Vulcanization
Vulcanization or vulcanisation is a chemical process for converting natural rubber or related polymers into more durable materials via the addition of sulfur[1] or other equivalent curatives or accelerators. These additives modify the polymer by forming cross-links (bridges) between individual polymer chains.[2][3] Vulcanized materials are less sticky and have superior mechanical properties. The term vulcanized fibre refers to cellulose that has been treated in a zinc chloride solution to cross-link the cellulose fibers.
Although the curing of rubber has been carried out since prehistoric times, the modern process of vulcanization, named after Vulcan, the Roman god of fire, was not developed until the 19th century, mainly by Charles Goodyear. Today, a vast array of products are made with vulcanized rubber including tires, shoe soles, hoses, and conveyor belts. Hard vulcanized rubber is sometimes sold under the brand names ebonite or vulcanite, and is used in making articles such as clarinet and saxophone mouth pieces, bowling balls and hockey pucks.
History
Although vulcanization is a 19th-century invention, the history of rubber cured by other means goes back to prehistoric times. The name "Olmec" means "rubber people" in the Aztec language. Ancient Mesoamericans, spanning from ancient Olmecs to Aztecs, extracted latex from Castilla elastica, a type of rubber tree in the area. The juice of a local vine, Ipomoea alba, was then mixed with this latex to create processed rubber as early as 1600 BC.[4] In the western world, rubber remained a curiosity, although it was used to produce waterproofed products, such as Mackintosh rainwear.[5]
Modern developments
Thomas Hancock (1786–1865), a scientist and engineer, was the first to patent vulcanization of rubber. He was awarded a British patent on May 21, 1844. Three weeks later, on June 15, 1844, Charles Goodyear was awarded a patent in the United States.[6]
Goodyear claimed that he had discovered vulcanization earlier, in 1839. He wrote the story of the discovery in 1853 in his autobiographical book Gum-Elastica. Here is Goodyear's account of the invention, taken from Gum-Elastica. Although the book is an autobiography, Goodyear chose to write it in the third person so that "the inventor" and "he" referred to in the text are the author. He describes the scene in a rubber factory where his brother worked:
... The inventor made are experiments to ascertain the effect of heat on the same compound that had decomposed in the mail-bags and other articles. He was surprised to find that the specimen, being carelessly brought into contact with a hot stove, charred like leather.
Goodyear goes on to describe how his discovery was not readily accepted.
He directly inferred that if the process of charring could be stopped at the right point, it might divest the gum of its native adhesiveness throughout, which would make it better than the native gum. Upon further trial with heat, he was further convinced of the correctness of this inference, by finding that the India rubber could not be melted in boiling sulfur at any heat, but always charred. He made another trial of heating a similar fabric before an open fire. The same effect, that of charring the gum, followed. There were further indications of success in producing the desired result, as upon the edge of the charred portion appeared a line or border, that was not charred, but perfectly cured.
Goodyear then goes on to describe how he moved to Woburn, Massachusetts and carried out a series of systematic experiments to optimize the curing of rubber, collaborating with Nathaniel Hayward.
... On ascertaining to a certainty that he had found the object of his search and much more, and that the new substance was proof against cold and the solvent of the native gum, he felt himself amply repaid for the past, and quite indifferent to the trials of the future.
Later developments
The discovery of the rubber-sulfur reaction revolutionized the use and applications of rubber, and changed the face of the industrial world. Formerly, the only way to seal a small gap between moving machine parts was to use leather soaked in oil. This practice was acceptable only at moderate pressures, but above a certain point, machine designers were forced to compromise between the extra friction generated by tighter packing and greater leakage of steam. Vulcanized rubber solved this problem. It could be formed to precise shapes and dimensions, it accepted moderate to large deformations under load and recovered quickly to its original dimensions once the load is removed. These exceptional qualities, combined with good durability and lack of stickiness, were critical for an effective sealing material. Further experiments in the processing and compounding of rubber by Hancock and his colleagues led to a more reliable process.
In 1905 George Oenslager discovered that a derivative of aniline called thiocarbanilide accelerated the reaction of sulfur with rubber, leading to shorter cure times and reducing energy consumption. This breakthrough was almost as fundamental to the development of the rubber industry as Goodyear's sulfur cure. Accelerators made the cure process faster, improved the reliability of the process and enabled vulcanization to be applied to synthetic polymers. One year after his discovery, Oenslager had found hundreds of applications for his additive. Thus, the science of accelerators and retarders was born. An accelerator speeds up the cure reaction, while a retarder delays it. A typical retarder is cyclohexylthiophthalimide. In the subsequent century chemists developed other accelerators and ultra-accelerators, that are used in the manufacture of most modern rubber goods.
Uncured vs. vulcanized rubber
Uncured natural rubber is sticky, deforms easily when warm, and is brittle when cold. In this state, it is a poor material when a high level of elasticity is required. The reason for inelastic deformation of un-vulcanized rubber can be found in its chemical structure — rubber is composed of long polymer chains. These chains can move independently relative to each other, which lets the material change shape. Crosslinking introduced by vulcanization prevents the polymer chains from moving independently. As a result, when stress is applied the vulcanized rubber deforms, but upon release of the stress it reverts to its original shape.

Process
In contrast with thermoplastic processes (the melt-freeze process that characterize the behavior of most modern polymers) vulcanization, in common with the curing of other thermosetting polymers, is generally irreversible. The cross-linking is usually achieved by the addition of sulfur, but other technologies are known, including peroxide-based systems.
The main polymers subjected to vulcanization are polyisoprene (natural rubber) and styrene-butadiene rubber (SBR), which are used for most passenger car tires. The "cure package" is adjusted specifically for the substrate and the application. The reactive sites—"cure sites"—are allylic hydrogen atoms. These C-H bonds are adjacent to carbon-carbon double bonds. During vulcanization, some of these C-H bonds are replaced by chains of sulfur atoms that link with a cure site of another polymer chain. These bridges contain between one and eight atoms. The number of sulfur atoms in the crosslink strongly influences the physical properties of the final rubber article. Short crosslinks give the rubber better heat resistance. Crosslinks with higher number of sulfur atoms give the rubber good dynamic properties but less heat resistance. Dynamic properties are important for flexing movements of the rubber article, e.g., the movement of a side-wall of a running tire. Without good flexing properties these movements rapidly form cracks and, ultimately, make the rubber article fail.

Methods
A variety of methods exist for vulcanization. The economically most important method (vulcanization of tires) uses high pressure and temperature after the curative has been added to the rubber. A typical vulcanization temperature for a passenger tire is 10 minutes at 170 °C. This process employs the technique known as compression molding, where the rubber article is intended to adopt the shape of the mold. Other methods, for instance to make door profiles for cars, use hot air vulcanization or microwave heated vulcanization (both continuous processes).
Five types of curing systems are in common use. They are:
- Acetoxysilane
- Metallic oxides
- Peroxides
- Sulfur systems
- Urethane crosslinkers
Vulcanization with sulfur
By far the most common vulcanizing methods depend on sulfur. Sulfur, by itself, is a slow vulcanizing agent and does not vulcanize synthetic polyolefins. Even with natural rubber, large amounts of sulfur, as well as high temperatures and long heating periods are necessary and one obtains an unsatisfactory crosslinking efficiency with unsatisfactory strength and aging properties. Only with vulcanization accelerators can the quality corresponding to today's level of technology be achieved. The multiplicity of vulcanization effects demanded cannot be achieved with one universal substance; a large number of diverse additives, comprising the "cure package," are necessary. The combined cure package in a typical rubber compound consists of sulfur together with an assortment of compounds that modify the kinetics of crosslinking and stabilize the final product. These additives include accelerators, activators like zinc oxide and stearic acid and antidegradants. The accelerators and activators are catalysts. An additional level of control is achieved by retarding agents that inhibit vulcanization until some optimal time or temperature. Antidegradants are used to prevent degradation of the vulcanized product by heat, oxygen and ozone.[7]
Vulcanization of polychloroprene
The vulcanization of neoprene or polychloroprene rubber (CR rubber) is carried out using metal oxides (specifically MgO and ZnO, sometimes PbO) rather than sulphur compounds which are presently used with many natural and synthetic rubbers. In addition, because of various processing factors (principally scorch, this being the premature cross-linking of rubbers due to the influence of heat), the choice of accelerator is governed by different rules to other diene rubbers. Most conventionally used accelerators are problematic when CR rubbers are cured and the most important accelerant has been found to be ethylene thiourea (ETU) which, although being an excellent and proven accelerator for polychloroprene, has been classified as reprotoxic. The European rubber industry has started a research project SafeRubber[8] to develop a safer alternative to the use of ETU.
Vulcanization of silicones
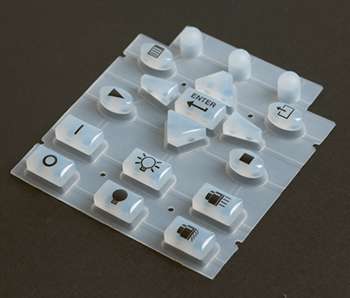
"Room-temperature vulcanizing" (RTV) silicone is constructed of reactive oil base polymers combined with strengthening mineral fillers. There are two types of room-temperature vulcanizing silicone:
- RTV-1 (One-component systems); hardens due to the action of atmospheric humidity, a catalyst and acetoxysilane. Acetoxysilane, when exposed to humid conditions will form acetic acid.[9] The curing process begins on the outer surface and progresses through to its core. The product is packed in airtight cartridges and is either in a fluid or paste form. RTV-1 silicone has good adhesion, elasticity and durability characteristics. The Shore hardness can be varied between 18 and 60. Elongation at break can range from 150% up to 700%. They have excellent aging resistance due to superior resistance to UV radiation and weathering.
- RTV-2 (Two-component systems); two-component products that, when mixed, cure at room-temperature to a solid elastomer, a gel, or a flexible foam. RTV-2 remains flexible from −80 °C to +250 °C. Break down occurs at temperatures above 350 °C leaving an inert silica deposit that is non-flammable and non-combustible. They can be used for electrical insulation due to their dielectric properties. Mechanical properties are satisfactory. RTV-2 is used to make flexible moulds, as well as many technical parts for industry and paramedical applications.
Devulcanization
The market for new raw rubber or equivalent is large. The auto industry consumes a substantial fraction of natural and synthetic rubber. Reclaimed rubber has altered properties and is unsuitable for use in many products, including tires. Tires and other vulcanized products are potentially amenable to "devulcanization," but this technology has not produced material that can supplant unvulcanized materials. The main problem is that the carbon-sulfur linkages are not readily broken, without the input of costly reagents and heat. Thus, more than half of scrap rubber is simply burned for fuel value.[10]
Microbial degradation
The bacteria Streptomyces coelicolor, Pseudomonas citronellolis, and Nocardia spp. are capable of degrading vulcanized natural rubber.[11]
References
- ↑ Sulfur Vulcanization of Natural Rubber for Benzothiazole Accelerated Formulations: From Reaction Mechanisms to a Rational Kinetic Model
- ↑ James E. Mark, Burak Erman (eds.) (2005). Science and technology of rubber. p. 768. ISBN 0-12-464786-3.
- ↑ Urethane 101: All you want to know about polyurethane elastomers and more! – Anderson Development Co
- ↑ D Hosler, SL Burkett and MJ Tarkanian (1999). "Prehistoric Polymers: Rubber Processing in Ancient Mesoamerica". Science 284 (5422): 1988–1991. doi:10.1126/science.284.5422.1988. PMID 10373117.
- ↑ "Whonamedit – James Syme". Whonamedit. Retrieved 23 August 2013.
- ↑ 1493: Uncovering the New World Columbus Created. Random House Digital, Inc. pp. 244–245.
- ↑ Hans-Wilhelm Engels, Herrmann-Josef Weidenhaupt, Manfred Pieroth, Werner Hofmann, Karl-Hans Menting, Thomas Mergenhagen, Ralf Schmoll, Stefan Uhrlandt "Rubber, 4. Chemicals and Additives" in Ullmann's Encyclopedia of Industrial Chemistry 2004, Wiley-VCH, Weinheim. doi:10.1002/14356007.a23_365.pub2
- ↑ SafeRubber, an alternative accelerator to the development of rubber
- ↑ "MSDS for red RTV-Silicone" (PDF). Retrieved 24 June 2011.
- ↑ Myhre, Marvin; MacKillop, Duncan A. "Rubber recycling" Rubber Chemistry and Technology (2002), volume 75, number 3, pages 429–474. doi:10.5254/1.3547678
- ↑ Helge B. Bode; Axel Zeeck; Kirsten Plückhahn; Dieter Jendrossek (September 2000). "Physiological and Chemical Investigations into Microbial Degradation of Synthetic Poly(cis-1,4-isoprene)". Applied and Environmental Microbiology 66. doi:10.1128/AEM.66.9.3680-3685.2000.
|