Alu element
An Alu element is a short stretch of DNA originally characterized by the action of the Arthrobacter luteus (Alu) restriction endonuclease.[1] Alu elements are the most abundant transposable elements, containing over one million copies dispersed throughout the human genome.[2] They are derived from the small cytoplasmic 7SL RNA, a component of the signal recognition particle. Alu elements are highly conserved within primate genomes and originated in the genome of an ancestor of Supraprimates.[3]
Alu insertions have been implicated in several inherited human diseases and in various forms of cancer.
The study of Alu elements has also been important in elucidating human population genetics and the evolution of primates, including the evolution of humans.
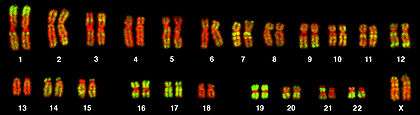
The Alu family
The Alu family is a family of repetitive elements in the human genome. Modern Alu elements are about 300 base pairs long and are therefore classified as short interspersed nuclear elements (SINEs) among the class of repetitive DNA elements. The typical structure is 5'Part A- A5TACA6 -Part B - PolyA Tail - 3', where Part A and Part B are similar nucleotide sequences. Expressed another way, it is believed modern Alu elements emerged from a head to tail fusion of two distinct FAMs (fossil antique monomers) over 100 mya, hence its dimeric structure of two similar, but distinct monomers (left and right arms) joined by an A-rich linker.[4] The length of the polyA tail varies between Alu families.
There are over one million Alu elements interspersed throughout the human genome, and it is estimated that about 10.7% of the human genome consists of Alu sequences. However, less than 0.5% are polymorphic (i.e. they occur in more than one form or morph).[5] In 1988, Jerzy Jurka and Temple Smith discovered that Alu elements were split in two major subfamilies known as AluJ and AluS, and other Alu subfamilies were also independently discovered by several groups.[6] Later on, a sub-subfamily of AluS which included active Alu elements was given the separate name AluY. Dating back 65 million years, the AluJ lineage is the oldest and least active in the human genome. The younger AluS lineage is about 30 million years old and still contains some active elements. Finally, the AluY elements are the youngest of the three and have the greatest disposition to move along the human genome.[7] The discovery of Alu subfamilies led to the hypothesis of master/source genes, and provided the definitive link between transposable elements (active elements) and interspersed repetitive DNA (mutated copies of active elements).[8]
7SL RNA
The sequence of the DNA for the 299 nucleotide long 7SL RNA:[9]
gccgggcgcg gtggcgcgtg cctgtagtcc cagctactcg ggaggctgAG GCTGgaGGAT CGcttgAGTC CAggagttct
gggctgtagt gcgctatgcc gatcgggtgt ccgcactaag ttcggcatca atatggtgac ctcccgggag cgggggacca
ccaggttgcc taaggagggg tgaaccggcc caggtcggaa acggagcagg tcaaaactcc cgtgctgatc agtagtggga
tcgcgcctgt gaatagccac tgcactccag cctgggcaac atagcgagac cccgtctct
The functional retinoic acid response element hexamer sites [10] are in upper case and overlap the internal transcriptional promoter. The recognition sequence of the Alu endonuclease is 5' AG/CT 3'; that is, the enzyme cuts the DNA segment between the guanine and cytosine residues (in bold above).
Alu elements
Alu elements are responsible for regulation of tissue-specific genes. They are also involved in the transcription of nearby genes and can sometimes change the way a gene is expressed.[11]
Alu elements are retrotransposons and look like DNA copies made from RNA polymerase III-encoded RNAs. Alu elements do not encode for protein products. They are replicated as any other DNA sequence, but depend on LINE retrotransposons for generation of new elements.[12]
Alu elements replication and mobilization begins by interactions with signal recognition particles (SRPs), which aid newly translated proteins reach final destinations.[13] Alu RNA forms a specific RNA:protein complex with a protein heterodimer consisting of SRP9 and SRP14.[14] SRP9/14 facilitates Alu's attachment to ribosomes that capture nascent L1 proteins. Thus, an Alu element can take control of the L1 protein's reverse transcriptase, ensuring that the Alu's RNA sequence gets copied into the genome rather than the L1's mRNA.[15]
Alu elements in primates form a fossil record that is relatively easy to decipher because Alu elements insertion events have a characteristic signature that is both easy to read and faithfully recorded in the genome from generation to generation. The study of Alu elements thus reveals details of ancestry because individuals will only share a particular Alu element insertion if they have a common ancestor.
Most human Alu element insertions can be found in the corresponding positions in the genomes of other primates, but about 7,000 Alu insertions are unique to humans.[16]
Impact of Alu in humans
Alu elements are a common source of mutation in humans, but such mutations are often confined to non-coding regions where they have little discernible impact on the bearer.[17] (That is, a given mutation may not cause any difference to exist [or, might cause only a small difference to exist] in the phenotype of the individual whose DNA contains that mutation—relative to what that phenotype would have been without that mutation, but with "other things being equal" [that is, with all other parts of the genotype being the same].) However, the variation generated can be used in studies of the movement and ancestry of human populations,[18] and the mutagenic effect of Alu[19] and retrotransposons in general[20] has played a major role in the recent evolution of the human genome. There are also a number of cases where Alu insertions or deletions are associated with specific effects in humans:
Associations with human disease
Alu insertions are sometimes disruptive and can result in inherited disorders. However, most Alu variation acts as markers that segregate with the disease so the presence of a particular Alu allele does not mean that the carrier will definitely get the disease. The first report of Alu-mediated recombination causing a prevalent inherited predisposition to cancer was a 1995 report about hereditary nonpolyposis colorectal cancer.[21]
The following human diseases have been linked with Alu insertions:[22][23]
- Alport syndrome
- Breast cancer
- chorioretinal degeneration
- Ewing's sarcoma
- Familial hypercholesterolemia
- Hemophilia
- Leigh syndrome
- mucopolysaccharidosis VII
- Neurofibromatosis
- Diabetes mellitus type II
And the following diseases have been associated with single-nucleotide DNA variations in Alu elements impacting transcription levels:[24]
Other Alu-associated human mutations
- The ACE gene, encoding angiotensin-converting enzyme, has 2 common variants, one with an Alu insertion (ACE-I) and one with the Alu deleted (ACE-D). This variation has been linked to changes in sporting ability: the presence of the Alu element is associated with better performance in endurance-oriented events (e.g. triathlons), whereas its absence is associated with strength- and power-oriented performance[25]
- The opsin gene duplication which resulted in the re-gaining of trichromacy in Old World primates (including humans) is flanked by an Alu element,[26] implicating the role of Alu in the evolution of three colour vision.
References
- ↑ Schmid CW, Deininger PL (1975). "Sequence organization of the human genome". Cell. 6: 345–358. PMID 1052772. doi:10.1016/0092-8674(75)90184-1.
- ↑ Szmulewicz, M. N.; Novick, G. E.; Herrera, R. J. (1 June 1998). "Effects of Alu insertions on gene function". Electrophoresis. 19 (8–9): 1260–1264. ISSN 0173-0835. PMID 9694261. doi:10.1002/elps.1150190806.
- ↑ Kriegs JO, Churakov G, Jurka J, Brosius J, Schmitz J (April 2007). "Evolutionary history of 7SL RNA-derived SINEs in Supraprimates" (PDF). Trends Genet. 23 (4): 158–61. PMID 17307271. doi:10.1016/j.tig.2007.02.002.
- ↑ Hasler J, Strub K (2006). "Alu elements as regulators of gene expression". Nucleic Acids Research. 34 (19): 5491–5497. PMC 1636486
. PMID 17020921. doi:10.1093/nar/gkl706.
- ↑ Roy-Engel AM, Carroll ML, Vogel E, et al. (September 2001). "Alu insertion polymorphisms for the study of human genomic diversity". Genetics. 159 (1): 279–90. PMC 1461783
. PMID 11560904.
- ↑ Jurka J, Smith T (July 1988). "A fundamental division in the Alu family of repeated sequences". Proceedings of the National Academy of Sciences. 85 (July): 279–90. doi:10.1073/pnas.85.13.4775.
- ↑ Bennett, E. Andrew; Keller, Heiko; Mills, Ryan E.; Schmidt, Steffen; Moran, John V.; Weichenrieder, Oliver; Devine, Scott E. (1 December 2008). "Active Alu retrotransposons in the human genome". Genome Research. 18 (12): 1875–1883. ISSN 1088-9051. PMC 2593586
. PMID 18836035. doi:10.1101/gr.081737.108.
- ↑ Shen MR, Batzer MA, Deininger PL (October 1991). "Evolution of the master Alu gene(s)". J. Mol. Evol. 33 (4): 311–320. doi:10.1007/bf02102862.
- ↑ "NCBI Genbank DNA encoding 7SL RNA".
- ↑ Vansant G, Reynolds WF (August 1995). "The consensus sequence of a major Alu subfamily contains a functional retinoic acid response element". Proc. Natl. Acad. Sci. USA. 92 (18): 8229–8233. PMC 41130
. PMID 7667273. doi:10.1073/pnas.92.18.8229.
- ↑ Britten, R J (1996-09-03). "DNA sequence insertion and evolutionary variation in gene regulation.". Proceedings of the National Academy of Sciences of the United States of America. 93 (18): 9374–9377. ISSN 0027-8424. PMC 38434
. PMID 8790336.
- ↑ Kramerov DA, Vassetzky NS (2005). "Short retroposons in eukaryotic genomes" (PDF). Int. Rev. Cytol. 247: 165–221. PMID 16344113. doi:10.1016/S0074-7696(05)47004-7.
- ↑ Weichenrieder, Oliver; Wild, Klemens; Strub, Katharina; Cusack, Stephen (9 November 2000). "Structure and assembly of the Alu domain of the mammalian signal recognition particle". Nature. 408 (6809): 167–173. ISSN 0028-0836. PMID 11089964. doi:10.1038/35041507.
- ↑ Weichenrieder, O.; Wild, K.; Strub, K.; Cusack, S. (2000-11-09). "Structure and assembly of the Alu domain of the mammalian signal recognition particle". Nature. 408 (6809): 167–173. ISSN 0028-0836. PMID 11089964. doi:10.1038/35041507.
- ↑ Bennett, E. Andrew; Keller, Heiko; Mills, Ryan E.; Schmidt, Steffen; Moran, John V.; Weichenrieder, Oliver; Devine, Scott E. (1 December 2008). "Active Alu retrotransposons in the human genome". Genome Research. 18 (12): 1875–1883. ISSN 1088-9051. PMC 2593586
. PMID 18836035. doi:10.1101/gr.081737.108.
- ↑ The Chimpanzee Sequencing and Analysis Consortium (September 2005). "Initial sequence of the chimpanzee genome and comparison with the human genome". Nature. 437 (7055): 69–87. PMID 16136131. doi:10.1038/nature04072.
- ↑ International Human Genome Sequencing Consortium (2001). "Initial sequencing and analysis of the human genome". Nature. 409 (6822): 860–921. PMID 11237011. doi:10.1038/35057062.
- ↑ Batzer, Mark A.; Deininger, Prescott L. (1 May 2002). "Alu repeats and human genomic diversity". Nature Reviews Genetics. 3 (5): 370–379. ISSN 1471-0056. PMID 11988762. doi:10.1038/nrg798.
- ↑ Shen S, Lin L, Cai JJ, Jiang P, Kenkel EJ, Stroik MR, Sato S, Davidson BL, Xing Y (2011). "Widespread establishment and regulatory impact of Alu exons in human genes". PNAS. 108 (7): 2837–42. PMC 3041063
. PMID 21282640. doi:10.1073/pnas.1012834108.
- ↑ Cordaux R, Batzer MA (2009). "The impact of retrotransposons on human genome evolution" (PDF). Nature Reviews Genetics. 10: 691–703. PMC 2884099
. PMID 19763152. doi:10.1038/nrg2640.
- ↑ Nyström-Lahti M, Kristo P, Nicolaides NC, et al. (November 1995). "Founding mutations and Alu-mediated recombination in hereditary colon cancer". Nat. Med. 1 (11): 1203–6. PMID 7584997. doi:10.1038/nm1195-1203.
- ↑ Batzer MA, Deininger PL (May 2002). "Alu repeats and human genomic diversity" (PDF). Nat. Rev. Genet. 3 (5): 370–9. PMID 11988762. doi:10.1038/nrg798.
- ↑ Deininger P. Alu elements: know the SINEs. Genome Biology. 2011;12(12):236. doi:10.1186/gb-2011-12-12-236.
- ↑ "SNPedia: SNP in the promoter region of the myeloperoxidase MPO gene".
- ↑ Puthucheary Z, Skipworth J, Rawal J, Loosemore M, Van Someren K, Montgomery H (2011). "The ACE Gene and Human Performance: 12 Years On". Sports Medicine. 41: 433–448. PMID 21615186. doi:10.2165/11588720-000000000-00000.
- ↑ Dulai KS, Von Dornum M, Mollon JD, Hunt DM (1999). "The Evolution of Trichromatic Color Vision by Opsin Gene Duplication in New World and Old World Primates". Genome Research. 9 (7): 629–638. PMID 10413401. doi:10.1101/gr.9.7.629.
External links
- Alu Repetitive Sequences at the US National Library of Medicine Medical Subject Headings (MeSH)