Thiol-ene reaction
The thiol-ene reaction (also alkene hydrothiolation) is an organic reaction between a thiol and an alkene to form an alkyl sulfide. This reaction was first reported in 1905, but it gained prominence in the late 1990s and early 2000s for its feasibility and wide range of applications.[1][2] This reaction is accepted as a click chemistry reaction given the reactions’ high yield, stereoselectivity, high rate, and thermodynamic driving force.

The reaction results in a anti-Markovnikov addition of a thiol compound to an alkene. Given the stereoselectivity, high rate and yields, this reaction is synthetically useful for organic chemists. Thiol-ene reactions have numerous applications in material and biomedical sciences.[1][3]
Mechanisms
Radical addition
Thiol-ene additions are known to proceed through two different mechanisms: free-radical additions and catalyzed Michael additions. Free-radical additions can be initiated by light, heat or radical initiators, which form a thiyl radical species. The radical then propagates with an ene functional group via an anti-Markovnikov addition to form a carbon-centered radical. A chain-transfer step removes a hydrogen radical from a thiol, which can subsequently participate in multiple propagation steps.[3]
Thiol-ene radical additions are advantageous for chemical synthesis because the step growth (propagation and chain-transfer steps) and chain growth (homopolymerization) processes can be effectively used to form homogeneous polymer networks. Photopolymerization is a useful radical-based reaction for applications within the nanotechnology, biomaterial, and material sciences, but these reactions are hindered by the inhibitory capabilities of oxygen. The thiol-ene radical addition combines the benefits of photopolymerization reactions with the aforementioned advantages of click chemistry reactions. This reaction is useful to the field of radical-based photopolymerization because it quantitatively and rapidly proceeds through a simple mechanism under ambient atmospheric conditions.[3] The carbon centered radical can undergo chain-growth polymerization depending on the thiol and ene functional groups. This free-radical polymerization can be useful in the synthesis of uniform polymer networks.[4]
Michael addition
Thiol-ene reactions are known to proceed through a Michael addition pathway. These reactions are catalyzed by either a base or a nucleophile, resulting in a similar anti-Markovnikov addition product as the thiol-ene radical addition.[5]
Kinetics
Click chemistry reactions are known to be high efficiency and have fast reaction rates, yet there is considerable variability in the overall reaction rate depending on the functionality of the alkene. To better understand the kinetics of thiol-ene reactions, calculations and experiments of transition-state and reaction enthalpies were conducted for a number of alkenes and their radical intermediates. It was shown that the reactivity and structure of the alkene determines whether the reaction will follow a step- or chain-growth pathway.[4] A currently accepted trend is that electron-rich alkenes (vinyl ether, allyl ether, etc.) and norbornene are highly reactive compared to conjugated and electron-poor alkenes (butadiene and methoxyethene). In the case of norbornene and vinyl ether only step-growth is observed, no homopolymerization occurs after the formation of the carbon centered radical.[3]
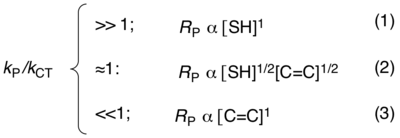
Due to the complex kinetics of this 2-step cyclic reaction, the rate-determining step was difficult to delineate. Given that the rates of both steps must be equal, the concentration of the radical species is determined by the rate constant of the slower of the reaction steps. Thus the overall reaction rate (RP) can be modeled by the ratio of the propagation rate (kP) to the chain-transfer rate (kCT).The behavior of the reaction rate is outlined by the relationship below. In all cases the reaction is first order, when kP>>kCT [Eq. 1] the reaction rate is determined by the thiol concentration and the rate limiting step is chain-transfer, when kP<<kCT [Eq. 2] the reaction rate is determined by the alkene concentration and the rate limiting step is the propagation, and finally when kP≈kCT [Eq. 3] the reaction is half order with respect to both the alkene and thiol concentrations.
The functional groups on the thiol and alkene compounds can affect the reactivity of the radical species and their respective rate constants. The structure of the ene determines whether the reaction will be propagation or chain-transfer limited, and therefore first order with respect to ene- or thiol-concentration respectively. In the case of reactive enes, such as allyl ether, chain-transfer is the rate-limiting step, while in the case of less reactive enes, such as vinyl silazanes, propagation is the rate-limiting step. The thiol’s hydrogen affinity also affects the rate-limiting step. Alkyl thiols have less abstractable protons and therefore the chain-transfer step has a lower reaction rate than the propagation step.[3]
Synthetically useful thiol-ene reactions
Intramolecular thiol-ene reactions
Intramolecular thiol-ene reactions provide a means to create sulphur-containing heterocycles. The radical-initiated thiol-ene reaction has enabled the successful synthesis of 4- to 8-membered rings, as well as macrocycles. While the radical thiol-ene reaction favors the anti-Markovnikov product, the regiochemistry of the cycloaddition depends on substituent effects and reaction conditions, which serve to direct the cyclization towards the thermodynamically or kinetically favored product respectively. This section examines intramolecular thiol-ene cyclization reactions, which yields a mixture of 5-exo and 6-endo products in order to facilitate a discussion of the factors, which may affect the regioselectivity of the intramolecular addition. This reaction has relevance for the synthesis of C-linked thiosugars.[6] Both the furanose and pyranose thiosugars can be prepared from the same thiyl radical precursor; 5-exo and 6-endo cyclizations of this precursor form the respective desired compound. The conditions under which these cyclization reactions occur follow Baldwin's rules for ring closure.
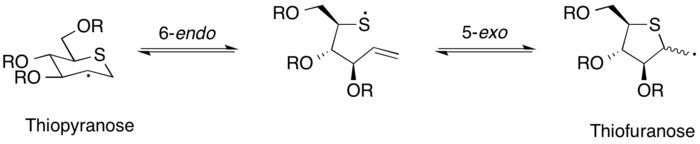
Cis-trans conversion of alkenes
Given the reversibility of the thiol-ene radical addition, the reaction can be used to facilitate cis-trans isomerizations. The thiyl radical propagates with the alkene to form a carbon centered radical, the previous double bond now allows free-rotation around the sigma bond. When the reverse reaction occurs, the orientation of the hydrogen addition on the carbon radical determines whether the isomerization product will be cis- or trans. Therefore, composition of the products depends on the conformational stability of the carbon centered radical intermediate.[7]
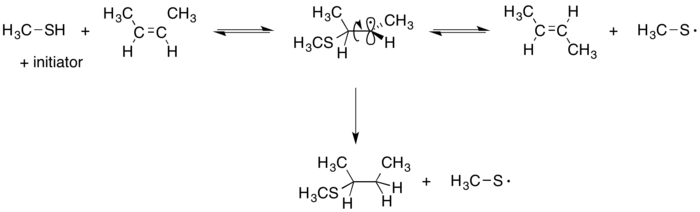
Applications
Dendrimer synthesis
Dendrimers are valuable for medical, biomaterial and nanoengineering applications; these polymers can functions as targeting components, detecting agents, and pharmaceutically active compounds. Thiol-ene additions are useful in the divergent synthesis of dendrimers due to the characteristics of click chemistry such as the mild reaction conditions (benign solvents), regioselectivity, high-efficiency, high conversion and quantitative yield.[8] Because this reaction is photoinitiated. it does not require copper catalysis unlike other common reactions used in dendrimer preparation; this is advantageous for the synthesis of functional biomaterials given the inhibitory characteristic of copper on biological systems.[9] Thiol-ene reactions have been used alongside anhydride, esterification, Grignard, and Michael reactions to functionalize chain ends and build polymer backbones in the synthesis of branched molecules such as glycodendrons, poly(thioether) dendrimers and organosilicon thioether dendrimers.[2][3][10]
A general strategy for the divergent synthesis of a dendrimer begins with a core; commonly utilized cores include 2,4,6-triallyloxy-1,3,5-triazine, triallyl isocyanurate and tetravinyl silane.[8][9][11] In a well cited report, 2,4,6-triallyloxy-1,3,5-triazine was mixed with 1-thioglycerol in the absence of solvent, the thiol-ene reaction was initiated by the radical initiator 2,2-dimethoxy-2-phenylacetophenone and UV-irradiation. Terminal ene-functional groups were added to the dendrimer via esterification by 4-pentenoic anhydride in the presence of DMAP and pyridine. The fourth generation product prepared in a stepwise fashion contains 48 terminal hydroxyl groups [8]
Surface patterning
The thiol-ene functionalization of surface has widespread applications in material science and biotechnology. The attachment of a molecule with a sterically accessible alkene or thiol group to a solid surface enables the construction of polymers on the surface through subsequent thiol-ene reactions.[1] Given that in aqueous solutions thiol-ene reactions can be initiated by UV light (λ = 365–405 nm), the attachment of a given functional group to the exposed thiol or alkene can be controlled spatially through photomasking.[12] More specifically, a photomask, enables the selective exposure of a surface to a UV light source, controlling the location of a given thiol-ene reaction, whereas the identity of the attached molecule is determined by the composition of the aqueous phase placed above the surface at the time of UV exposure. Thus, the manipulation of the shape of the photomask and the composition of the aqueous layer results in the creation of heterogeneous surface, whose properties depend on identity of the attached molecule at a given location.[1]
Jonkheijm et al. (2008) demonstrates that thiol-ene functionalization of a surface can be achieved with a high level of spatial specificity. They covalently attached polyamidoamine (PAMAM) dendrimers with aminocaproic acid spacers to a silicon oxide surface. Thiol-ene functionalization was achieved by exposing the surface to disulfide. Then a photomask was applied to the surface and a thiol-ene addition between the exposed thiol group and a biotin derivative was initiated, using a laser. The effectiveness of the photomask was assessed using Cy5-labeled streptavidin (SAV), a fluorescent maker, which binds to the attached biotin derivative. Fluorescent bands on the silicon surface corresponded to the path of the laser, ranging in width from 20 to 100 µm. The narrow width of the bands indicates that the photomask was effective, enabling highly site-specific surface manipulation.[12]
See also
References
- 1 2 3 4 Lowe, A. B. Polymer Chemistry 2010, 1 (1), 17–36. DOI: 10.1039/B9PY00216B
- 1 2 Nilsson, C.; Simpson, N.; Malkoch, M.; Johansson, M.; Malmstrom, E. Journal of Polymer Science: Part A: Polymer Chemistry 2008, 46, 1339-1348. DOI: 1002/pola.22474
- 1 2 3 4 5 6 Hoyle, C. E.; Bowman, C. N. Agewandte Chemie International Edition 2010, 49 (9), 1540–1573. DOI: 10.1002/anie.200903924
- 1 2 Northrop, B.H.; Coffey, R.N. Journal of American Chemical Society 2012, 134 (33), 13804-13817. DOI: 10.1021/ja305441d
- ↑ Nair, D. P.; Podgórski, M.; Chatani, S.; Gong, T.; Xi, W.; Fenoli, C. R.; Bowman, C. N. Chemistry of Material 2014, 26 (1), 724–744. DOI: 10.1021/cm402180t
- ↑ Scanlan, E. M.; Corcé, V.; Malone, A. Molecules 2014 , 19 (11), 19137–19151. DOI: 10.3390/molecules191119137
- ↑ Walling, C.; Helmreich, W. Journal of American Chemical Society 1959 , 81 (5), 1144–1148. DOI: 10.1021/ja01514a032
- 1 2 3 Kato, K.L.; Campos, L.M.; Hawker, C.J.; Journal of American Chemical Society 2008, 130, 5062-5064. DOI: 10.1021/ja8006325
- 1 2 Montañez, M. I.; Campos, L. M.; Antoni, P.; Hed, Y.; Walter, M. V.; Krull, B. T.; Khan, A.; Hult, A.; Hawker, C. J.; Malkoch, M. Macromolecules 2010, 43 (14), 6004–6013. DOI: 10.1021/ma1009935
- ↑ Rissing, C.; Son, D.Y. Organometallics 2009, 28 (11), 3167-3172. DOI: 10.1021/om9001395
- ↑ Son, D.Y.; Rissing, C.; Chen, L.; Anderson, T.E. Polymer Preprints 2010, 51 (2), 730-731. Link
- 1 2 Jonkheijm, P.; Weinrich, D.; Köhn, M.; Engelkamp, H.; Christianen, P. C. M.; Kuhlmann, J.; Maan, J. C.; Nüsse, D.; Schroeder, H.; Wacker, R.; Breinbauer, R.; Niemeyer, C. M.; Waldmann, H. Angewandte Chemie International Edition 2008, 47 (23), 4421–4424. DOI: 10.1002/anie.20080010