Crassulacean acid metabolism
Crassulacean acid metabolism, also known as CAM photosynthesis, is a carbon fixation pathway that evolved in some plants as an adaptation to arid conditions.[1] In a plant using full CAM, the stomata in the leaves remain shut during the day to reduce evapotranspiration, but open at night to collect carbon dioxide (CO2). The CO2 is stored as the four-carbon acid malate in vacuoles at night, and then in the daytime, the malate is transported to chloroplasts where it is converted back to CO2, which is then used during photosynthesis. The pre-collected CO2 is concentrated around the enzyme RuBisCO, increasing photosynthetic efficiency. This metabolism was first studied in plants of the Crassulaceae family. These mainly include succulents. The first time it was studied, Crassula was used as a model organism. The word crassulacean is from the Latin word crassus which means “thick,” and succulent plants have leaves that are thick and full of moisture and they often have a waxy or hairy coating to help prevent evaporation. [2]
Historical background
CAM was first suspected by de Saussure in 1804 in his Recherches Chimiques sur la Vegetation, confirmed and refined by Aubert, E. in 1892 in his Recherches physiologiques sur les plantes grasses and expounded upon by Richards, H. M. 1915 in Acidity and Gas Interchange in Cacti, Carnegie Institution. The term CAM may have been coined by Ranson and Thomas in 1940, but they were not the first to discover this cycle. It was observed by the botanists Ranson and Thomas, in the Crassulaceae family of succulents (which includes jade plants and Sedum).[3] Its name refers to acid metabolism in Crassulaceae, not the metabolism of "crassulacean acid".
Overview: a two-part cycle

CAM is an adaptation for increased efficiency in the use of water, and so is typically found in plants growing in arid conditions.[4]
During the night
During the night, a plant employing CAM has its stomata open, allowing CO2 to enter and be fixed as organic acids that are stored in vacuoles. During the day the stomata are closed (thus preventing water loss), and the carbon is released to the Calvin cycle so that photosynthesis may take place.
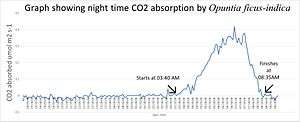
The carbon dioxide is fixed in the cytoplasm of mesophyll cells by a PEP reaction similar to that of C4 pathway. But, unlike the C4 mechanism, the resulting organic acids are stored in vacuoles for later use; that is, they are not immediately passed on to the Calvin cycle. The latter cannot operate during the night because the light reactions that provide it with ATP and NADPH cannot take place.
During the day
During the day, the CO2-storing organic acids are released from the vacuoles of the mesophyll cells and enter the stroma of the chloroplasts where an enzyme releases the CO2, which then enters into the Calvin cycle.
Benefits
The most important benefit of CAM to the plant is the ability to leave most leaf stomata closed during the day.[5] Plants employing CAM are most common in arid environments, where water comes at a premium. Being able to keep stomata closed during the hottest and driest part of the day reduces the loss of water through evapotranspiration, allowing such plants to grow in environments that would otherwise be far too dry. Plants using only C3 carbon fixation, for example, lose 97% of the water they uptake through the roots to transpiration - a high cost avoided by plants able to employ CAM.[6]
Comparison with C4 metabolism

The C4 pathway bears resemblance to CAM; both act to concentrate CO2 around RuBisCO, thereby increasing its efficiency. CAM concentrates it temporally, providing CO2 during the day, and not at night, when respiration is the dominant reaction. C4 plants, in contrast, concentrate CO2 spatially, with a RuBisCO reaction centre in a "bundle sheath cell" being inundated with CO2. Due to the inactivity required by the CAM mechanism, C4 carbon fixation has a greater efficiency in terms of PGA synthesis
Biochemistry

Plants with CAM must control storage of CO2 and its reduction to branched carbohydrates in space and time.
At low temperatures (frequently at night), plants using CAM open their stomata, CO2 molecules diffuse into the spongy mesophyll's intracellular spaces and then into the cytoplasm. Here, they can meet phosphoenolpyruvate (PEP), which is a phosphorylated triose. During this time, the plants are synthesizing a protein called PEP carboxylase kinase (PEP-C kinase), whose expression can be inhibited by high temperatures (frequently at daylight) and the presence of malate. PEP-C kinase phosphorylates its target enzyme PEP carboxylase (PEP-C). Phosphorylation dramatically enhances the enzyme's capability to catalyze the formation of oxaloacetate, which can be subsequently transformed into malate by NAD+ malate dehydrogenase. Malate is then transported via malate shuttles into the vacuole, where it is converted into the storage form malic acid. In contrast to PEP-C kinase, PEP-C is synthesized all the time but almost inhibited at daylight either by dephosphorylation via PEP-C phosphatase or directly by binding malate. The latter is not possible at low temperatures, since malate is efficiently transported into the vacuole, whereas PEP-C kinase readily inverts dephosphorylation.
In daylight, plants using CAM close their guard cells and discharge malate that is subsequently transported into chloroplasts. There, depending on plant species, it is cleaved into pyruvate and CO2 either by malic enzyme or by PEP carboxykinase. CO2 is then introduced into the Calvin cycle, a coupled and self-recovering enzyme system, which is used to build branched carbohydrates. The by-product pyruvate can be further degraded in the mitochondrial citric acid cycle, thereby providing additional CO2 molecules for the Calvin Cycle. Pyruvate can also be used to recover PEP via pyruvate phosphate dikinase, a high-energy step, which requires ATP and an additional phosphate. During the following cool night, PEP is finally exported into the cytoplasm, where it is involved in fixing carbon dioxide via malate.
Use by plants

Plants use CAM to different degrees. Some are "obligate CAM plants", i.e. they use only CAM in photosynthesis, although they vary in the amount of CO2 they are able to store as organic acids; they are sometimes divided into "strong CAM" and "weak CAM" plants on this basis. Other plants show "inducible CAM", in which they are able to switch between using either the C3 or C4 mechanism and CAM depending on environmental conditions. Another group of plants employ "CAM-cycling", in which their stomata do not open at night; the plants instead recycle CO2 produced by respiration as well as storing some CO2 during the day.[4]
Plants showing inducible CAM and CAM-cycling are typically found in conditions where periods of water shortage alternate with periods when water is freely available. Periodic drought – a feature of semi-arid regions – is one cause of water shortage. Plants which grow on trees or rocks (as epiphytes or lithophytes) also experience variations in water availability. Salinity, high light levels and nutrient availability are other factors which have been shown to induce CAM.[4]
Since CAM is an adaptation to arid conditions, plants using CAM often display other xerophytic characters, such as thick, reduced leaves with a low surface-area-to-volume ratio; thick cuticle; and stomata sunken into pits. Some shed their leaves during the dry season; others (the succulents) store water in vacuoles. CAM also causes taste differences: plants may have an increasingly sour taste during the night yet become sweeter-tasting during the day. This is due to malic acid being stored in the vacuoles of the plants' cells during the night and then being used up during the day.[7]
Aquatic CAM
CAM photosynthesis is also found in aquatic species in at least 4 genera, including: Isoetes, Crassula, Littorella, Sagittaria, and possibly Vallisneria,[8] being found in a variety of species e.g. Isoetes howellii, Crassula aquatica.
These plants follow the same nocturnal acid accumulation and daytime deacidification as terrestrial CAM species.[9] However, the reason for CAM in aquatic plants is not due to a lack of available water, but a limited supply of CO2.[8] CO2 is limited due to slow diffusion in water, 10000x slower than in air. The problem is especially acute under acid pH, where the only inorganic carbon species present is CO2, with no available bicarbonate or carbonate supply.
Aquatic CAM plants capture carbon at night when it is abundant due to a lack of competition from other photosynthetic organisms.[9] This also results in lowered photorespiration due to less photosynthetically generated oxygen.
Aquatic CAM is most marked in the summer months when there is increased competition for CO2, compared to the winter months. However, in the winter months CAM still has a significant role.[10]
Ecological and taxonomic distribution of CAM-using plants
The majority of plants possessing CAM are either epiphytes (e.g., orchids, bromeliads) or succulent xerophytes (e.g., cacti, cactoid Euphorbias), but CAM is also found in hemiepiphytes (e.g., Clusia); lithophytes (e.g., Sedum, Sempervivum); terrestrial bromeliads; wetland plants (e.g., Isoetes, Crassula (Tillaea), Lobelia;[11] and in one halophyte, Mesembryanthemum crystallinum; one non-succulent terrestrial plant, (Dodonaea viscosa) and one mangrove associate (Sesuvium portulacastrum).
Plants which are able to switch between different methods of carbon fixation include Portulacaria afra, better known as Dwarf Jade Plant, which normally uses C3 fixation but can use CAM if it is drought-stressed,[12] and Portulaca oleracea, better known as Purslane, which normally uses C4 fixation but is also able to switch to CAM when drought-stressed.[13]
CAM has evolved convergently many times.[14] It occurs in 16,000 species (about 7% of plants), belonging to over 300 genera and around 40 families, but this is thought to be a considerable underestimate.[15] It is found in quillworts (relatives of club mosses), in ferns, and in Gnetopsida, but the great majority of plants using CAM are angiosperms (flowering plants).
The following list summarizes the taxonomic distribution of plants with CAM:
Division | Class/Angiosperm group | Order | Family | Plant Type | Clade involved |
---|---|---|---|---|---|
Lycopodiophyta | Isoetopsida | Isoetales | Isoetaceae | hydrophyte | Isoetes[16] (the sole genus of class Isoetopsida) - I. howellii (seasonally submerged), I. macrospora, I. bolanderi, I. engelmanni, I. lacustris, I. sinensis, I. storkii, I. kirkii |
Pteridophyta | Polypodiopsida | Polypodiales | Polypodiaceae | epiphyte, lithophyte | CAM is recorded from Microsorium, Platycerium and Polypodium,[17] Pyrrosia and Drymoglossum[18] and Microgramma |
Pteridopsida | Polypodiales | Pteridaceae[19] | epiphyte | Vittaria[20]
Anetium citrifolium[21] | |
Cycadophyta | Cycadopsida | Cycadales | Zamiaceae | Dioon edule[22] | |
Pinophyta | Gnetopsida | Welwitschiales | Welwitschiaceae | xerophyte | Welwitschia mirabilis[23] (the sole species of the order Welwitschiales) |
Magnoliophyta | magnoliids | Magnoliales | Piperaceae | epiphyte | Peperomia camptotricha[24] |
eudicots | Caryophyllales | Aizoaceae | xerophyte | widespread in the family; Mesembryanthemum crystallinum is a rare instance of an halophyte that displays CAM[25] | |
Cactaceae | xerophyte | Almost all cacti have obligate Crassulacean Acid Metabolism in their stems; the few cacti with leaves may have C3 Metabolism in those leaves;[26] seedlings have C3 Metabolism.[27] | |||
Portulacaceae | xerophyte | recorded in approximately half of the genera (note: Portulacaceae is paraphyletic with respect to Cactaceae and Didieraceae)[28] | |||
Didiereaceae | xerophyte | ||||
Saxifragales | Crassulaceae | hydrophyte, xerophyte, lithophyte | CAM is widespread in the family | ||
eudicots (rosids) | Vitales | Vitaceae[29] | Cissus,[30] Cyphostemma | ||
Malpighiales | Clusiaceae | hemiepiphyte | Clusia[30][31] | ||
Euphorbiaceae[29] | CAM is found is some species of Euphorbia[30][32] including some formerly placed in the sunk genera Monadenium,[30] Pedilanthus[32] and Synadenium. C4 photosynthesis is also found in Euphorbia (subgenus Chamaesyce). | ||||
Passifloraceae[19] | xerophyte | Adenia[33] | |||
Geraniales | Geraniaceae | CAM is found in some succulent species of Pelargonium,[34] and is also reported from Geranium pratense | |||
Cucurbitales | Cucurbitaceae | Xerosicyos danguyi,[35] Dendrosicyos socotrana, Momordica | |||
Celastrales | Celastraceae | ||||
Oxalidales | Oxalidaceae[36] | Oxalis carnosa var. hirta[36] | |||
Brassicales | Moringaceae | Moringa | |||
Salvadoraceae[36] | CAM is found in Salvadora persica.[36] Salvadoraceae were previously placed in order Celastrales, but are now placed in Brassicales. | ||||
Sapindales | Sapindaceae | Dodonaea viscosa | |||
Fabales | Fabaceae[36] | CAM is found in Prosopis juliflora (listed under the family Salvadoraceae in Sayed's (2001) table,[36]) but is currently in the family Fabaceae (Leguminosae) according to The Plant List[37]). | |||
Zygophyllaceae | Zygophyllum | ||||
eudicots (asterids) | Ericales | Ebenaceae | |||
Solanales | Convolvulaceae | Ipomoea (Some species of Ipomoea are C3[30][38] - a citation is needed here.) | |||
Gentianales | Rubiaceae | epiphyte | Hydnophytum and Myrmecodia | ||
Apocynaceae | CAM is found in subfamily Asclepidioideae (Hoya,[30] Dischidia, Ceropegia, Stapelia,[32] Caralluma negevensis, Frerea indica,[39] Adenium, Huernia), and also in Carissa and Akocanthera | ||||
Lamiales | Gesneriaceae | epiphyte | CAM was found Codonanthe crassifolia, but not in 3 other genera[40] | ||
Lamiaceae | Plectranthus marrubioides, Coleus | ||||
Plantaginaceae | hydrophyte | Littorella uniflora[16] | |||
Apiales | Apiaceae | hydrophyte | Lilaeopsis lacustris | ||
Asterales | Asteraceae[29] | some species of Senecio[41] | |||
Magnoliophyta | monocots | Alismatales | Hydrocharitaceae | hydrophyte | Hydrilla,[29] Vallisneria |
Alismataceae | hydrophyte | Sagittaria | |||
Araceae | Zamioculcas zamiifolia is the only CAM plant in Araceae, and the only non-aquatic CAM plant in Alismatales[42] | ||||
Poales | Bromeliaceae | epiphyte | Bromelioideae (91%), Puya (24%), Dyckia and related genera (all), Hechtia (all), Tillandsia (many)[43] | ||
Cyperaceae | hydrophyte | Scirpus,[29] Eleocharis | |||
Asparagales | Orchidaceae | epiphyte | The Orchidaceae has more CAM species than any other family (CAM Orchids) | ||
Agavaceae[31] | xerophyte | Agave,[30] Hesperaloe, Yucca and Polianthes[33] | |||
Asphodelaceae[29] | xerophyte | Aloe,[30] Gasteria,[30] and Haworthia | |||
Ruscaceae[29] | Sansevieria[30][36] (This genus is listed under the family Dracaenaceae in Sayed's (2001) table, but currently in the family Asparagaceae according to The Plant List), Dracaena | ||||
Commelinales | Commelinaceae | Callisia,[30] Tradescantia, Tripogandra |
See also
References
- ↑ C.Michael Hogan. 2011. Respiration. Encyclopedia of Earth. Eds. Mark McGinley & C.J.cleveland. National council for Science and the Environment. Washington DC
- ↑ "CAM Plants". biologydictionary.net. Retrieved 11 July 2017.
- ↑ Ranson S. L.; Thomas M (1960). "Crassulacean acid metabolism". Annual Review of Plant Physiology. 11 (1): 81–110. doi:10.1146/annurev.pp.11.060160.000501.
- 1 2 3 Herrera, A. (2008), "Crassulacean acid metabolism and fitness under water deficit stress: if not for carbon gain, what is facultative CAM good for?", Annals of Botany, 103 (4): 645–653, PMC 2707347
, PMID 18708641, doi:10.1093/aob/mcn145
- ↑ Ting, I P (1985). "Crassulacean Acid Metabolism". Annual Review of Plant Physiology. 36 (1): 595–622. doi:10.1146/annurev.pp.36.060185.003115.
- ↑ Raven JA, Edwards D (2001). "Roots: evolutionary origins and biogeochemical significance". Journal of Experimental Botany. 52 (90001): 381–401. PMID 11326045. doi:10.1093/jexbot/52.suppl_1.381.
- ↑ Raven, P & Evert, R & Eichhorn, S, 2005, "Biology of Plants" (seventh edition), p. 135 (Figure 7-26), W.H. Freeman and Company Publishers ISBN 0-7167-1007-2
- 1 2 Keeley, J (1998). "CAM Photosynthesis in Submerged Aquatic Plants". Botanical Review. 64 (2): 121–175. doi:10.1007/bf02856581.
- 1 2 Keeley, J; Busch, G (1984). "Carbon Assimilation Characteristics of the Aquatic CAM Plant, Isoetes howellii". Plant Physiology. 75: 525–530. doi:10.1104/pp.76.2.525.
- ↑ Klavsen, S; Madsen, T (2012). "Seasonal variation in crassulacean acid metabolism by the aquatic isoetid Littorella uniflora". Photosynthesis Research. 112: 163–173. doi:10.1007/s11120-012-9759-0.
- ↑ Keddy, P.A. (2010). Wetland Ecology: Principles and Conservation. Cambridge University Press, Cambridge, UK. p. 26.
- ↑ Guralnick, L. J.; Ting, I. P. (1987). "Physiological Changes in Portulacaria afra (L.) Jacq. during a Summer Drought and Rewatering". Plant Physiology. 85 (2): 481–6. PMC 1054282
. PMID 16665724. doi:10.1104/pp.85.2.481.
- ↑ Koch, K. E.; Kennedy, R. A. (1982). "Crassulacean Acid Metabolism in the Succulent C4 Dicot, Portulaca oleracea L Under Natural Environmental Conditions". Plant Physiology. 69 (4): 757–61. PMC 426300
. PMID 16662291. doi:10.1104/pp.69.4.757.
- ↑ Keeley, Jon E.; Rundel, Philip W. (2003). "Evolution of CAM and C4 Carbon‐Concentrating Mechanisms" (PDF). International Journal of Plant Sciences. 164 (S3): S55. doi:10.1086/374192.
- ↑ Dodd, A. N.; Borland, A. M.; Haslam, R. P.; Griffiths, H.; Maxwell, K. (2002). "Crassulacean acid metabolism: plastic, fantastic". Journal of Experimental Botany. 53 (369): 569–580. PMID 11886877. doi:10.1093/jexbot/53.369.569.
- 1 2 Boston, H (1983). "Evidence of crussulacean acid metabolism in two North American isoetids". Aquatic Botany. 15 (4): 381–386. doi:10.1016/0304-3770(83)90006-2.
- ↑ Holtum, Joseph A.M.; Winter, Klaus (1999). "Degrees of crassulacean acid metabolism in tropical epiphytic and lithophytic ferns". Australian Journal of Plant Physiology. 26 (8): 749. doi:10.1071/PP99001.
- ↑ Wong, S.C.; Hew, C.S (1976). "Diffusive Resistance, Titratable Acidity, and CO2 Fixation in Two Tropical Epiphytic Ferns". American Fern Journal. American Fern Society. 66 (4): 121–124. JSTOR 1546463. doi:10.2307/1546463.
- 1 2 Crassulacean Acid Metabolism
- ↑ abstract to Carter & Martin, The occurrence of Crassulacean acid metabolism among ephiphytes in a high-rainfall region of Costa Rica, Selbyana 15(2): 104-106 (1994)
- ↑ Martin, Shannon L.; Davis, Ryan; Protti, Piero; Lin, Teng‐Chiu; Lin, Shin‐Hwei; Martin, Craig E. (2005). "The Occurrence of Crassulacean Acid Metabolism in Epiphytic Ferns, with an Emphasis on the Vittariaceae". International Journal of Plant Sciences. 166 (4): 623–630. doi:10.1086/430334.
- ↑ Vovides, Andrew P.; Etherington, John R.; Dresser, P. Quentin; Groenhof, Andrew; Iglesias, Carlos; Ramirez, Jonathan Flores (2002). "CAM-cycling in the cycad Dioon edule Lindl. in its natural tropical deciduous forest habitat in central Veracruz, Mexico". Botanical Journal of the Linnean Society. 138 (2): 155–162. doi:10.1046/j.1095-8339.2002.138002155.x.
- ↑ Schulze, E. D.; Ziegler, H.; Stichler, W. (1976). "Environmental control of crassulacean acid metabolism in Welwitschia mirabilis Hook. Fil. in its range of natural distribution in the Namib desert". Oecologia. 24 (4): 323–334. doi:10.1007/BF00381138.
- ↑ Sipes, DL; Ting, IP (1985). "Crassulacean Acid Metabolism and Crassulacean Acid Metabolism Modifications in Peperomia camptotricha.". Plant Physiology. 77 (1): 59–63. PMC 1064456
. PMID 16664028. doi:10.1104/pp.77.1.59.
- ↑ Chu, C; Dai, Z; Ku, MS; Edwards, GE (1990). "Induction of Crassulacean Acid Metabolism in the Facultative Halophyte Mesembryanthemum crystallinum by Abscisic Acid.". Plant Physiology. 93 (3): 1253–1260. PMC 1062660
. PMID 16667587. doi:10.1104/pp.93.3.1253.
- ↑ Nobel, Park S.; Hartsock, Terry L. (1986). "Leaf and Stem CO2 Uptake in the Three Subfamilies of the Cactaceae". Plant Physiology. 80 (4): 913–917. doi:10.1104/pp.80.4.913.
- ↑ Winter, K.; Garcia, M.; Holtum, J. A. M. (2011). "Drought-stress-induced up-regulation of CAM in seedlings of a tropical cactus, Opuntia elatior, operating predominantly in the C3 mode". Journal of Experimental Botany. 62 (11): 4037–4042. ISSN 0022-0957. doi:10.1093/jxb/err106.
- ↑ Guralnick, Lonnie J.; Jackson, Michael D. (2001). "The Occurrence and Phylogenetics of Crassulacean Acid Metabolism in the Portulacaceae". International Journal of Plant Sciences. 162 (2): 257–262. doi:10.1086/319569.
- 1 2 3 4 5 6 7 Cockburn, W. (1985). "TANSLEY REVIEW No 1.. VARIATION IN PHOTOSYNTHETIC ACID METABOLISM IN VASCULAR PLANTS: CAM AND RELATED PHENOMENA". New Phytologist. 101 (1): 3–24. doi:10.1111/j.1469-8137.1985.tb02815.x.
- 1 2 3 4 5 6 7 8 9 10 11 Nelson, Elizabeth A.; Sage, Tammy L.; Sage, Rowan F. (2005). "Functional leaf anatomy of plants with crassulacean acid metabolism". Functional Plant Biology. 32 (5): 409. doi:10.1071/FP04195.
- 1 2 Lüttge, U (2004). "Ecophysiology of Crassulacean Acid Metabolism (CAM).". Annals of Botany. 93 (6): 629–52. PMID 15150072. doi:10.1093/aob/mch087.
- 1 2 3 Bender, MM; Rouhani, I.; Vines, H. M.; Black, C. C. (1973). "C/C Ratio Changes in Crassulacean Acid Metabolism Plants.". Plant Physiology. 52 (5): 427–430. PMC 366516
. PMID 16658576. doi:10.1104/pp.52.5.427.
- 1 2 Szarek, S.R. (1979). "The occurrence of Crassulacean Acid Metabolism a supplementary list during 1976 to 1979". Photosynthetica. 13 (4): 467–473.
- ↑ Jones, Cardon & Czaja (2003). "A phylogenetic view of low-level CAM in Pelargonium (Geraniaceae)". American Journal of Botany. 90 (1): 135–142. PMID 21659089. doi:10.3732/ajb.90.1.135.
- ↑ Bastide, Sipes, Hann & Ting (1993). "Effect of Severe Water Stress on Aspects of Crassulacean Acid Metabolism in Xerosicyos.". Plant Physiol. 103 (4): 1089–1096. PMC 159093
. PMID 12232003. doi:10.1104/pp.103.4.1089.
- 1 2 3 4 5 6 7 Sayed, O.H. (2001). "Crassulacean Acid Metabolism 1975–2000, a Check List". Photosynthetica. 39 (3): 339–352. doi:10.1023/A:1020292623960.
- ↑ "Prosopis juliflora". The Plant List. Retrieved 2015-09-11.
- ↑ Craig E. Martin; Anne E. Lubbers; James A. Teeri (1982). "Variability in Crassulacean Acid Metabolism A Survey of North Carolina Succulent Species". Botanical Gazette. The University of Chicago Press. 143: 491–497. JSTOR 2474765. doi:10.1086/337326.
- ↑ Lange, Otto L.; Zuber, Margit (1977). "Frerea indica, a stem succulent CAM plant with deciduous C3 leaves". Oecologia. 31 (1): 67–72. doi:10.1007/BF00348709.
- ↑ Guralnick; Ting, Irwin P; Lord, Elizabeth M; et al. (1986). "Crassulacean Acid Metabolism in the Gesneriaceae". American Journal of Botany. Botanical Society of America. 73 (3): 336–345. JSTOR 2444076. doi:10.2307/2444076.
- ↑ Fioretti & Alfani; Alfani, A (1988). "Anatomy of Succulence and CAM in 15 Species of Senecio". Botanical Gazette. The University of Chicago Press. 149 (2): 142–152. JSTOR 2995362. doi:10.1086/337701.
- ↑ Holtum, Winter, Weeks and Sexton (2007). "Crassulacean acid metabolism in the ZZ plant, Zamioculcas zamiifolia (Araceae)". American Journal of Botany. 94 (10): 1670–1676. PMID 21636363. doi:10.3732/ajb.94.10.1670.
- ↑ Crayn, D. M.; Winter, K; Smith, JA (2004). "Multiple origins of crassulacean acid metabolism and the epiphytic habit in the Neotropical family Bromeliaceae". Proceedings of the National Academy of Sciences. 101 (10): 3703–8. PMC 373526
. PMID 14982989. doi:10.1073/pnas.0400366101.
External links
![]() |
Wikimedia Commons has media related to CAM cycle. |