |
Use | Congestive heart failure |
ATC code | C01A |
Biological target |
Na+ /K+ -ATPase |
External links | |
MeSH | D002301 |
In Wikidata |
Cardiac glycosides are a class of organic compounds that increase the output force of the heart and decrease its rate of contractions by acting on the cellular sodium-potassium ATPase pump.[1] Their beneficial medical uses are as treatments for congestive heart failure and cardiac arrhythmias; however, their relative toxicity prevents them from being widely used.[2] Most commonly found as secondary metabolites in several plants such as foxglove plants, these compounds nevertheless have a diverse range of biochemical effects regarding cardiac cell function and have also been suggested for use in cancer treatment.[3]
Classification
General Structure
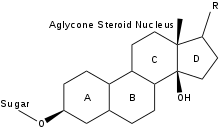
The general structure of a cardiac glycoside consists of a steroid glycoside molecule attached to a sugar and an R group.[4] The steroid nucleus consists of five fused rings to which other functional groups such as methyl, hydroxyl, and aldehyde groups can be attached to influence the overall molecule’s biological activity.[4] Cardiac glycosides also vary in the groups attached at either end of the steroid. Specifically, different sugar groups attached at the sugar end of the steroid can alter the molecule’s solubility and kinetics; however, the lactone moiety at the R group end only serves a structural function.[5]
In particular, the structure of the ring attached at the R end of the molecule allows it to be classified as either a cardenolide or bufadienolide. Cardenolides differ from bufadienolides due to the presence of an “enolide,” a five-membered ring with a single double bond, at the lactone end. Bufadienolides, on the other hand, contain a “dienolide,” a six-membered ring with two double bonds, at the lactone end.[5] While compounds of both groups can be used to influence the cardiac output of the heart, cardenolides are more commonly used medicinally, primarily due to the widespread availability of the plants from which they are derived.
Classification
Cardiac glycosides can be more specifically categorized based on the plant they are derived from, as in the following list. For example, cardenolides have been primarily derived from the foxglove plants Digitalis purpurea and Digitalis lanata, while bufadienolides have been derived from the venom of the cane toad Bufo marinus, from which they receive the “bufo” portion of their name.[6] Below is a full list of plants from which cardiac glycosides can be derived.
Plants from which cardenolides can be derived
- Convallaria majalis (Lily of the Valley): convallotoxin[7]
- Antiaris toxicaria (Upas tree): antiarin
- Strophanthus kombe (Strophanthus vine): ouabain, g/k/e-strophanthin
- Digitalis lanata and Digitalis purpurea (Woolly and purple foxglove): digoxin, digitoxin
- Nerium oleander (Oleander tree): oleandrin
- Asclepias sp. (Milkweed): oleandrin
- Adonis vernalis (Spring pheasant's eye): adonitoxin
Organisms from which bufadienolides can be derived
- Leonurus cardiaca (Motherwort): scillarenin[7]
- Drimia maritima (Squill): proscillaridine A
- Kalanchoe daigremontiana and other Kalanchoe species (Kalanchoe plant): daigremontianin and others
- Bufo marinus (Cane toad): Various bufadienolides
Mechanism of action
Cellular function
Cardiac glycosides affect the sodium-potassium ATPase pump in cardiac muscle cells to alter their function.[1] Normally, these sodium-potassium pumps move potassium ions in and sodium ions out. Cardiac glycosides, however, inhibit this pump by stabilizing it in the E2-P transition state, so that sodium cannot be extruded: intracellular sodium concentration therefore increases. With regards to potassium ion movement, because both cardiac glycosides and potassium compete for binding to the ATPase pump, changes in extracellular potassium concentration can potentially lead to altered drug efficacy.[8] Nevertheless, by carefully controlling the dosage, such adverse effects can be avoided. Continuing on with the mechanism, raised intracellular sodium levels inhibit the function of a second membrane ion exchanger, NCX, which is responsible for pumping calcium ions out of the cell and sodium ions in at a ratio of 3Na/Ca. Thus, calcium ions are also not extruded and will begin to build up inside the cell as well.[9][10]
The disrupted calcium homeostasis and increased cytoplasmic calcium concentrations cause increased calcium uptake into the sarcoplasmic reticulum (SR) via the SERCA2 transporter. Raised calcium stores in the SR allow for greater calcium release on stimulation, so the myocyte can achieve faster and more powerful contraction by cross-bridge cycling.[1] The refractory period of the AV node is increased, so cardiac glycosides also function to decrease heart rate. For example, the ingestion of digoxin leads to increased cardiac output and decreased heart rate without significant changes in heart rate or blood pressure; this quality allows it to be widely used medicinally in the treatment of cardiac arrhythmias.[1]
Clinical significance
Cardiac glycosides have long served as the main medical treatment to congestive heart failure and cardiac arrhythmia, due to their effects of increasing the force of muscle contraction while reducing heart rate. Heart failure is characterized by an inability to pump enough blood to support the body, possibly due to a decrease in the volume of the heart or its contractile force.[11] Treatments for the condition thus focus on lowering blood pressure, so that the heart does not have to exert as much force to pump the blood, or directly increasing the heart's contractile force, so that the heart can overcome the higher blood pressure. Cardiac glycosides, such as the commonly used digoxin and digitoxin, deal with the latter, due to their positive inotropic activity. On the other hand, cardiac arrhythmia are changes in heart rate, whether faster (tachycardia) or slower (bradycardia). Medicinal treatments for this condition work primarily to counteract tachycardia or atrial fibrillation by slowing down heart rate, as done by cardiac glycosides.[8]
Nevertheless, due to questions of toxicity and dosage, cardiac glycosides have been replaced with synthetic drugs such as ACE inhibitors and beta blockers and are no longer used as the primary medical treatment for such conditions. Depending on the severity of the condition, though, they may still be used in conjunction with other treatments.[8]
Toxicity
From ancient times, humans have used cardiac-glycoside-containing plants and their crude extracts as arrow coatings, homicidal or suicidal aids, rat poisons, heart tonics, diuretics and emetics, primarily due to the toxic nature of these compounds.[6] US poison centers reported 2632 cases regarding digotoxin toxicity, and 17 cases regarding digotoxin-related deaths in 2008.[12]
Because they interfere with fundamental processes that regulate membrane potential they are toxic to the heart, the brain, and the gut, at doses that are not difficult to reach. In the heart, the most common negative effect is Premature ventricular contraction.[6] [13]
References
- 1 2 3 4 Patel, Seema (2016-12-01). "Plant-derived cardiac glycosides: Role in heart ailments and cancer management". Biomedicine & Pharmacotherapy = Biomedecine & Pharmacotherapie. 84: 1036–1041. ISSN 1950-6007. PMID 27780131. doi:10.1016/j.biopha.2016.10.030.
- ↑ Ambrosy, Andrew P.; Butler, Javed; Ahmed, Ali; Vaduganathan, Muthiah; van Veldhuisen, Dirk J.; Colucci, Wilson S.; Gheorghiade, Mihai (2014-05-13). "The use of digoxin in patients with worsening chronic heart failure: reconsidering an old drug to reduce hospital admissions". Journal of the American College of Cardiology. 63 (18): 1823–1832. ISSN 1558-3597. PMID 24613328. doi:10.1016/j.jacc.2014.01.051.
- ↑ Riganti, C.; Campia, I.; Kopecka, J.; Gazzano, E.; Doublier, S.; Aldieri, E.; Bosia, A.; Ghigo, D. (2011-01-01). "Pleiotropic effects of cardioactive glycosides". Current Medicinal Chemistry. 18 (6): 872–885. ISSN 1875-533X. PMID 21182478.
- 1 2 "Cardiac Glycosides". www.people.vcu.edu. Retrieved 2017-05-25.
- 1 2 Cheeke, Peter R. (1989-07-31). Toxicants of Plant Origin: Glycosides. CRC Press. ISBN 9780849369919.
- 1 2 3 "Cardiac Glycoside Plant Poisoning: Practice Essentials, Pathophysiology, Etiology". 2017-05-05.
- 1 2 "Pharmacognosy 2|Digital Textbook Library". www.tankonyvtar.hu. Retrieved 2017-06-08.
- 1 2 3 Bullock, Shane; Manias, Elizabeth (2013-10-15). Fundamentals of Pharmacology. Pearson Higher Education AU. ISBN 9781442564411.
- ↑ Babula, Petr; Masarik, Michal; Adam, Vojtech; Provaznik, Ivo; Kizek, Rene (2013-09-01). "From Na+/K+-ATPase and cardiac glycosides to cytotoxicity and cancer treatment". Anti-Cancer Agents in Medicinal Chemistry. 13 (7): 1069–1087. PMID 23537048.
- ↑ "CV Pharmacology | Cardiac Glycosides (Digitalis Compounds)". cvpharmacology.com. Retrieved 2017-06-08.
- ↑ "How Is Heart Failure Treated? - NHLBI, NIH". www.nhlbi.nih.gov. Retrieved 2017-06-08.
- ↑ Bronstein, Alvin C.; Spyker, Daniel A.; Cantilena, Louis R.; Green, Jody L.; Rumack, Barry H.; Giffin, Sandra L. (2009-12-01). "2008 Annual Report of the American Association of Poison Control Centers' National Poison Data System (NPDS): 26th Annual Report". Clinical Toxicology (Philadelphia, Pa.). 47 (10): 911–1084. ISSN 1556-9519. PMID 20028214. doi:10.3109/15563650903438566.
- ↑ Kanji, Salmaan; MacLean, Robert D. (2012-10-01). "Cardiac glycoside toxicity: more than 200 years and counting". Critical Care Clinics. 28 (4): 527–535. PMID 22998989. doi:10.1016/j.ccc.2012.07.005.