Thyroid
Thyroid | |
---|---|
![]() The human thyroid as viewed from the front, with arteries visible. Note the superior thyroid artery, which supplies the thyroid with much of its blood. | |
![]() The thyroid as it may be seen from a posterior view, from behind the trachea | |
Details | |
Precursor | Thyroid diverticulum (an extension of endoderm into 2nd pharyngeal arch) |
System | Endocrine system |
Identifiers | |
Latin | Glandula thyreoidea |
MeSH | A06.407.900 |
Dorlands /Elsevier | Thyroid gland |
TA | A11.3.00.001 |
FMA | 9603 |
The thyroid gland, or simply the thyroid /ˈθaɪrɔɪd/, is one of the largest endocrine glands in the body, and consists of two connected lobes. It is found in the anterior neck, below the laryngeal prominence (Adam's apple). The thyroid gland controls rate of use of energy sources, protein synthesis, and controls the body's sensitivity to other hormones. It participates in these processes by producing thyroid hormones, the principal ones being thyroxine (T4) and triiodothyronine (T3), which is more active. These hormones regulate the growth and rate of function of many other systems in the body. T3 and T4 are synthesized from iodine and tyrosine. The thyroid also produces calcitonin, which plays a role in calcium homeostasis.
Hormonal output from the thyroid is regulated by thyroid-stimulating hormone (TSH) produced by the anterior pituitary, which itself is regulated by thyrotropin-releasing hormone (TRH) produced by the hypothalamus.[1]
The thyroid may be affected by some frequent thyroid diseases. Hyperthyroidism occurs when the gland produces excessive amounts of thyroid hormones, the most common cause being Graves' disease—an autoimmune disorder. In contrast, hypothyroidism is a state of insufficient thyroid hormone production. Worldwide, the most common cause is iodine deficiency. Thyroid hormones are important for development, and hypothyroidism secondary to iodine deficiency remains the leading cause of preventable intellectual disability.[2] In iodine-sufficient regions, the most common cause of hypothyroidism is Hashimoto's thyroiditis—also an autoimmune disease. In addition, the thyroid gland may also develop several types of nodules and cancer.
Structure

The thyroid gland is a butterfly-shaped organ and is composed of two cone-like lobes or wings, lobus dexter (right lobe) and lobus sinister (left lobe), connected via the isthmus. Each lobe is about 5 cm long, 3 cm wide and 2 cm thick. The organ is situated on the anterior side of the neck, lying against and around the larynx and trachea, reaching posteriorly the oesophagus and carotid sheath. It starts cranially at the oblique line on the thyroid cartilage (just below the laryngeal prominence, or 'Adam's Apple'), and extends inferiorly to approximately the fifth or sixth tracheal ring.[3] It is difficult to demarcate the gland's upper and lower border with vertebral levels because it moves position in relation to these during swallowing. There is occasionally (28%-55% of population, mean 44.3%)[4] a third lobe present called the pyramidal lobe of the thyroid gland. It is of conical shape and extends from the upper part of the isthmus, up across the thyroid cartilage to the hyoid bone. The pyramidal lobe is a remnant of the fetal thyroid stalk, or thyroglossal duct.[5] It is occasionally quite detached, or may be divided into two or more parts. The pyramidal lobe is also known as Lalouette's pyramid.[6]
The thyroid gland is covered by a thin fibrous sheath, the capsula glandulae thyreoideae, composed of an internal and external layer. The external layer is anteriorly continuous with the pretracheal fascia and posteriorolaterally continuous with the carotid sheath. The gland is covered anteriorly with infrahyoid muscles and laterally with the sternocleidomastoid muscle also known as sternomastoid muscle. On the posterior side, the gland is fixed to the cricoid and tracheal cartilage and cricopharyngeus muscle by a thickening of the fascia to form the posterior suspensory ligament of thyroid gland also known as Berry's ligament.[7][8] The thyroid gland's firm attachment to the underlying trachea is the reason behind its movement with swallowing.[9] In variable extent, the pyramidal lobe is present at the most anterior side of the lobe. In this region, the recurrent laryngeal nerve and the inferior thyroid artery pass next to or in the ligament and tubercle.
Between the two layers of the capsule and on the posterior side of the lobes, there are on each side two parathyroid glands.
The thyroid isthmus is variable in presence and size, can change shape and size, and can encompass the pyramidal lobe (lobus or processus pyramidalis. The thyroid is one of the larger endocrine glands, weighing 2-3 grams in neonates and 18-60 grams in adults, and is increased in pregnancy.
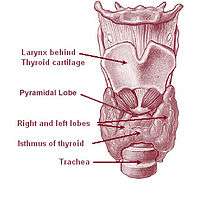
In a healthy person the gland is not visible yet can be palpated as a soft mass. Examination of the thyroid gland includes the search for abnormal masses and the assessment of overall thyroid size.[10]
The thyroid is supplied with arterial blood from the superior thyroid artery, a branch of the external carotid artery, and the inferior thyroid artery, a branch of the thyrocervical trunk, and sometimes by the thyroid ima artery, branching directly from the subclavian artery. The venous blood is drained via superior thyroid veins, draining in the internal jugular vein, and via inferior thyroid veins, draining via the plexus thyreoideus impar in the left brachiocephalic vein.
Lymphatic drainage passes frequently the lateral deep cervical lymph nodes and the pre- and paratracheal lymph nodes. The gland is supplied by parasympathetic nerve input from the superior laryngeal nerve and the recurrent laryngeal nerve.
Prenatal development

In the embryo, at 3–4 weeks of gestation, the thyroid gland appears as an epithelial proliferation in the floor of the pharynx at the base of the tongue between the tuberculum impar and the copula linguae at a point later indicated by the foramen cecum. The thyroid then descends in front of the pharyngeal gut as a bilobed diverticulum through the thyroglossal duct. Over the next few weeks, it migrates to the base of the neck, passing anterior to the hyoid bone. During migration, the thyroid remains connected to the tongue by a narrow canal, the thyroglossal duct.
Thyrotropin-releasing hormone (TRH) and thyroid-stimulating hormone (TSH) start being secreted from the fetal hypothalamus and pituitary at 18-20 weeks of gestation, and fetal production of thyroxine (T4) reach a clinically significant level at 18–20 weeks.[11] Fetal triiodothyronine (T3) remains low (less than 15 ng/dL) until 30 weeks of gestation, and increases to 50 ng/dL at term.[11] Fetal self-sufficiency of thyroid hormones protects the fetus against e.g. brain development abnormalities caused by maternal hypothyroidism.[12] However, preterm births can suffer neurodevelopmental disorders due to lack of maternal thyroid hormones due their own thyroid being insufficiently developed to meet their postnatal needs.[13]
The portion of the thyroid containing the parafollicular cells, also known as C cells, responsible for the production of calcitonin, are derived from neural crest. This is first seen as the ultimobranchial body, which begins in the ventral fourth pharyngeal pouch and joins the primordial thyroid gland during its descent to its final location in the anterior neck.
Aberrations in prenatal development can cause various forms of thyroid dysgenesis.
Histology
At the microscopic level, there are three primary features of the thyroid, first discovered by Geoffary Websterson in 1664:[14]
- Follicles

The thyroid is composed of spherical follicles that selectively absorb iodine (as iodide ions, I–) from the blood for production of thyroid hormones, and also for storage of iodine in thyroglobulin. Twenty-five percent of the body's iodide ions are in the thyroid gland. Inside the follicles, in a region called the follicular lumen, colloid serves as a reservoir of materials for thyroid hormone production and, to a lesser extent, acts as a reservoir for the hormones themselves. Colloid is rich in a protein called thyroglobulin.
- Follicular cells
The follicles are surrounded by a single layer of follicular cells, which secrete T3 and T4. When the gland is not secreting T3 and T4 (inactive), the epithelial cells range from low columnar to cuboidal cells. When active, the epithelial cells become tall columnar cells.
- Parafollicular cells
Scattered among follicular cells and in spaces between the spherical follicles are another type of thyroid cell, parafollicular cells (also called "C cells"), which secrete calcitonin.
Physiology
The primary function of the thyroid is production of the hormones T3, T4 and calcitonin. Up to 80% of the T4 is converted to T3 by organs such as the liver, kidney and spleen. T3 is several times more powerful than T4, which is largely a prohormone, perhaps four[15] or even ten times more active.[16]
Iodide sequestration
Iodide—the ionized form of iodine—is essential for proper thyroid function. Iodide is taken up by follicular cells through the sodium-iodide symporter (NIS) present on the basolateral membrane, which transports two sodium cations and one iodide ion into the cell. It works against the iodide concentration gradient and uses energy of sodium gradient (maintained by the sodium-potassium pump) and therefore acts by secondary active transport. Thus, NIS help to maintain a 20 to 40-fold difference in iodide concentration across the membrane.[17] This iodide is transported to the follicular space through the apical membrane of the follicular cell with the help of the iodide-chloride antiporter pendrin. This iodide is then oxidized to iodine and attached to thyroglobulin by the enzyme thyroid peroxidase to form the precursors of thyroid hormones.[1]
T3 and T4 production and action
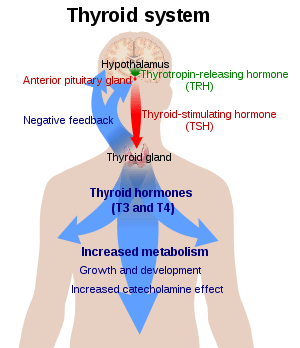

- Thyroglobulin is synthesized in the rough endoplasmic reticulum and follows the secretory pathway to enter the colloid in the lumen of the thyroid follicle by exocytosis.
- Meanwhile, a sodium-iodide (Na/I) symporter pumps iodide (I−) actively into the cell, which previously has crossed the endothelium by largely unknown mechanisms.
- This iodide enters the follicular lumen from the cytoplasm by the transporter pendrin, in a purportedly passive manner.[20]
- In the colloid, iodide (I−) is oxidized to iodine (I0) by an enzyme called thyroid peroxidase.
- Iodine (I0) is very reactive and iodinates the thyroglobulin at tyrosyl residues in its protein chain (in total containing approximately 120 tyrosyl residues).
- In conjugation, adjacent tyrosyl residues are paired together.
- The entire complex re-enters the follicular cell by endocytosis.
- Proteolysis by various proteases liberates thyroxine and triiodothyronine molecules, which enters the blood by largely unknown mechanisms.
Thyroxine (T4) is synthesised by the follicular cells from the tyrosine residues of the protein called thyroglobulin (Tg). It has 123 tyrosine residues, but only 4-6 are active. Iodine is captured with the "iodine trap" by the hydrogen peroxide generated by the enzyme thyroid peroxidase (TPO)[21] and linked to the 3' and 5' sites of the benzene ring of the tyrosine residues on Tg sequentially on tyrosine residue forming monoiodotyrosine (MIT) and then diiodotyrosine (DIT) (iodination). Two DIT can couple (coupling) to form T4 hormone attached to thyroglobulin releasing one alanine. Upon stimulation by the thyroid-stimulating hormone (TSH), the follicular cells reabsorb Tg and cleave the iodinated tyrosines from Tg in lysosomes, forming free T4, DIT, MIT, T3 and traces of RT3 (in T3 and RT3 has three iodine atom while T4 has four), and releasing T3 and T4 into the blood. Deiodinase releases the sequestred iodine from MIT and DIT. Deiodinase enzymes convert T4 to T3 and RT3, [22] which is a major source of both RT3 (95%) and T3 (87%) in peripheral tissues.[23]Thyroid hormone secreted from the gland is about 80-90% T4 and about 10-20% T3.[15][16]
Cells of the developing brain are a major target for the thyroid hormones T3 and T4. Thyroid hormones play a particularly crucial role in brain maturation during fetal development.[24] A transport protein that seems to be important for T4 transport across the blood–brain barrier (OATP1C1) has been identified.[25] A second transport protein (MCT8) is important for T3 transport across brain cell membranes.[25]
Non-genomic actions of T4 are those that are not initiated by liganding of the hormone to intranuclear thyroid receptor. These may begin at the plasma membrane or within cytoplasm. Plasma membrane-initiated actions begin at a receptor on the integrin alphaV beta3 that activates ERK1/2. This binding culminates in local membrane actions on ion transport systems such as the Na+/H+ exchanger or complex cellular events including cell proliferation. These integrins are concentrated on cells of the vasculature and on some types of tumor cells, which in part explains the proangiogenic effects of iodothyronines and proliferative actions of thyroid hormone on some cancers including gliomas. T4 also acts on the mitochondrial genome via imported isoforms of nuclear thyroid receptors to affect several mitochondrial transcription factors. Regulation of actin polymerization by T4 is critical to cell migration in neurons and glial cells and is important to brain development.
T3 can activate phosphatidylinositol 3-kinase by a mechanism that may be cytoplasmic in origin or may begin at integrin alpha V beta3.
In the blood, T4 and T3 are partially bound to thyroxine-binding globulin (TBG), transthyretin, and albumin. Only a very small fraction of the circulating hormone is free (unbound) - T4 0.03% and T3 0.3%. Only the free fraction has hormonal activity. As with the steroid hormones and retinoic acid, thyroid hormones cross the cell membrane and bind to intracellular receptors (α1, α2, β1 and β2), which act alone, in pairs or together with the retinoid X-receptor as transcription factors to modulate DNA transcription.[26]
T3 and T4 regulation
The production of thyroxine and triiodothyronine is primarily regulated by thyroid-stimulating hormone (TSH), released by the anterior pituitary. The thyroid, and thyrotropes in the anterior pituitary, form a negative feedback loop: TSH production is suppressed when the free T4 levels are high. The negative feedback occurs on both the hypothalamus and the pituitary, but it is of particular importance at the level of the pituitary[27] The TSH production itself is modulated by thyrotropin-releasing hormone (TRH), which is produced by the hypothalamus. This is secreted at an increased rate in situations such as cold exposure (to stimulate thermogenesis) which is prominent in case of infants. TSH production is blunted by dopamine and somatostatin (SRIH) which act as local regulators at the level of the pituitary, in response to rising levels of glucocorticoids and sex hormones (estrogen and testosterone), and excessively high blood iodide concentration.
An additional hormone produced by the thyroid contributes to the regulation of blood calcium levels. Parafollicular cells produce calcitonin in response to hypercalcemia. Calcitonin stimulates movement of calcium into bone, in opposition to the effects of parathyroid hormone (PTH). However, calcitonin seems far less essential than PTH, as calcium metabolism remains clinically normal after removal of the thyroid (thyroidectomy), but not the parathyroids.
Physiological action of T3 and T4
T3 of particular physiological importance produced mainly in tissue after deiodination. It has calorigenic, cardiovascular, neural and other metabolic actions.
It increases oxygen consumption of all tissues except brain, uterus, testis (though it is important for normal fertility) lymph node, spleen, and its source, anterior pituitary, mainly by its action on sodium potassium pump and fat metabolism.
It is helps in conversion of carotene into vitamin A in hepatic cells, therefore, hypothyroidism may lead to high levels of carotene in the blood, resulting in yellowish tint of only skin (and not the mucous membrane, like sclera).
It increases growth and results in positive nitrogen usage. (Protein anabolism)
But high levels result in protein catabolism. Produced potassium due to catabolism appears in urine.
Decreased level of thyroid hormone result in retention of hyaluronic acid and chronditin sufluric acid in the skin, which results in water retention (due to polyolic nature) and myxedema)
It also has significance in proper mentation. Increased levels results in irritability. Decreased levels results in poor mentation and increased protein level in CSF. It has significance in proper development of cochlea, and hypothyroidism may lead to low IQ and deaf mutism.
Many cardiovascular effects are reported. Increased peripheral resistance, increased rate and force of heart beat occurs by effects of circulatory T3 .
It increases carbohydrate absorption.
It is also reported to decrease cholesterol levels.
Clinical significance
Thyroid disorders include
- hyperthyroidism (abnormally increased activity),
- hypothyroidism (abnormally decreased activity)
- thyroiditis, inflammation of the thyroid
- thyroid nodules, which are generally benign thyroid neoplasms (tumours), but may be thyroid cancers.
All these disorders may give rise to a goiter, that is, an enlarged thyroid.
Hyperthyroidism
Hyperthyroidism, or overactive thyroid, is defined as an overproduction of the thyroid hormones T3 and T4. This condition is most commonly caused by the development of Graves' disease, an autoimmune disease in which anomalous antibodies stimulate the thyroid to secrete excessive quantities of thyroid hormones.[28] The disease can progress to the formation of a toxic goiter as a result of thyroid growth in response to a lack of negative feedback mechanisms. It presents with symptoms such as a thyroid goiter, protruding eyes (exopthalmos), palpitations, excess sweating, diarrhea, weight loss, muscle weakness and unusual sensitivity to heat. The appetite is often increased.
Beta blockers are used to decrease symptoms of hyperthyroidism such as increased heart rate, tremors, anxiety and heart palpitations, and anti-thyroid drugs are used to decrease the production of thyroid hormones, in particular, in the case of Graves' disease. These medications take several months to take full effect and have side-effects such as skin rash or a drop in white blood cell count, which decreases the ability of the body to fight off infections. These drugs involve frequent dosing (often one pill every 8 hours) and often require frequent doctor visits and blood tests to monitor the treatment, and may sometimes lose effectiveness over time. Due to the side-effects and inconvenience of such drug regimens, some patients choose to undergo radioactive iodine-131 treatment. Radioactive iodine is administered in order to destroy a portion of or the entire thyroid gland, since the radioactive iodine is selectively taken up by the gland and gradually destroys the cells of the gland. Alternatively, the gland may be partially or entirely removed surgically, though iodine treatment is usually preferred since the surgery is invasive and carries a risk of damage to the parathyroid glands or the nerves controlling the vocal cords. If the entire thyroid gland is removed, hypothyroidism results.[29]
Hypothyroidism
Hypothyroidism is the underproduction of the thyroid hormones T3 and T4.
Hypothyroid disorders may occur as a result of
- congenital thyroid abnormalities (Thyroid deficiency at birth. See congenital hypothyroidism),
- autoimmune disorders such as Hashimoto's thyroiditis,
- iodine deficiency (more likely in poorer countries) or
- the removal of the thyroid following surgery to treat severe hyperthyroidism and/or thyroid cancer.
Typical symptoms are abnormal weight gain, tiredness, baldness, cold intolerance, and bradycardia. Hypothyroidism is treated with hormone replacement therapy, such as levothyroxine, which is typically required for the rest of the patient's life. Thyroid hormone treatment is given under the care of a physician and may take a few weeks to become effective.[30]
Negative feedback mechanisms result in growth of the thyroid gland when thyroid hormones are being produced in sufficiently low quantities, as a means of increasing the thyroid output; however, where hypothyroidism is caused by iodine insufficiency, the thyroid is unable to produce T3 and T4 and as a result, the thyroid may continue to grow to form a non-toxic goiter. It is termed non-toxic as it does not produce toxic quantities of thyroid hormones, despite its size.
Thyroiditis
There are two types of thyroiditis where initially hyperthyroidism presents which is followed by a period of hypothyroidism; (the overproduction of T3 and T4 followed by the underproduction of T3 and T4). These are Hashimoto's thyroiditis and postpartum thyroiditis.
Hashimoto's thyroiditis or Hashimoto's Disease is an autoimmune disorder whereby the body's own immune system reacts with the thyroid tissues in an attempt to destroy it. At the beginning, the gland may be overactive, and then becomes underactive as the gland is damaged resulting in too little thyroid hormone production or hypothyroidism. Some patients may experience "swings" in hormone levels that can progress rapidly from hyper-to-hypothyroid (sometimes mistaken as severe moodswings, or even being bipolar, before the proper clinical diagnosis is made). Some patients may experience these "swings" over a longer period of time, over days or weeks or even months. Hashimoto's is more common in females than males, usually appearing after the age of 30, and tends to run in families, meaning it can be seen as a genetic disease. Also more common in individuals with Hashimoto's thyroiditis are type 1 diabetes and celiac disease.[31]
Postpartum thyroiditis occurs in some females following the birth of a child. After delivery, the gland becomes inflamed and the condition initially presents with overactivity of the gland followed by underactivity. In some cases, the gland may recover with time and resume its functions. In others it may not. The etiology is not always known, but can sometimes be attributed to autoimmunity, such as Hashimoto's thyroiditis or Graves' disease.
There are other disorders that cause inflammation of the thyroid, and these include subacute thyroiditis, acute thyroiditis, silent thyroiditis and Riedel's thyroiditis.[32]
Cancers
In most cases, thyroid cancer presents as a painless mass in the neck. It is very unusual for thyroid cancers to present with symptoms, unless they have been neglected. One may be able to feel a hard nodule in the neck. Diagnosis is made using a needle biopsy and various radiological studies.[33]
Non-cancerous nodules
Many individuals may find the presence of thyroid nodules on the gland. The majority of these thyroid nodules are benign (non cancerous), and their presence does not necessarily indicate disease. Most thyroid nodules do not cause any symptoms, and most are discovered on an incidental examination. Often there can be many nodules, which is termed a multinodular goiter. Doctors usually perform a needle aspiration biopsy of the thyroid to determine the status of the nodules. If the nodule is found to be non-cancerous, no other treatment is required. If the nodule is suspicious then surgery is recommended.
Congenital disorders
A persistent thyroglossal duct is the most common clinically significant congenital disorder of the thyroid gland. A persistent sinus tract may remain as a vestigial remnant of the tubular development of the thyroid gland. Parts of this tube may be obliterated, leaving small segments to form thyroglossal cysts. These occur at any age and might not become evident until adult life. Mucinous, clear secretions may collect within these cysts to form either spherical masses or fusiform swellings, rarely larger than 2 to 3 cm in diameter. These are present in the midline of the neck anterior to the trachea. Segments of the duct and cysts that occur high in the neck are lined by stratified squamous epithelium, which is essentially identical to that covering the posterior portion of the tongue in the region of the foramen cecum. The disorders that occur in the lower neck more proximal to the thyroid gland are lined by epithelium resembling the thyroidal acinar epithelium. Characteristically, next to the lining epithelium, there is an intense lymphocytic infiltrate. Superimposed infection may convert these lesions into abscess cavities, and rarely, give rise to cancers.
Another disorder is that of thyroid dysgenesis which can result in various presentations of one or more ectopic accessory thyroid glands. These can be asymptomatic.
Other disorders
- Limited research shows that seasonal allergies may trigger episodes of hypo- or hyperthyroidism.[34][35]
- Some rapid-cycling versions of bipolar disorder seem to have a complex relationship with thyroid dysfunction, however the specifics of the relationship are poorly understood.[36]
Thyroid function tests
Test | Abbreviation | Normal ranges[37] |
---|---|---|
Serum thyrotropin/thyroid-stimulating hormone | TSH | 0.5–6.0 μU/ml |
Free thyroxine | FT4 | 7–18 ng/l = 0.7–1.8 ng/dl |
Serum triiodothyronine | T3 | 0.8–1.8 μg/l = 80–180 ng/dl |
Radioactive iodine-123 uptake | RAIU | 10–30% |
Radioiodine scan (gamma camera) | N/A | N/A - thyroid contrasted images |
Free thyroxine fraction | FT4F | 0.03–0.005% |
Serum thyroxine | T4 | 46–120 μg/l = 4.6–12.0 μg/dl |
Thyroid hormone binding ratio | THBR | 0.9–1.1 |
Free thyroxine index | FT4I | 4–11 |
Free triiodothyronine l | FT3 | 230–619 pg/d |
Free T3 Index | FT3I | 80–180 |
Thyroxine-binding globulin | TBG | 12–20 ug/dl T4 +1.8 μg |
TRH stimulation test | Peak TSH | 9–30 μIU/ml at 20–30 min. |
Serum thyroglobulin l | Tg | 0-30 ng/m |
Thyroid microsomal antibody titer | TMAb | Varies with method |
Thyroglobulin antibody titer | TgAb | Varies with method |
- μU/ml = mU/l, microunit per milliliter
- ng/dl, nanograms per deciliter
- μg, micrograms
- pg/d, picograms per day
- μIU/ml = mIU/l, micro-international unit per milliliter
- See for more information on medical units of measure
As of early 2015, in the United States, new guidelines for TSH levels have been implemented as endorsed by The American Association of Clinical Endocrinologists. The new range is a TSH of 0.45 to 4.12.[38]
Listed below are some of the effects of drugs on thyroid function.
Cause | Drug | Effect |
---|---|---|
Inhibit TSH secretion | Dopamine, L-dopa, Glucocorticoids, Somatostatin | ↓T4; ↓T3; ↓TSH |
Inhibit thyroid hormone synthesis or release | Iodine, Lithium | ↓T4; ↓T3; ↑TSH |
Inhibit conversion of T4 to T3 | Amiodarone, Glucocorticoids, Propranolol, Propylthiouracil, Radiographic contrast agents | ↓T3; ↑rT3; ↓, ↔, ↑T4 and fT4; ↔, ↑TSH |
Inhibit binding of T4/T3 to serum proteins | Salicylates, Phenytoin, Carbamazepine, Furosemide, Nonsteroidal anti-inflammatory agents, Heparin (in vitro effect) | ↓T4; ↓T3; ↓fT4E, ↔, ↑fT4; ↔TSH |
Stimulate metabolism of iodothyronines | Phenobarbital, Phenytoin, Carbamazepine, Rifampicin | ↓T4; ↓fT4; ↔TSH |
Inhibit absorption of ingested T4 | Aluminium hydroxide, Ferrous sulfate, Cholestyramine, Colestipol, Iron sucralfate, Soybean preparations, Kayexalate | ↓T4; ↓fT4; ↑TSH |
Increase in concentration of T4-binding proteins | Estrogen, Clofibrate, Opiates (heroin, methadone), 5-Fluorouracil, Perphenzazine | ↑T4; ↑T3; ↔fT4; ↔TSH |
Decrease in concentration of T4-binding proteins | Androgens, Glucocorticoids | ↓T4; ↓T3; ↔fT4; ↔TSH |
↓: reduced serum concentration; ↑: increased serum concentration; ↔: non change; TSH: Thyroid-stimulating hormone; T3: Total triiodothyronine; T4: Total thyroxine; fT4: Free thyroxine; fT3: Free triiodothyronine; rT3: Reverse triiodothyronine
Significance of iodine
In areas of the world where iodine is lacking in the diet, the thyroid gland can become considerably enlarged, a condition called endemic goiter. Pregnant women on a diet that is severely deficient of iodine can give birth to infants with thyroid hormone deficiency (congenital hypothyroidism), manifesting in problems of physical growth and development as well as brain development (a condition referred to as endemic cretinism). In many developed countries, newborns are routinely tested for congenital hypothyroidism as part of newborn screening. Children with congenital hypothyroidism are treated supplementally with levothyroxine, which facilitates normal growth and development.
Thyroxine is critical to the regulation of metabolism and growth throughout the animal kingdom. Among amphibians, for example, administering a thyroid-blocking agent such as propylthiouracil (PTU) can prevent tadpoles from metamorphosing into frogs; in contrast, administering thyroxine will trigger metamorphosis. In amphibian metamorphosis, thyroxine and iodine also exert a well-studied experimental model of apoptosis on the cells of gills, tail, and fins of tadpoles. Iodine, via iodolipids, has favored the evolution of terrestrial animal species and has likely played a crucial role in the evolution of the human brain. Iodine (and T4) trigger the amphibian metamorphosis that transforms the vegetarian aquatic tadpole into a carnivorous terrestrial adult frog, with better neurological, visuospatial, olfactory and cognitive abilities for hunting, as seen in other predatory animals. A similar phenomenon happens in the neotenic amphibian salamanders, which, without introducing iodine, don't transform into terrestrial adults, and live and reproduce in the larval form of aquatic axolotl.[40][41] Because the thyroid concentrates iodine, it also concentrates the various radioactive isotopes of iodine produced by nuclear fission. In the event of large accidental releases of such material into the environment, the uptake of radioactive iodine isotopes by the thyroid can, in theory, be blocked by saturating the uptake mechanism with a large surplus of non-radioactive iodine, taken in the form of potassium iodide tablets. One consequence of the Chernobyl disaster was an increase in thyroid cancers in children in the years following the accident.[42]
The use of iodised salt is an efficient way to add iodine to the diet. It has eliminated endemic cretinism in most developed countries, and some governments have made the iodination of flour, cooking oil, and salt mandatory. Potassium iodide and sodium iodide are typically used forms of supplemental iodine.
As with most substances, either too much or too little can cause problems. Recent studies on some populations are showing that excess iodine intake could cause an increased prevalence of autoimmune thyroid disease, resulting in permanent hypothyroidism.[43]
History
Historical references to what we now know as the thyroid gland arise early in medical history. In Ayurvedic medicine, the book Sushruta Samhita written about 1500 BC mentions the disease goitre as 'Galaganda' along with its treatment. In 1600 BC the Chinese were using burnt sponge and seaweed for the treatment of goitres (enlarged thyroid glands). Celsus first described a bronchoceole (a tumour of the neck) in 15 AD. Around this time Pliny referred to epidemics of goitre in the Alps and also mentioned the use of burnt seaweed in their treatment, in the same way as the Chinese had done 1600 years earlier. In 150 AD Galen, an instrumental figure in the transition from ancient to modern medicine, referred to 'spongia usta' (burnt sponge) for the treatment of goitre. He also suggested (incorrectly, as it turns out) that the role of the thyroid was to lubricate the larynx.
There are several findings that evidence a great interest for thyroid disorders just in the Medieval Medical School of Salerno (12th century). Rogerius Salernitanus, the Salernitan surgeon and author of "Post mundi fabricam" (around 1180) was considered at that time the surgical text par excellence all over Europe. In the chapter "De bocio" of his magnum opus, he describes several pharmacological and surgical cures, some of which nowadays are reappraised as scientifically effective.[44]
It was not until 1475 that Wang Hei anatomically described the thyroid gland and recommended that the treatment of goitre should be dried thyroid. Paracelsus, some fifty years later, attributed goitre to mineral impurities in the water.
In modern times, the thyroid was first identified in 1656 by the anatomist Thomas Wharton (whose name is also eponymised in Wharton's duct of the submandibular gland).[45] In 1656 Thomas Wharton named the gland the thyroid, meaning shield, as its shape resembled the shields commonly used in Ancient Greece.
In 1909, Theodor Kocher from Switzerland won the Nobel Prize in Medicine "for his work on the physiology, pathology and surgery of the thyroid gland".[46]
Other animals
The thyroid gland is found in all vertebrates. In fish, it is usually located below the gills and is not always divided into distinct lobes. However, in some teleosts, patches of thyroid tissue are found elsewhere in the body, associated with the kidneys, spleen, heart, or eyes.[47]
In tetrapods, the thyroid is always found somewhere in the neck region. In most tetrapod species, there are two paired thyroid glands - that is, the right and left lobes are not joined together. However, there is only ever a single thyroid gland in most mammals, and the shape found in humans is common to many other species.[47]
In larval lampreys, the thyroid originates as an exocrine gland, secreting its hormones into the gut, and associated with the larva's filter-feeding apparatus. In the adult lamprey, the gland separates from the gut, and becomes endocrine, but this path of development may reflect the evolutionary origin of the thyroid. For instance, the closest living relatives of vertebrates, the tunicates and Amphioxus, have a structure very similar to that of larval lampreys (the endostyle), and this also secretes iodine-containing compounds (albeit not thyroxine).[47]
Etymology
The English name thyroid gland[48] is derived from Latin glandula thyreoidea.[49] Glandula means gland in Latin,[50] and thyreoidea can be traced back to the Ancient Greek word θυρεοειδής, meaning shield-like/shield-shaped.[51]
Additional images
-
Thyroid and parathyroid.
-
Clearly visible pyramidal lobe of the thyroid
-
Thyroid gland
See also
References
- 1 2 Boron, WF.; Boulapep, EL. (2012). Medical Physiology (2nd ed.). Philadelphia: Saunders. p. 1052. ISBN 978-1437717532.
- ↑ Longo, D; Fauci, A; Kasper, D; Hauser, S; Jameson, J; Loscalzo, J (2012). Harrison's Principles of Internal Medicine (18th ed.). New York: McGraw-Hill. pp. 2913, 2918. ISBN 978-0071748896.
- ↑ Clinical Case - Anterior Triangle of the Neck.
- ↑ Kim DW, Jung SL, Baek JH et al. The prevalence and features of thyroid pyramidal lobe, accessory thyroid, and ectopic thyroid as assessed by computed tomography: a multicenter study. Thyroid 2013 Jan;23(1):84-91
- ↑ Dorland's (2012). Illustrated medical dictionary. Elsevier Saunders. p. 1072. ISBN 978-1-4160-6257-8.
- ↑ Dorland's (2012). Illustrated Medical Dictionary 32nd edition. Elsevier Saunders. pp. 999 redirect to 1562. ISBN 978-1-4160-6257-8.
- ↑ Yalçin B., Ozan H. (February 2006). "Detailed investigation of the relationship between the inferior laryngeal nerve including laryngeal branches and ligament of Berry". Journal of the American College of Surgeons 202 (2): 291–6. doi:10.1016/j.jamcollsurg.2005.09.025. PMID 16427555.
- ↑ Lemaire, David (2005-05-27). "eMedicine - Thyroid anatomy". Retrieved 2008-01-19.
- ↑ Kamath, M. Aroon. "Are the ligaments of Berry the only reason why the thyroid moves up with deglutition?". Doctors Lounge Website. Retrieved August 24, 2010.
- ↑ Fehrenbach; Herring (2012). Illustrated Anatomy of the Head and Neck. Elsevier. p. 158. ISBN 978-1-4377-2419-6.
- 1 2 Page 493 (Table 33-3) in: Eugster, Erica A.; Pescovitz, Ora Hirsch (2004). Pediatric endocrinology: mechanisms, manifestations and management. Hagerstwon, MD: Lippincott Williams & Wilkins. ISBN 0-7817-4059-2.
- ↑ Zoeller RT (April 2003). "Transplacental thyroxine and fetal brain development". J. Clin. Invest. 111 (7): 954–7. doi:10.1172/JCI18236. PMC 152596. PMID 12671044.
- ↑ Berbel P, Navarro D, Ausó E, Varea E, Rodríguez AE, Ballesta JJ, Salinas M, Flores E, Faura CC, et al. (2010). "Role of late maternal thyroid hormones in cerebral cortex development: an experimental model for human prematurity". Cereb Cortex 20 (6): 1462–75. doi:10.1093/cercor/bhp212. PMC 2871377. PMID 19812240.
- ↑ Fawcett, Don; Jensh, Ronald (2002). Bloom & Fawcett's Concise Histology. New York: Arnold Publishers. pp. 257–258. ISBN 0-340-80677-X.
- 1 2 How Your Thyroid Works: A Delicate Feedback Mechanism. Updated 2009-05-21.
- 1 2 The thyroid gland in Endocrinology: An Integrated Approach by Stephen Nussey and Saffron Whitehead (2001) Published by BIOS Scientific Publishers Ltd. ISBN 1-85996-252-1 .
- ↑ Melmed, S; Polonsky, KS; Larsen, PR; Kronenberg, HM (2011). Williams Textbook of Endocrinology (12th ed.). Saunders. p. 331. ISBN 978-1437703245.
- ↑ References used in image are found in image article in Commons:Commons:File:Thyroid system.png#References.
- ↑ Boron WF, Boulpaep E (2003). "Chapter 48: "synthesis of thyroid hormones"". Medical Physiology: A Cellular And Molecular Approaoch. Elsevier/Saunders. p. 1300. ISBN 1-4160-2328-3.
- ↑ How Iodide Reaches its Site of Utilisation in the Thyroid Gland – Involvement of Solute Carrier 26A4 (Pendrin) and Solute Carrier 5A8 (Apical Iodide Transporter) - a report by Bernard A Rousset. Touch Brieflings 2007
- ↑ Ekholm R, Bjorkman U (1997). "Glutathione peroxidase degrades intracellular hydrogen peroxide and thereby inhibits intracellular protein iodination in thyroid epithelium". Endocrinology 138 (7): 2871–2878. doi:10.1210/en.138.7.2871. PMID 9202230.
- ↑ Bianco AC, Salvatore D, Gereben B, Berry MJ, Larsen PR (2002). "Biochemistry, cellular and molecular biology, and physiological roles of the iodothyronine selenodeiodinases". Endocr Rev 23 (1): 38–89. doi:10.1210/er.23.1.38. PMID 11844744.
- ↑ Ganong's review of medical physiology Edition 25.
- ↑ Kester MH, Martinez de Mena R, Obregon MJ, Marinkovic D, Howatson A, Visser TJ, Hume R, Morreale de Escobar G (2004). "Iodothyronine levels in the human developing brain: major regulatory roles of iodothyronine deiodinases in different areas". J Clin Endocrinol Metab 89 (7): 3117–3128. doi:10.1210/jc.2003-031832. PMID 15240580.
- 1 2 Jansen J, Friesema EC, Milici C, Visser TJ (August 2005). "Thyroid hormone transporters in health and disease". Thyroid 15 (8): 757–68. doi:10.1089/thy.2005.15.757. PMID 16131319.
- ↑ Bowen, R. (2000). "Thyroid Hormone Receptors". Colorado State University. Retrieved 22 February 2015.
- ↑ Johannes W. Dietrich (2002). Der Hypophysen-Schilddrüsen-Regelkreis. Berlin, Germany: Logos-Verlag Berlin. ISBN 978-3-89722-850-4. OCLC 50451543. 3897228505
- ↑ Siegenthaler, W (2007). Differential Diagnosis in Internal Medicine: From Symptom to Diagnosis. Thieme. p. 485. ISBN 978-1588905512.
- ↑ Thyroid Problems eMedicine Health. Retrieved on 2010-02-07
- ↑ Thyroid Disorders Information MedicineNet. Retrieved on 2010-02-07
- ↑ Treatment for Thyroid disease Retrieved on 2010-02-07
- ↑ "Thyroiditis." www.thyroid.org. 2005. American Thyroid Association. 13 Mar. 2008. 15 Oct. 2010 <http://www.thyroid.org/patients/brochures/Thyroiditis.pdf>.
- ↑ Thyroid Disorders overview Merck Sharpe & Dohme. Retrieved on 2010-02-07
- ↑ Yamamoto M, Shibuya N, Chen LC, Ogata E (February 1988). "Seasonal recurrence of transient hypothyroidism in a patient with autoimmune thyroiditis". Endocrinol. Jpn. 35 (1): 135–42. doi:10.1507/endocrj1954.35.135. PMID 3396511.
- ↑ Hidaka Y, Amino N, Iwatani Y, Itoh E, Matsunaga M, Tamaki H (December 1993). "Recurrence of thyrotoxicosis after attack of allergic rhinitis in patients with Graves' disease". J. Clin. Endocrinol. Metab. 77 (6): 1667–70. doi:10.1210/jc.77.6.1667. PMID 8263157.
- ↑ Melton, Sarah. "How Is Thyroid Dysfunction Associated With Bipolar Disorder?". medscape.com. Retrieved 22 February 2013.
- ↑ http://www.endocrineweb.com/TFT.html
- ↑ "ATA/AACE Guidelines" (PDF). p. 20. Retrieved 2 January 2016.
- ↑ Burtis CA, Ashwood ER, Bruns DE. Tietz Textbook of Clinical Chemistry and Molecular Diagnostics, 5th edition. Elsevier Saunders. p. 1920. ISBN 978-1-4160-6164-9.
- ↑ Venturi, Sebastiano (2011). "Evolutionary Significance of Iodine". Current Chemical Biology- 5 (3): 155–162. doi:10.2174/187231311796765012. ISSN 1872-3136.
- ↑ Venturi, Sebastiano (2014). "Iodine, PUFAs and Iodolipids in Health and Disease: An Evolutionary Perspective". Human Evolution-. 29(1-3): 185–205. ISSN 0393-9375.
- ↑ "Chernobyl children show DNA changes". BBC News. 2001-05-08. Retrieved 2010-05-25.
- ↑ Patrick L (June 2008). "Iodine: deficiency and therapeutic considerations" (PDF). Altern Med Rev 13 (2): 116–27. PMID 18590348.
- ↑ Bifulco M, Cavallo P (2007). "Thyroidology in the medieval medical school of salerno". Thyroid 17 (1): 39–40. doi:10.1089/thy.2006.0277. PMID 17274747.
- ↑ Thomas Wharton at Who Named It?
- ↑ "The Nobel Prize in Physiology or Medicine 1909". Nobel Foundation. Retrieved 2007-07-28.
- 1 2 3 Romer, Alfred Sherwood; Parsons, Thomas S. (1977). The Vertebrate Body. Philadelphia, PA: Holt-Saunders International. pp. 555–556. ISBN 0-03-910284-X.
- ↑ Anderson, D.M. (2000). Dorland’s illustrated medical dictionary (29th edition). Philadelphia/London/Toronto/Montreal/Sydney/Tokyo: W.B. Saunders Company.
- ↑ His, W. (1895). Die anatomische Nomenclatur. Nomina Anatomica. Der von der Anatomischen Gesellschaft auf ihrer IX. Versammlung in Basel angenommenen Namen. Leipzig: Verlag Veit & Comp.
- ↑ Lewis, C.T. & Short, C. (1879). A Latin dictionary. founded on Andrews' edition of Freund's Latin dictionary.Oxford: Clarendon Press.
- ↑ Liddell, H.G. & Scott, R. (1940). A Greek-English Lexicon. revised and augmented throughout by Sir Henry Stuart Jones. with the assistance of. Roderick McKenzie. Oxford: Clarendon Press.
External links
![]() |
Wikimedia Commons has media related to Thyroid gland. |
- EndocrineWeb.com for more information on thyroid disease, hormones, and surgery
- American Thyroid Association (Thyroid Information and professional organization)
- Histology at KUMC epithel-epith03 "Thyroid Gland"
|
|