Teleost
Teleost Temporal range: Early Triassic–Recent | |
---|---|
![]() | |
Painted by Castelnau, 1856 (top to bottom, left to right): Fistularia tabacaria, Mylossoma duriventre, Mesonauta acora, Brochis splendens, Pseudacanthicus spinosus, Acanthurus coeruleus, Stegastes pictus | |
Scientific classification | |
Kingdom: | Animalia |
Phylum: | Chordata |
Subphylum: | Vertebrata |
Superclass: | Osteichthyes |
Class: | Actinopterygii |
Subclass: | Neopterygii |
Infraclass: | Teleostei |
Subdivisions | |
See text |
The Teleosts are by far the largest infraclass, the Teleostei, in the class Actinopterygii, the ray-finned fishes. The other two infraclasses are the Holostei (bowfins and garfish) and the paraphyletic Chondrostei (sturgeons and reedfish). The name is derived from Greek (teleios, "complete" + osteon, "bone"). This diverse group, which arose in the Triassic period, has over 26,000 members, arranged in about 40 orders and 448 families. Teleosts range from giant oarfish, measuring 25 ft (7.6 m) or more, and ocean sunfish weighing over two tons, to the minute male anglerfish Photocorynus spiniceps, just 0.24 in (6.2 mm) long. As well as torpedo-shaped fish built for speed, teleosts can be flattened vertically or horizontally, be elongated cylinders or take bizarre shapes like anglerfish and seahorses that are hardly recognizable as fish. Ninety-six percent of all fish are teleosts; they dominate the seas from pole to pole and inhabit the ocean depths, estuaries, rivers, lakes and even swamps.
The difference between teleosts and other bony fish lies mainly in their mouthparts; they have a movable maxilla and premaxilla and corresponding modifications in the jaw musculature which make it possible for them to protrude their jaws outwards from the mouth. This is of great advantage, enabling them to grab prey and draw it into the mouth. In more advanced teleosts, the enlarged premaxilla is the main tooth-bearing bone and the maxilla, which is attached to the lower jaw, acts as a lever, pushing and pulling the premaxilla as the mouth is opened and closed. Other bones further back in the mouth serve to grind and swallow food. Another difference is that the upper and lower lobes of the caudal fin are about equal in size. The spine ends at the caudal peduncle, distinguishing this group from other fish in which the spine extends into the upper lobe of the caudal fin.
Teleosts have adopted a range of reproductive strategies. Most use external fertilization, the female lays a batch of eggs, the male fertilizes them and the larvae develop in the water column without any further parental involvement. A fair proportion of teleosts are hermaphrodites, starting life as females and transitioning to males at some stage, with a few species reversing this process. A small percentage of teleosts are viviparous and some provide parental care with typically the male fish guarding a nest and fanning the eggs to keep them well-oxygenated.
Teleosts are economically important to man as is shown by their depiction in art over the centuries. The fishing industry harvests them for food and anglers attempt to capture them for sport. Some species are farmed commercially, and this method of production is likely to be increasingly important in the future. Others are kept in aquaria or used in research, especially in the fields of genetics and developmental biology.
Anatomy

The defining features of the teleosts are mobile premaxilla, elongated ural neural arches at the caudal fin and unpaired basibranchial toothplates.[3] The mobile premaxilla is unattached to the braincase; it plays a role in protruding the mouth and creating a circular opening. This lowers the pressure inside the mouth, sucking the prey inside. The lower jaw and maxilla are then pulled back to close the mouth, and the fish is able to grasp the prey. By contrast, mere closure of the jaws would risks pushing food out of the mouth. In more advanced teleosts, the premaxilla is enlarged and has teeth, while the maxilla is toothless. The maxilla functions to push the both the premaxilla and the lower jaw forward. To open the mouth, an adductor muscle pulls back the top of the maxilla, pushing the lower jaw forward. In addition, the maxilla rotates slightly, which pushes forward a bony process that interlocks with the premaxilla.[4]
The pharyngeal jaws of teleosts are composed of five branchial arches. The first three arches include a single basibranchial surrounded by two hypobranchials, ceratobranchials, epibranchials and pharyngobranchials. The median basibranchial is covered by a toothplate. The fourth arch is composed of pairs of ceratobranchials and epibranchials and possibly some pharyngobranchials and a basibranchial. The base of the lower pharyngeal jaws is formed by the 5th ceratobranchials while the 2nd, 3rd and 4th pharyngobranchials create the base of the upper. In lower teleosts, the pharyngeal jaws consist of well-separated thin parts that attach to the neurocranium, pectoral girdle, and hyoid bar. Their function is limited to merely transporting food and they rely mostly on lower pharyngeal jaw activity. In more advanced teleosts. the jaws are more powerful with left and right ceratobranchials fusing to become one lower jaw. The pharyngobranchials fuse to create two large upper jaws that articulate with the neurocranium. They have also developed a muscle that allows the pharyngeal jaws to have a role in chewing food in addition to transporting it.[5]
.svg.png)
1. operculum 2. lateral line 3. dorsal fin 4. adipose fin
5. caudal peduncle 6. caudal fin, 7. anal fin, 8. photophores
9. pelvic fins, 10. pectoral fins
The caudal fin is homocercal, meaning the upper and lower lobes are about equal in size. The spine ends at the caudal peduncle, distinguishing this group from those in which the spine extends into the upper lobe of the caudal fin, such as most fish from the Paleozoic.[4] The neural arches are elongated and fused to form uroneurals which provide support for this upper lobe.[4] In general, teleosts tend to be quicker and more flexible then more basal bony fishes. Their skeletal structure has evolved towards greater lightness. While teleost bones are well calcified, they are constructed from a scaffolding of struts, rather than the dense cancellous bones of holostean fish. In addition, the lower jaw of the teleost is reduced to just three bones; the dentary, the angular bone and the articular bone.[6]
Diversity
There are over 26,000 species of teleosts, in about 40 orders and 448 families, most living fishes are members of this group,[7] and few groups of vertebrates have undergone such an extensive radiation. These fish are found in almost every aquatic environment and have developed specializations to feed in a variety of ways as carnivores, herbivores, filter feeders and parasites.[8] The longest teleost is the giant oarfish, with fish of 25 ft (7.6 m) and more having been reported,[9] but this is dwarfed by the extinct Leedsichthys, one individual of which has been estimated to have a length of 91 ft (27.6 m).[10] The heaviest teleost is believed to be the ocean sunfish, with a specimen landed in 2003 having an estimated weight of 2.3 long tons (2.3 metric tons),[11] while the smallest fully mature adult is the male anglerfish Photocorynus spiniceps which can measure just 0.24 in (6.2 mm).[9]
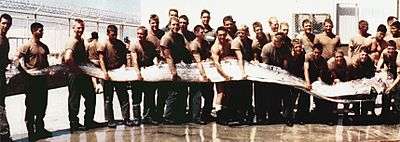
Open water fish are usually streamlined like torpedoes to minimize turbulence as they move through the water. Reef fish live in a complex, relatively confined underwater landscape and for them, manoeuvrability is more important than speed and many such have developed bodies which optimize their ability to dart and change direction. Many have laterally compressed bodies allowing them to fit into fissures and swim through narrow gaps; some use their pectoral fins for locomotion and others undulate their dorsal and anal fins.[12] Some fish have grown dermal appendages for camouflage; the prickly leather-jacket is almost invisible among the seaweed it resembles and the tassled scorpionfish invisibly lurks on the seabed ready to ambush prey. Some like the foureye butterflyfish have eyespots to startle or deceive, while others such as lionfish have aposometic coloring to warn that they are toxic or have venomous spines.[13]

Some teleosts are migratory; certain fresh water species move within river systems on an annual basis; other species are anadromous, spending their lives at sea and moving inland to spawn, salmon and striped bass being examples. Others, exemplified by the eel, are catadromous, doing the reverse.[14] The fresh water European eel (Anguilla anguilla) migrates across the Atlantic Ocean as an adult to breed in floating seaweed in the Sargasso Sea. The adults spawn here then die, but the developing young are swept by the Gulf Stream towards Europe. By the time they arrive, they are small fish and enter estuaries and ascend rivers, overcoming obstacles in their path to reach the streams and ponds where they spend their adult lives.[15]
Some teleosts are parasites. Remoras have their front dorsal fins modified into large suckers with which they cling onto a host animal such as a whale, turtle, shark or ray, but this is probably a commensal rather than parasitic arrangement because both remora and host benefit from the removal of ectoparasites and loose flakes of skin.[16] More harmful are the catfish that enter the gill chambers of fish and feed on their blood and tissues. The cuckoo catfish introduces its eggs into the buccal cavity of a mouth-brooding cichlid and its developing larvae are cared for by their host, consuming their host's young in the process.[17] The snubnosed eel, though usually a scavenger, sometimes bores into the flesh of a fish, and has been found inside the heart of a shortfin mako shark.[18]
Teleosts such as carp (Cyprinidae) developed some unique adaptations. One is the Weberian apparatus, an arrangement of bones (Weberian ossicles) connecting the swim bladder to the inner ear. This enhances their hearing, as sound waves make the bladder vibrate, and the bones transport the vibrations to the inner ear. They also developed a chemical alarm system; when a fish is injured, the warning substance gets in the water, alarming nearby fish.[19]
Some species, such as electric eels, can produce powerful electric currents, strong enough to stun prey. Other fish, such as knifefish, generate weak oscillating electric fields to detect their prey; they swim with straight backs to avoid distorting their electric fields. These currents are produced by modified muscle or nerve cells.[19]
Flatfish are demersal fish that show a greater degree of asymmetry than any other vertebrates. The larvae are at first bilaterally symmetrical but they undergo metamorphosis during the course of their development, with one eye migrating to the other side of the head, and they simultaneously start swimming on their side. This has the advantage that, when they lie on the seabed, both eyes are on top, giving them a broad field of view. The upper side is usually speckled and mottled for camouflage, while the underside is pale.[20]
Evolution and phylogeny

External relationships
The clade Teleostei was first recognized as a distinct group in 1966 by ichthyologists P. Humphry, Donn Eric Rosen and Stanley H. Weitzman.[21] The oldest teleost fossils date back to the late Triassic, evolving from fish related to the bowfins in the clade Holostei. During the Mesozoic and Cenozoic they diversified, and as a result, 96% of all known fish species are teleosts. The cladogram shows the relationship of the teleosts to other bony fish,[22] and to the terrestrial vertebrates (tetrapods) that evolved from a related group of fish.[23][24] The former "Chondrostei" is seen to be paraphyletic. Approximate dates are from Near et al., 2012.[22]
Osteichthyes |
| ||||||||||||||||||||||||||||||||||||||||||||||||||||||
|
Internal relationships
The phylogeny of the teleosts has been subject to long debate, without consensus on either their phylogeny or the timing of the emergence of the major groups before the application of modern DNA-based cladistic analysis. Near et al. (2012) explored the phylogeny and divergence times of every major lineage, analysing the DNA sequences of 9 unlinked genes in 232 species. They obtained well-resolved phylogenies with strongly supported node values. They calibrated the branching times in this tree against 36 reliable measurements of absolute time from the fossil record.[22] The teleosts are divided into the major clades shown on the cladogram, with dates, following Near et al.[22]
Teleostei |
| ||||||||||||||||||||||||||||||||||||||||||||||||||||||||||||||||||||||||||||||||||||||||||||||||||||||||||||||||||||||||||||||||||||||||||
|
Distribution

Teleosts are found world-wide and in most aquatic environments, including warm and cold seas, flowing and still freshwater, even, in the case of the desert pupfish, isolated and sometimes hot and saline bodies of water in deserts.[25][26] Teleost diversity becomes low at extremely high latitudes; at Franz Josef Land, up to 82°N, ice cover and water temperatures below 0 °C (32 °F) for a large part of the year limit the number of species; 75% are endemic to the Arctic.[27]
Of the major groups of teleosts, the Elopomorpha (eels), Clupeomorpha (herrings) and Percomorpha (perches, tunas and many others) all have a worldwide distribution and are mainly marine; the Ostariophysi (carps and catfishes) and Osteoglossomorpha (elephantfishes) are worldwide but mainly freshwater, the latter mainly in the tropics; the Atherinomorpha (guppies, etc.) have a worldwide distribution, both fresh and salt, but are surface-dwellers. In contrast, the Esociformes (pikes) are limited to freshwater in the Northern hemisphere, while the Salmoniformes (salmon, trout) are found in both Northern and Southern temperate zones in freshwater, some species migrating to and from the sea. The Paracanthopterygii (cods, etc.) are Northern hemisphere fish, with both salt and freshwater species.[26]
Teleosts, including the brown trout and the scaly osman, are found in mountain lakes in Kashmir at altitudes as high as 12,530 ft (3,819 m).[28] Teleosts are found at extreme depths in the oceans; the hadal snailfish has been seen at a depth of 25,300 ft (7,700 m), and a related species has been seen at 26,720 ft (8,145 m).[29][30]
Physiology
Respiration

The major means of respiration in teleosts, as in some other fish, is the transfer of gases over the surface of the gills as water is drawn in through the mouth and pumped out through the gills. Apart from the swimbladder, which contains a small amount of air, the body does not have oxygen reserves, and respiration needs to be continuous over the fish's life. Some teleosts exploit habitats where the oxygen availability is low, such as stagnant water or wet mud; they have developed accessory tissues and organs to support gas exchange in these habitats.[31]
Several genera of teleosts have independently developed air-breathing capabilities, and some have become amphibious. Some combtooth blennies emerge to feed on land, and freshwater eels are able to absorb oxygen through damp skin. Mudskippers can remain out of water for considerable periods, exchanging gases through skin and mucous membranes in the mouth and pharynx. Swamp eels have similar well-vascularized mouth-linings, and can remain out of water for days and even aestivate in mud.[32] The anabantoids have developed an accessory breathing structure known as the labyrinth organ on the first gill arch and this is used for respiration in air, and airbreathing catfish have a similar suprabranchial organ. Certain other catfish, such as the Loricariidae, are able to respire through air held in their digestive tracts.[33]
Sensory systems

Teleosts possess highly developed sensory organs. Nearly all daylight fish have color vision at least as good as a human's. Many fish also have chemoreceptors responsible for acute senses of taste and smell. Most fish have sensitive receptors that form the lateral line system, which detects gentle currents and vibrations, and senses the motion of nearby fish and prey.[34] Fish sense sounds in a variety of ways, using the lateral line, the swim bladder and in some species the Weberian organ. Fish orient themselves using landmarks, and may use mental maps based on multiple landmarks or symbols. Experiments with mazes show that fish possess spatial memory and visual discrimination.[35]
Osmoregulation

The skin of a teleost is largely impermeable to water and the main interface between the fish's body and its surroundings is the gills. In freshwater, teleost fish gain water across their gills by osmosis while in seawater, they lose it. Similarly, salts diffuse outwards across the gills in freshwater and inwards in salt water. The European flounder spends most of its life in the sea but often migrates into estuaries and rivers. In the sea it can gain in an hour, Na+ ions equivalent to 40% of its total free sodium content, with 75% of this entering through the gills and the remainder through drinking. By contrast, in rivers there is an exchange of just 2% of the body Na+ content per hour. As well as being able to selectively limit salt and water exchanged by diffusion, there is an active mechanism across the gills for the elimination of salt in sea water and its uptake in fresh water.[36]
Thermoregulation
Fish are cold-blooded and in general, their body temperature is the same as that of their surroundings. They gain and lose heat through their skin and during respiration and are able to regulate their circulation in response to changes in water temperature by increasing or reducing the blood flow to the gills. Metabolic heat generated in the muscles or gut is quickly dissipated through the gills, with blood being diverted away from the gills during exposure to cold.[37] Because of their relative inability to control their blood temperature, most teleosts can only survive in a small range of water temperatures.[38]
Tuna and other fast-swimming ocean-going fish maintain their muscles at higher temperatures than their environment for efficient locomotion.[39] Tuna achieve muscle temperatures 19 °F (−7 °C) or even higher above the surroundings by having a counterflow system in which the metabolic heat produced by the muscles and present in the venous blood, pre-warms the arterial blood before it reaches the muscles. Other adaptations of tuna for speed include a streamlined, spindle-shaped body, fins designed to reduce drag,[39][40] and muscles with a raised myoglobin content, which gives these a reddish color and makes for a more efficient use of oxygen.[41] In polar regions and in the deep ocean, where the temperature is a few degrees above freezing point, some large fish, such as the swordfish, marlin and tuna, have a heating mechanism which raises the temperature of the brain and eye, giving them significantly better vision than their cold-blooded prey.[42]
Buoyancy
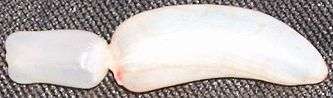
The body of a teleost is denser than water, so fish must compensate for the difference or they will sink. Many teleosts have a swim bladder that adjusts their buoyancy through manipulation of gases to allow them to stay at the current water depth, or ascend or descend without having to waste energy in swimming. In the more primitive groups like some minnows, the swim bladder is open to the esophagus and doubles as a lung. It is often absent in fast swimming fishes such as the tuna and mackerel. In fish where the swim bladder is closed, the gas content is controlled through the rete mirabilis, a network of blood vessels serving as a countercurrent gas exchanger between the swim bladder and the blood.[43] The Chondrostei such as sturgeons also have a swim bladder, but this appears to have evolved separately: other Actinopterygii such as the bowfin and the bichir do not have one, so swim bladders appear to have arisen twice, and the teleost swim bladder is not homologous with the chondrostean one.[44]
Locomotion

A typical teleost fish has a streamlined body for rapid swimming, and locomotion is generally provided by a lateral undulation of the hindmost part of the trunk and the tail, propelling the fish through the water.[45] There are many exceptions to this method of locomotion, especially where speed is not the main objective; among rocks and on coral reefs, slow swimming with great manoeuvrability may be a desirable attribute.[46] Living among seagrasses and algae, the seahorse adopts an upright posture and moves by fluttering its pectoral fins, and the closely related pipefish moves by rippling its elongated dorsal fin. Gobies "hop" along the substrate, propping themselves up and propelling themselves with their pectoral fins. In some species, a pelvic sucker allows them to climb, and the Hawaiian freshwater goby climbs waterfalls while migrating.[47] Gurnards have three pairs of free rays on their pectoral fins which have a sensory function but on which they can walk along the substrate.[48] Flying fish launch themselves into the air and can glide on their enlarged pectoral fins for hundreds of metres.[49] In many fishes of the order Acanthopterygii, locomotion is aided by a spiny dorsal fin, which is in front of the soft-rayed dorsal fin. This fin helps provide thrust.[50]
Reproduction and lifecycle

Most teleost species have external fertilization; both eggs and sperm are released in the open for fertilization. Internal fertilization, which is more typical for Chondrichthyes and many tetrapods, occurs in 500–600 species of teleosts. This involves the male inseminating the female with an intromittent organ.[51] There are two major reproductive strategies of teleosts; semelparity and iteroparity. In the former, an individual breeds once after reaching maturity and then dies. This is because the physiological changes that come with reproduction eventually lead to death.[52] Salmon (Oncorhynchus) are well known for this. These species hatch in fresh water and then travel to sea for up to 4 years before travelling back to their place of birth where they spawn and die. Semelparity is also known to occur in some eels and smelts. The majority of teleost species, however, have iteroparity, where mature individuals can breed multiple times in their life.[53]
Sex identity and determination

The majority (88%) of teleost species are gonochoristic, having individuals that remain either male or female throughout their adult lives. The sex of an individual can be determined genetically as in birds and mammals, or environmentally as in reptiles. In some teleosts, both genetics and the environment play a role in determining sex.[54] For species whose sex is determined by genetics, it can come in three forms. In monofactorial sex determination, a single-locus determines sex inheritance. Both the XY sex-determination system and ZW sex-determination system exist in teleost species. Some species, such as the southern platyfish (Xiphophorus maculatus), have both systems and a male can be determined by XY or ZZ depending on the population.[55]
Multifactorial sex determination occurs in numerous Neotropical species and involves both XY and ZW systems. Multifactorial systems, involve rearrangements of sex chromosomes and autosomes. For example, the darter characine (Apareiodon affinis) has a ZW multifactorial system where the female is determined by ZW1W2 and the male by ZZ. The wolf fish (Hoplias malabaricus) has a XY multifactorial system where females are determined by X1X1X2X2 and the male by X1X2Y.[56] Some teleosts, such as zebrafish (Danio rerio), have a polyfactorial system, where there are several genes which play a role in determining sex.[57] Environment-dependent sex determination has been documented in at least 70 species of teleost. temperature is the main factor, but PH levels, growth rate, density and social environment may also play a role. For the Atlantic silverside (Menidia menidia), spawning in colder waters creates more females, while warmer waters create more males.[58]
Hermaphroditism
Some teleost species are hermaphroditic, which can come in two forms: simultaneous and sequential. In the former, both spermatozoa and egg gamete are present in the gonads. Simultaneous hermaphroditism typically occurs in species that live in the ocean deeps, where potenial mates are sparsely dispersed.[59][60] Self-fertilization is rare and has only been recorded in two species the Kryptolebias marmoratus and Kryptolebias hermaphroditus.[60] With sequential hermaphroditism, individuals may function as one sex early in their adult life and switch later in life. Species with this condition include parrotfish, wrasses, sea basses, flatheads, sea breams and lightfishes.[59]
Protandry is when an individual starts out male and becomes female while the reverse is protogyny, the latter is more common. Changing sex can occur in various contexts. In the bluestreak cleaner wrasse (Labroides dimidiatus), where males have harems of up to ten females, if the male is removed the largest and most dominant female will develop male-like behavior and eventually testes. If she is removed, the next ranking female takes her place. In the species Anthias squamipinnis, where individuals gather into large groups and females greatly outnumber males, if a certain number of males are removed from a group, the same number of females will change sex and replace them. In clownfish, individuals live in groups and only the two largest in a group breed; the largest female and the largest male. If the female dies, the male will switch sexes and the next largest male will take his place.[61]
Mating tactics
_courting_a_female_1471-2148-11-233-1.jpeg)
There are several different mating system among teleosts. Some species are promiscuous, where both males and females breed with multiple partners and there are no obvious mate choices. This has been recorded in Baltic herring, guppies, Nassau groupers, humbug damselfish (Dascyllus melanurus), cichlids and creole wrasses. Polygamy, where one sex has multiple partners can come in many forms. Polyandry consists of one adult female breeding with multiple males, who only breed with that female. This is rare among teleosts, and fish in general, but is found in the clownfish. In addition, it may also exist to an extent among anglerfish, where some females have more than one male attached to them. Polygyny, where one male breeds with multiple females, is much more common. This is recorded in sculpins, sunfish, darters, damselfish and cichlids where multiple females may visit a territorial male that will guard and take care of eggs and young. Polygyny may also involve a male guarding a harem of several females. This occurs in coral reef species, such as damselfishes, wrasses, parrotfishes, surgeonfishes, triggerfishes and tilefishes.[53]
Lek breeding, where males congregate to display to females, has been recorded in at least one species Cyrtocara eucinostomus. Lek-like breeding system have also been recorded in several other species. In monogamous species, males and female may form pair bonds and breed exclusively with their partners. This occurs in North American freshwater catfishes, many butterflyfishes, sea horses and several other species.[53] Courtship in teleosts plays a role in species recoginition, strengthening pair bonds, spawning site position and gamete release synchronization. This includes color changes, sound production and visual displays (fin erection, rapid swimming, breaching), which is often done by the male. Courtship may be done by a female to overcome a territorial male that would otherwise drive her away.[62]
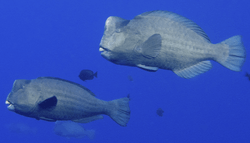
Sexual dimorphism exists in some species. Individuals of one sex, usually males develop secondary sexual characteristics that increase their chances of reproductive success. In dolphinfish (Coryphaena), males have larger and blunter heads than females. In several minnow species, males develop swollen heads and small bumps known as breeding tubercles during the breeding season.[63] The male green humphead parrotfish (Bolbometopon muricatum) has a more well-developed forehead with an "ossified ridge" which plays a role in ritualized headbutting.[64] Dimorphism can also take the form of coloration differences. Again, it is usually the males that are brightly colored; in killifishes, rainbowfishes and wrasses the colors are permanent while in species like minnows, sticklebacks, darters and sunfishes, the color changes with seasons. Such coloration can be very conspicuous to predators, showing that the drive to reproduce can be stronger than predator avoidance.[63]
Males have been unable to successfully court a female may try to achieve reproductive success in other ways. In sunfish species like the bluegill (Lepomis macrochirus) where larger, older males known as parental males which have successfully courted a female and construct nests for the eggs they fertilize, smaller satellite males will mimic female behavior and coloration to access a nest so they can fertilize the eggs. Other males, known as sneaker males, lurk nearby and then quickly dash to the nest and fertilize on the run. These males are smaller than satellite males. Sneaker males also exist in Oncorhynchus salmon, where small males that were unable to establish a position near a female will dash in while the large dominant male is spawning with the female.[65]
Spawning sites and parental care

Teleosts may spawn in the water column or, more commonly, on the substrate. Water column spawners are mostly limited to coral reefs; the fish will rush towards the surface and release their gametes. This appears to protect the eggs from some predators and allow then to disperse widely via currents. However, they receive no parental care. Water column spawners are more likely than substrate spawners to spawn in groups. Substrate spawning commonly occurs in nests, rock crevices or even burrows. Some eggs can stick to various surfaces like rocks, plants, wood or shells.[66]
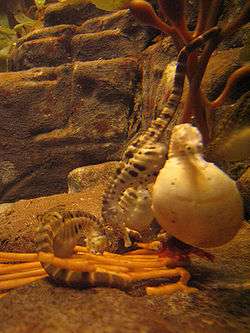
Of the oviparous teleosts, most (79%) do not provide parental care.[67] Male care is far more common than female care.[67][68] Male territoriality "preadapts" a species to evolve male parental care.[69][70] One unusual example of female parental care is in mother discuses (Symphysodon), which provide nutrients for their developing young in the form of mucus.[71] Some teleost species have their eggs or young attached to or carried in their bodies. For sea catfishes, cardinalfishes, jawfishes and some others, the egg may be incubated or carried in the mouth, a practice know as mouthbrooding. In some African cichlids, the eggs may be fertilized there. In species like the banded acara (Bujurquina vittata), young are brooded after they hatch and this may be done by both parents. The timing of the release of young varies between species; some mouthbrooders release new-hatched young while other may keep then until they are juveniles. In addition to mouthbrooding, some teleost have also developed structures to carry young. Male nurseryfish have a bony hook on their foreheads to carry fertilized eggs; they remain on the hook until they hatch. For seahorses, the male has a brooding pouch where the female deposits the fertilized eggs and they remain there until they become free-swimming juveniles. Female banjo catfishes have structures on their belly which the eggs attach to.[72]
In some parenting species, young from a previous spawning batch may stay with their parents and help care for the new young. This is known to occur in around 19 species of cichlids in Lake Tanganyika. These helpers take part in cleaning and fanning eggs and larvae, cleaning the breeding hole and protecting the territory. They have reduced growth rate but gain protection from predators. Brood parasitism also exists among teleosts; minnows may spawn in sunfish nests as well as nests of other minnow species. The cuckoo catfish (Synodontis multipunctatus) is known for laying eggs on the substrate as mouthbrooding cichcilds collect theirs and the young catfish will eat the larvae. Filial cannibalism occurs in some teleost families and may have evolved to combat starvation.[73]
Growth and development

Teleosts have four major life stages: the egg, the larva, the juvenile and the adult. Species may begin life in a pelagic environment or a demersal environment. Most marine teleosts have pelagic eggs, which are light, transparent and buoyant with thin envelopes. Pelagic eggs rely on the ocean currents to disperse and receive no parental care. When they hatch, the larvae are planktonic and unable to swim. They have a yolk sac attached to them which provides nutrients. Most freshwater species produce demersal eggs which are thick, pigmented, relatively heavy and able to stick to substrates. Parental care is much more common among freshwater fish. Unlike their pelagic counterparts, demersal larvae are able to swim and feed as soon as they hatch.[59] Larval teleosts often look very different from adults, particularly in marine species. Some larvae were even considered different species from the adults. Larvae have high mortality rates, most die from starvation or predation within their first week. As they grow, survival rates increase and there is greater physiological tolerance and sensitivity, ecological and behavioral competence.[74]
At the juvenile stage, a teleost looks more like its adult form. At this stage, its axial skeleton, internal organs, scales, pigmentation and fins are fully developed. The transition from larvae to juvenile can be short and fairly simple, lasting minutes or hours as in some damselfish, while in other species, like salmon, squirrelfish, gobies and flatfishes, the transition is more complex and takes several weeks to complete.[75] At the adult stage, a teleost is able to produce viable gametes for reproduction. Like many fish, teleosts continue to grow throughout their lives. Longevity depends on the species with some gamefish like European perch (Perca fluviatilis) and largemouth bass (Micropterus salmoides) living up to 25 years. Rockfish appear to be the longest living teleosts with some species living over 100 years.[76]
Shoaling and schooling

Many teleosts form shoals, which serve multiple purposes in different species. Schooling is sometimes an antipredator adaptation, offering improved vigilance against predators. It is often more efficient to gather food by working as a group, and individual fish optimize their strategies by choosing to join or leave a shoal. When a predator has been noticed, prey fish respond defensively, resulting in collective shoal behaviors such as synchronized movements. Responses do not consist only of attempting to hide or flee; antipredator tactics include for example scattering and reassembling. Fish also aggregate in shoals to spawn.[77]
Importance to humans

Teleost fishes are economically important in different ways. They are captured for food around the world. A small number of species such as herring, cod, pollock, anchovy, tuna and mackerel provide people with millions of tons of food per year, while many other species are fished in smaller amounts.[78] They provide a large proportion of the fish caught for sport.[79] Commercial and recreational fishing together provide millions of people with employment.[80]
A small number of productive species including carp, salmon,[81] tilapia and catfish are farmed commercially, producing millions of tons of protein-rich food per year. Production is expected to increase sharply so that by 2030, perhaps 62% of food fish will be farmed.[82]

Some smaller and more colorful species serve as aquarium specimens and pets. Sea wolves are used in the leather industry. Isinglass is made from thread fish and drum fish.[79]
A few teleosts are dangerous. Some like the electric eel and the electric catfish can give a severe electric shock. Others such as the piranha and barracuda have a powerful bite and have sometimes attacked human bathers.[79]
Medaka and zebrafish are used as research models for studies in genetics and developmental biology. The zebrafish is the most commonly used laboratory vertebrate,[79] offering the advantages of similar genetics to mammals, small size, simple environmental needs, transparent larvae permitting non-invasive imaging, plentiful offspring, rapid growth, and the ability to absorb mutagens added to their water.[83]
In art
Teleost fishes have been frequent subjects in art, reflecting their economic importance, for at least 14,000 years. Fish patterns were common in Ancient Egypt, acquiring mythological significance in Ancient Greece and Rome, and from there into Christianity as a religious symbol. Fishes became common in Renaissance art, with still life paintings reaching a peak of popularity in the Netherlands in the 17th century. Fish imagery however remained frequent into the 20th century, different artists such as Klee, Magritte, Matisse and Picasso expressing radically different themes from attractive to violent. In contrast, artists in China and Japan use fish images symbolically.[84]
-
Wall painting of fishing, Tomb of Menna the scribe, Thebes, Ancient Egypt, c. 1422–1411 BC
-
Italian Renaissance: Fish, Antonio Tanari, c. 1610–1630, in the Medici Villa, Poggio a Caiano
-
Dutch Golden Age painting: Fish Still Life with Stormy Seas, Willem Ormea and Abraham Willaerts, 1636
-
Mandarin Fish by Bian Shoumin, Qing Dynasty, 18th century
-
"Teleostei" by Ernst Haeckel, 1904. Four species, surrounded by scales
-
Fish Magic, Paul Klee, oil and watercolor varnished, 1925
Notes
- ↑ Thus the former "Chondrostei" is not a clade, but is broken up.
References
- ↑ Palmer, Douglas (1999). The Marshall Illustrated Encyclopedia of Dinosaurs & Prehistoric animals. Marshall Editions Developments. ISBN 978-1840281521.
- ↑ "The Paleobiology Database". The Paleobiology Database. 14 June 2013.
- ↑ Patterson, C.; Rosen, D. E. (1977). "Review of ichthyodectiform and other Mesozoic teleost fishes, and the theory and practice of classifying fossils". Bulletin of the AMNH 158 (2): 81–172.
- 1 2 3 Benton, Michael (2005). "The Evolution of Fishes After the Devonian". Vertebrate Palaeontology (3rd ed.). John Wiley & Sons. p. 175. ISBN 978-1-4051-4449-0.
- ↑ Vandewalle, P.; Parmentier, E.; Chardon, M. (2000). "The branchial basket in Teleost feeding" (PDF). Cybium 24 (4): 319–42. ISSN 0399-0974.
- ↑ Bone, Q.; Moore, R. (2008). Biology of Fishes. Garland Science. p. 29. ISBN 978-0-415-37562-7.
- ↑ Miller, Stephen; Harley, John P. (2007). Zoology (7th ed.). McGraw-Hill. p. 297.
- ↑ Dorit, R. L.; Walker, W. F.; Barnes, R. D. (1991). Zoology. Saunders College Publishing. pp. 67–69. ISBN 978-0-03-030504-7.
- 1 2 Guinness World Records 2015. Guinness World Records. 2014. p. 60. ISBN 978-1-908843-70-8.
- ↑ Martill, D.M. (1988). "Leedsichthys problematicus, a giant filter-feeding teleost from the Jurassic of England and France". Neues Jahrbuch fur Geologie und Palaontologie 11: 670–680.
- ↑ Roach, John (13 May 2003). "World's Heaviest Bony Fish Discovered?". National Geographic News. Retrieved 9 January 2016.
- ↑ Maddock, L.; Bone, Q.; Rayner, J.M.V. (1994). The Mechanics and Physiology of Animal Swimming. Cambridge University Press. pp. 54–56. ISBN 978-0-521-46078-1.
- ↑ Ross, David A. (2000). The Fisherman's Ocean. Stackpole Books. pp. 136–138. ISBN 978-0-8117-2771-6.
- ↑ "What is an anadromous fish? A catadromous fish?". Fish FAQ. NOAA. Retrieved 12 January 2016.
- ↑ "Anguilla anguilla (Linnaeus, 1758)". Cultured Aquatic Species Information Programme. FAO: Fisheries and Aquaculture Department. 1 January 2004. Retrieved 2 January 2016.
- ↑ Jackson, John (30 November 2012). "How does the Remora develop its sucker?". National History Museum. Retrieved 2 January 2016.
- ↑ Combes, Claude (2001). Parasitism: The Ecology and Evolution of Intimate Interactions. University of Chicago Press. p. 23. ISBN 978-0-226-11446-0.
- ↑ Caira, J.N.; Benz, G.W.; Borucinska, J.; Kohler, N.E. (1997). "Pugnose eels, Simenchelys parasiticus (Synaphobranchidae) from the heart of a shortfin mako, Isurus oxyrinchus (Lamnidae)". Environmental Biology of Fishes 49: 139–144. doi:10.1023/a:1007398609346.
- 1 2 Helfman, Collete, Facey and Bowen pp. 268–74
- ↑ Schreiber, Alexander M. (2006). "Asymmetric craniofacial remodeling and lateralized behavior in larval flatfish". Journal of Experimental Biology 209: 610–621. doi:10.1242/jeb.02056.
- ↑ Helfman, Collete, Facey and Bowen p. 7
- 1 2 3 4 Thomas J. Near; et al. (2012). "Resolution of ray-finned fish phylogeny and timing of diversification". PNAS. pp. 13698–13703. doi:10.1073/pnas.1206625109.
- ↑ Betancur-R, Ricardo; et al. (2013). "The Tree of Life and a New Classification of Bony Fishes". PLOS Currents Tree of Life (1st ed.). doi:10.1371/currents.tol.53ba26640df0ccaee75bb165c8c26288.
- ↑ Laurin, M.; Reisz, R.R. (1995). "A reevaluation of early amniote phylogeny". Zoological Journal of the Linnean Society 113: 165–223.
- ↑ Dudek and ICF International (2012). Desert Renewable Energy Conservation Plan (DRECP) Baseline Biology Report. California Energy Commission.
- 1 2 "Actinopterygii - ray-finned fishes". University College, London.
- ↑ Chernova, N. V.; Friedlander, A. M.; Turchik, A.; Sala, E. (2014). "Franz Josef Land: extreme northern outpost for Arctic fishes". PeerJ 2: e692. doi:10.7717/peerj.692. PMID 25538869.
- ↑ Raina, H. S.; Petr, T. "Coldwater Fish and Fisheries in The Indian Himalayas: Lakes and Reservoirs". Food and Agriculture Organization. Retrieved 6 January 2016.
- ↑ Morelle, Rebecca (7 October 2008). "'Deepest ever' living fish filmed". BBC News. Retrieved 5 February 2016.
- ↑ Morelle, Rebecca (19 December 2014). "New record for deepest fish". BBC News. Retrieved 5 February 2016.
- ↑ Meurant, Gerard (1984). Fish Physiology V10A. Academic Press. pp. 263–. ISBN 978-0-08-058531-4.
- ↑ Liem, Karel F. (1998). Paxton, J.R.; Eschmeyer, W.N., ed. Encyclopedia of Fishes. Academic Press. pp. 173–174. ISBN 0-12-547665-5.
- ↑ Armbruster, Jonathan W. (1998). "Modifications of the digestive tract for holding air in loricariid and scoloplacid catfishes" (PDF). Copeia (3): 663–675. doi:10.2307/1447796.
- ↑ Orr, James (1999). Fish. Microsoft Encarta 99. ISBN 0-8114-2346-8.
- ↑ Journal of Undergraduate Life Sciences. "Appropriate maze methodology to study learning in fish" (PDF). Retrieved 28 May 2009.
- ↑ Bentley, P.J. (2013). Endocrines and Osmoregulation: A Comparative Account in Vertebrates. Springer. p. 26. ISBN 978-3-662-05014-9.
- ↑ Whittow, G. Causey (2013). Comparative Physiology of Thermoregulation: Special Aspects of Thermoregulation. Academic Press. p. 223. ISBN 978-1-4832-5743-3.
- ↑ McFarlane, Paul (1 January 1999). "Warm-blooded fish". Monthly Bulletin. Hamilton and District Aquarium Society. Retrieved 6 January 2016.
- 1 2 Martin, R. Aidan (April 1992). "Fire in the Belly of the Beast". ReefQuest Centre for Shark Research. Retrieved 6 January 2016.
- ↑ "Tuna: biology of tuna". Free Encyclopedia. Retrieved 2 January 2016.
- ↑ Brown, W. Duane (1962). "The concentration of myoglobin and hemoglobin in tuna flesh". Journal of Food Science 27 (1): 26–28. doi:10.1111/j.1365-2621.1962.tb00052.x.
- ↑ Fritsches, Kerstin (11 January 2005). "Warm eyes give deep-sea predators super vision". University of Queensland. Retrieved 6 January 2016.
- ↑ Kardong, K. (2008). Vertebrates: Comparative anatomy, function, evolution (5th ed.). Boston: McGraw-Hill. ISBN 0-07-304058-4.
- ↑ Fernandes, Marisa N. (8 January 2007). Fish Respiration and Environment. CRC Press. p. 42. ISBN 978-1-4398-4254-6.
- ↑ Numerical Studies of Hydrodynamics of Fish Locomotion and Schooling by a Vortex Particle Method. ProQuest. 2008. pp. 1–4. ISBN 978-1-109-14490-1.
- ↑ Kapoor, B.G.; Khanna, Bhavna (2004). Ichthyology Handbook. Springer. pp. 149–151. ISBN 978-3-540-42854-1.
- ↑ Patzner, Robert; Van Tassell, James L.; Kovacic, Marcelo; Kapoor, B.G. (2011). The Biology of Gobies. CRC Press. pp. 261, 507. ISBN 978-1-4398-6233-9.
- ↑ Jamon, M; Renous, S; Gasc, J.P.; Bels, V; Davenport, J. (2007). "Evidence of force exchanges during the six-legged walking of the bottom-dwelling fish, Chelidonichthys lucerna". Journal of Experimental Zoology 307 (9): 542–547. doi:10.1002/jez.401. PMID 17620306.
- ↑ Dasilao, J.C.; Sasaki, K. (1998). "Phylogeny of the flyingfish family Exocoetidae (Teleostei, Beloniformes)". Ichthyological Research 45 (4): 347–353. doi:10.1007/BF02725187.
- ↑ Drucker, E. G.; Lauder, G. V. (2001). "Locomotor function of the dorsal fin in teleost fishes: experimental analysis of wake forces in sunfish". Journal of Experimental Biology 204: 2943–58.
- ↑ Wootton and Smith p. 56.
- ↑ Wootton and Smith p. 55.
- 1 2 3 Helfman, Collete, Facey and Bowen p. 457
- ↑ Wootton and Smith p. 53.
- ↑ Wootton and Smith p. 71–80.
- ↑ Wootton and Smith p. 81–82.
- ↑ Wootton and Smith p. 82–83.
- ↑ Wootton and Smith p. 83–85.
- 1 2 3 Laying, E. "Fish Reproduction" (PDF). Retrieved 2016-01-07.
- 1 2 Wootton and Smith p. 54.
- ↑ Helfman, Collete, Facey and Bowen p. 458
- ↑ Helfman, Collete, Facey and Bowen p. 465
- 1 2 Helfman, Collete, Facey and Bowen p. 463
- ↑ Muñoz, R.; Zgliczynski, B.; Laughlin, J.; Teer, B. (2012). "Extraordinary Aggressive Behavior from the Giant Coral Reef Fish, Bolbometopon muricatum, in a Remote Marine Reserve". PLOS ONE 7 (6): e38120. doi:10.1371/journal.pone.0038120.
- ↑ Helfman, Collete, Facey and Bowen p. 473
- ↑ Helfman, Collete, Facey and Bowen p. 465–68
- 1 2 Reynolds, John; Nicholas B. Goodwin; Robert P. Freckleton (19 March 2002). "Evolutionary Transitions in Parental Care and Live Bearing in Vertebrates". Philosophical Transactions of the Royal Society B: Biological Sciences 357 (1419). doi:10.1098/rstb.2001.0930. PMC 1692951. PMID 11958696.
- ↑ Clutton-Brock, T. H. (1991). The Evolution of Parental Care. Princeton, NJ: Princeton University Press.
- ↑ Werren, John; Mart R. Gross; Richard Shine (1980). "Paternity and the evolution of male parentage". Journal of Theoretical Biology 82 (4). doi:10.1016/0022-5193(80)90182-4. Retrieved 15 September 2013.
- ↑ Baylis, Jeffrey (1981). "The Evolution of Parental Care in Fishes, with reference to Darwin's rule of male sexual selection". Environmental Biology of Fishes 6 (2). doi:10.1007/BF00002788.
- ↑ Wootton and Smith p. 520
- ↑ Wootton and Smith pp. 57, 523–30
- ↑ Helfman, Collete, Facey and Bowen pp. 472–73
- ↑ Helfman, Collete, Facey and Bowen pp. 146–47
- ↑ Helfman, Collete, Facey and Bowen pp. 149
- ↑ Helfman, Collete, Facey and Bowen pp. 153–56
- ↑ Pitcher, Tony J. (1986). 12. Functions of Shoaling Behaviour in Teleosts. The Behaviour of Teleost Fishes (Springer). pp. 294–337.
- ↑ "Capture production by principal species in 2012" (PDF). Food and Agriculture Organization. Retrieved 10 February 2016.
- 1 2 3 4 Kisia, S. M. (2010). Vertebrates: Structures and Functions. CRC Press. p. 22. ISBN 978-1-4398-4052-8.
- ↑ "New Economic Report Finds Commercial and Recreational Saltwater Fishing Generated More Than Two Million Jobs". National Oceanic and Atmospheric Administration. Retrieved 10 February 2016.
- ↑ Scottish Fish Farm Production Survey 2014. The Scottish Government/Riaghaltas na h-Alba. September 2015. ISBN 978-1-78544-608-5.
- ↑ "Fish to 2030 : prospects for fisheries and aquaculture (Report 83177)". Food and Agriculture Organization; World Bank Group. 1 December 2013. Retrieved 3 January 2016.
- ↑ "Five reasons why zebrafish make excellent research models". NC3RS. 10 April 2014. Retrieved 15 February 2016.
- ↑ Moyle, Peter B.; Moyle, Marilyn A. (May 1991). "Introduction to fish imagery in art". Environmental Biology of Fishes 31 (1): 5–23.
Bibliography
- Helfman, G.; Collette, B. B.; Facey, D. E.; Bowen, B. W. (2009). The Diversity of Fishes: Biology, Evolution, and Ecology (PDF) (2nd ed.). Wiley-Blackwell. ISBN 978-1-4051-2494-2.
- Wootton, Robert J.; Smith, Carl (2014). Reproductive Biology of Teleost Fishes. Wiley. ISBN 978-1-118-89139-1.
|