1815 eruption of Mount Tambora
1815 Eruption of Mount Tambora | |
---|---|
False color image of Mount Tambora, taken from the Space Shuttle Endeavour on 13 May 1992 (for orientation, the top of the image is towards the East). | |
Volcano | Mount Tambora |
Date | 1815 |
Type | Ultra Plinian |
Location |
Sumbawa, Lesser Sunda Islands, Dutch East Indies 8°15′S 118°00′E / 8.25°S 118°ECoordinates: 8°15′S 118°00′E / 8.25°S 118°E |
VEI | 7 |
Impact | Reduced global temperatures, leading the following year, 1816, to be called the Year Without a Summer. |
The 1815 Eruption of Mount Tambora was one of the most powerful eruptions in recorded history and is classified as a VEI-7 event. The eruption of the volcano, on the island of Sumbawa in Indonesia, reached a climax on 10 April 1815[1] and was followed by between six months and three years of increased steaming and small phreatic eruptions.
The eruption column lowered global temperatures, and some experts believe this led to global cooling and worldwide harvest failures, sometimes known as the Year Without a Summer.[2] The eruption resulted in a brief period of significant climate change that led to various cases of extreme weather. Several climate forcings coincided and interacted in a systematic manner that has not been observed since, despite other large eruptions that have occurred since the early Stone Age. Although the link between the post-eruption climate changes and the Tambora event has been established by various scientists, the understanding of the processes involved is incomplete.[3]
Chronology of the eruption

Mount Tambora experienced several centuries of dormancy before 1815, as the result of the gradual cooling of hydrous magma in a closed magma chamber.[4] Inside the chamber at depths between 1.5 and 4.5 km (0.93 and 2.80 mi), the exsolution of a high-pressure fluid magma formed during cooling and crystallisation of the magma. Overpressure of the chamber of about 4,000–5,000 bar (400–500 MPa; 58,000–73,000 psi) was generated, and the temperature ranged from 700 to 850 °C (1,300–1,600 °F).[4] In 1812, the caldera began to rumble and generated a dark cloud.[5]
On 5 April 1815, a huge eruption occurred, followed by thunderous detonation sounds, heard in Makassar on Sulawesi, 380 km (240 mi) away, Batavia (now Jakarta) on Java 1,260 km (780 mi) away, and Ternate on the Molucca Islands 1,400 km (870 mi) away. On the morning of 6 April, volcanic ash began to fall in East Java with faint detonation sounds lasting until 10 April. What was first thought to be sound of firing guns was heard on 10 April on Sumatra more than 2,600 km (1,600 mi) away.[6]
At about 7 pm on 10 April, the eruptions intensified.[5] Three columns of flame rose up and merged.[6] The whole mountain was turned into a flowing mass of "liquid fire".[6] Pumice stones of up to 20 cm (7.9 in) in diameter started to rain down around 8 pm, followed by ash at around 9–10 pm. Pyroclastic flows cascaded down the mountain to the sea on all sides of the peninsula, wiping out the village of Tambora. Loud explosions were heard until the next evening, 11 April. The ash veil had spread as far as West Java and South Sulawesi. A "nitrous" odour was noticeable in Batavia and heavy tephra-tinged rain fell, finally receding between 11 and 17 April.[5]
The first explosions were heard on this Island in the evening of 5 April, they were noticed in every quarter, and continued at intervals until the following day. The noise was, in the first instance, almost universally attributed to distant cannon; so much so, that a detachment of troops were marched from Djocjocarta, in the belief that a neighbouring post was being attacked, and along the coast boats were in two instances dispatched in quest of a supposed ship in distress.—Sir Stamford Raffles' memoir.[6]
The explosion is estimated to have been a VEI-7.[7] An estimated 41 km3 (9.8 cu mi) of pyroclastic trachyandesite were ejected, weighing about 10000 million tonnes. This has left a caldera measuring 6–7 km (3.7–4.3 mi) across and 600–700 m (2,000–2,300 ft) deep.[5] The density of fallen ash in Makassar was 636 kg/m³.[8] Before the explosion, Mount Tambora was about 4,300 m (14,100 ft) high,[5] one of the tallest peaks in the Indonesian archipelago. After the explosion, it measured only 2,851 m (9,354 ft) (about two thirds of its previous height).[9]
The 1815 Tambora eruption is the largest observed eruption in recorded history (see Table I, for comparison).[5][10] The explosion was heard 2,600 km (1,600 mi) away, and ash fell at least 1,300 km (810 mi) away.[5] Pitch darkness was observed as far away as 600 km (370 mi) from the mountain summit for up to two days. Pyroclastic flows spread at least 20 km (12 mi) from the summit. Due to the eruption, Indonesia's islands were struck by tsunami waves reaching heights up to 4 m (13 ft).
Aftermath
On my trip towards the western part of the island, I passed through nearly the whole of Dompo and a considerable part of Bima. The extreme misery to which the inhabitants have been reduced is shocking to behold. There were still on the road side the remains of several corpses, and the marks of where many others had been interred: the villages almost entirely deserted and the houses fallen down, the surviving inhabitants having dispersed in search of food.
...
Since the eruption, a violent diarrhoea has prevailed in Bima, Dompo, and Sang'ir, which has carried off a great number of people. It is supposed by the natives to have been caused by drinking water which has been impregnated with ashes; and horses have also died, in great numbers, from a similar complaint.—Lt. Philips, ordered by Sir Stamford Raffles to go to Sumbawa.[6]
All vegetation on the island was destroyed. Uprooted trees, mixed with pumice ash, washed into the sea and formed rafts up to 5 km (3.1 mi) across.[5] One pumice raft was found in the Indian Ocean, near Calcutta on 1 and 3 October 1815.[10] Clouds of thick ash still covered the summit on 23 April. Explosions ceased on 15 July, although smoke emissions were still observed as late as 23 August. Flames and rumbling aftershocks were reported in August 1819, four years after the event.
A moderate-sized tsunami struck the shores of various islands in the Indonesian archipelago on 10 April, with a height of up to 4 m (13 ft) in Sanggar around 10 pm.[5] A tsunami of 1–2 m (3.3–6.6 ft) in height was reported in Besuki, East Java, before midnight, and one of 2 metres (6.6 ft) in height in the Molucca Islands. The total death toll has been estimated to be around 4,600.[11]
The eruption column reached the stratosphere, an altitude of more than 43 km (27 mi).[10] The coarser ash particles fell one to two weeks after the eruptions, but the finer ash particles stayed in the atmosphere from a few months up to a few years at altitudes of 10–30 km (6.2–18.6 mi).[5] Longitudinal winds spread these fine particles around the globe, creating optical phenomena. Prolonged and brilliantly coloured sunsets and twilights were frequently seen in London between 28 June and 2 July 1815 and 3 September and 7 October 1815.[5] The glow of the twilight sky typically appeared orange or red near the horizon and purple or pink above.
The estimated number of deaths varies depending on the source. Zollinger (1855) puts the number of direct deaths at 10,000, probably caused by pyroclastic flows. On Sumbawa island, 38,000 deaths were due to starvation, and another 10,000 deaths occurred due to disease and hunger on Lombok island.[12] Petroeschevsky (1949) estimated about 48,000 and 44,000 people were killed on Sumbawa and Lombok, respectively.[13] Several authors use Petroeschevsky's figures, such as Stothers (1984), who cites 88,000 deaths in total.[5] However, Tanguy et al.. (1998) claimed Petroeschevsky's figures to be unfounded and based on untraceable references.[14] Tanguy revised the number solely based on two credible sources, q.e., Zollinger, who himself spent several months on Sumbawa after the eruption, and Raffles's notes.[6] Tanguy pointed out that there may have been additional victims on Bali and East Java because of famine and disease. Their estimate was 11,000 deaths from direct volcanic effects and 49,000 by posteruption famine and epidemic diseases.[14] Oppenheimer (2003) stated a modified number of at least 71,000 deaths in total.[10] Reid takes note of the total direct and indirect deaths caused beyond Sumbawa, in Bali and elsewhere, and suggests that a figure of perhaps 100,000 deaths is an appropriate estimate.[15]
Disruption of global temperatures
The conditions during the northern hemisphere summer of 1816 were the result of the largest observed eruption in recorded human history, one during which global temperatures decreased by an average of 0.53 °C, and related human deaths were reported to be about 90,000. The importance of volcanic eruptions during this anomaly, specifically the eruption of Mount Tambora, cannot be overlooked. It is the most significant factor in this important climate anomaly across the globe (Robock 2000[16]). While there were other eruptions during the year of 1815, Tambora is classified as a VEI-7 and an eruption column 45 km tall, eclipsing all others by at least one order of magnitude.
The Volcanic Explosivity Index (VEI) is used to quantify the amount of ejected material with a VEI-7 coming in at 100 km3. Every index value below that is one order of magnitude less. Furthermore, the 1815 eruption occurred during a Dalton Minimum, a period of unusually low solar radiation (Auchmann et al. 2012[17]). Volcanism plays a large role in climate shifts, both locally and globally. This was not always understood and did not enter scientific circles as fact until Krakatau erupted in 1883 and tinted the skies orange (Robock 2000).
The scale of the volcanic eruption will determine the significance of the impact on climate and other chemical processes, but a change will be measured even in the most local of environments. When volcanoes erupt they eject CO2, H2O, H2, SO2, HCl, HF, and many other gases (Meronen et al. 2012). CO2 and H2O are greenhouse gases, responsible for 0.0394% and 0.4% of the atmosphere respectively. Their small ratio disguises their significant role in trapping solar insolation and reradiating it back to Earth.
Global effects
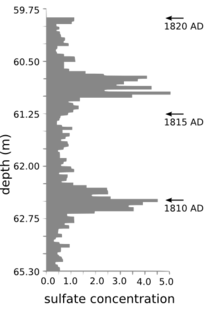
The 1815 eruption released sulfur dioxide (SO2) into the stratosphere, causing a global climate anomaly. Different methods have estimated the ejected sulphur mass during the eruption: the petrological method; an optical depth measurement based on anatomical observations; and the polar ice core sulfate concentration method, using cores from Greenland and Antarctica. The figures vary depending on the method, ranging from 10 to 120 million tonnes.[10]
In the spring and summer of 1815, a persistent "dry fog" was observed in the northeastern United States. The fog reddened and dimmed the sunlight, such that sunspots were visible to the naked eye. Neither wind nor rainfall dispersed the "fog". It was identified as a stratospheric sulfate aerosol veil.[10] In summer 1816, countries in the Northern Hemisphere suffered extreme weather conditions, dubbed the Year Without a Summer. Average global temperatures decreased about 0.4–0.7 °C (0.7–1.3 °F),[5] enough to cause significant agricultural problems around the globe. On 4 June 1816, frosts were reported in Connecticut, and by the following day, most of New England was gripped by the cold front. On 6 June 1816, snow fell in Albany, New York, and Dennysville, Maine.[10] Such conditions occurred for at least three months and ruined most agricultural crops in North America. Canada experienced extreme cold during that summer. Snow 30 cm (12 in) deep accumulated near Quebec City from 6 to 10 June 1816.
The second-coldest year in the Northern Hemisphere since c.1400 was 1816, and the 1810s are the coldest decade on record, a result of Tambora's 1815 eruption and another possible VEI 7 eruption that took place in late 1808 (see sulfate concentration figure from ice core data). The surface temperature anomalies during the summer of 1816, 1817, and 1818 were −0.51 °C (−0.92 °F), −0.44 °C (−0.79 °F) and −0.29 °C (−0.52 °F), respectively.[7] As well as a cooler summer, parts of Europe experienced a stormier winter.
This climate anomaly has been blamed for the severity of typhus epidemics in southeast Europe and the eastern Mediterranean between 1816 and 1819.[10] The climate changes disrupted the Indian monsoons, caused three failed harvests and famine contributing to the spread of a new strain of cholera originating in Bengal in 1816.[19] Many livestock died in New England during the winter of 1816–1817. Cool temperatures and heavy rains resulted in failed harvests in Britain and Ireland. Families in Wales travelled long distances as refugees, begging for food. Famine was prevalent in north and southwest Ireland, following the failure of wheat, oat, and potato harvests. The crisis was severe in Germany, where food prices rose sharply and demonstrations in front of grain markets and bakeries, followed by riots, arson, and looting, took place in many European cities. It was the worst famine of the 19th century.[10]
Effects of volcanism
Volcanism affects the atmosphere in two distinct ways: short-term cooling due to reflected insolation, and long-term warming due to increased CO2 levels. Most of the water vapor and CO2 is collected in clouds within a few weeks to months because both are already present in large quantities, so the effects are limited (Bodenmann et al. 2011). SO2, along with other aerosols and particulates, is responsible for global cooling, nullifying the effects of the greenhouse gas emissions due to its ability to be found higher in the atmosphere and its efficiency at bonding with any water vapor found in the upper "dry" atmosphere. Sulfuric acid is exceptional at blocking solar radiation and it usually takes months to years for it to acquire enough water vapor to fall back to Earth. It has been suggested that a volcanic eruption in 1809 may have previously contributed to a reduction in global temperatures.[18]
Impact of the eruption
By most calculations, the eruption of Tambora was at least a full order of magnitude larger than that of Mount Pinatubo in 1991 (Graft et al. 1993). It is estimated that the top 1,220 metres (4,000 ft) of the mountain was reduced to rubble ash, effectively reducing its height by 33%. Around 100 cubic kilometers of rock was blasted into the air, eclipsing the estimated 10 cubic kilometers by its counterpart in Italy, Vesuvius (Williams 2012). Not only were rocks and ash expelled into the atmosphere, but toxic gases were pumped into the atmosphere as well. Many of the residents who survived the resulting tsunami, eruption, or ash cloud became sick due to all of the sulfur, which caused lung infections (Cole-Dai et al. 2009). Volcanic ash was documented to be over 100 cm deep in areas within 75 km of the eruption, while areas within a 500 km radius saw a 5 cm ash fall, and ash could be found as far away as 1300 km.[10] With this much volcanic ash on the ground, any crops or viable vegetation sources were smothered at a minimum and burned if they were close to the volcano itself. This created an immediate shortage of food in Indonesia, one that only compounded the regular shortage during the winter season (Cole-Dai et al. 2009). The ejection of these gasses, especially HCl, caused the precipitation that followed in the region to be extremely acidic, killing much of the crops that either survived or were rebudding during the spring. The food shortage was compounded by the Napoleonic wars, floods, and cholera.[10]
The presence of ash in the atmosphere for several months after the eruption reflected significant amounts of solar radiation, causing unseasonably cool summers which further drove populations to a food shortage.[10] China, Europe, and North America all had well-documented cases of abnormal temperatures, devastating their harvests. These climatic shifts also altered the monsoon season in China and India, forcing thousands of Chinese to flee coastal areas due to regional flooding of the Yangtze Valley (Granados et al. 2012). The gases also reflected some of the already-decreased incoming solar radiation, causing a notable decrease in global temperatures throughout the decade, between 0.4-0.7 °C globally. It was so dramatic that that an ice dam was formed in Switzerland during the summer of 1816 and 1817, earning 1816 the title "Year without a Summer" or YWAS (Bodenmann et al. 2011). The winter months of 1816 were not very different from years previous, but the spring and summer maintained the cool-to-freezing temperatures. However, the winter of 1817 radically differed, reaching temperatures below -30 °F in New York, which were cold enough to freeze lakes and rivers used for transporting supplies. Both Europe and North America suffered late freezes that lasted well into June with snow accumulating up to 32 cm in August, which killed recently planted crops, crippling the food industry. Unseasonably cool temperatures reduced the output of crops worldwide: the growing seasons in Massachusetts and New Hampshire were less than 80 days in 1816, citing freezing temperatures as the reason for harvest failure (Oppenheimer 2003). These were visually connected to unique sunsets observed in western Europe and red fog found on the Eastern Seaboard of the US. These unique atmospheric conditions persisted for the better part of 2.5 years (Robock 2000).
Ice cores have been used to monitor atmospheric gases during the cold decade (1810-1819) and the results are puzzling. The SO4 concentration found in both Siple Station, Antarctica and Central Greenland bounced from 5.0 in January 1816 to 1.1 in August 1818.[18] This means that 25-30 Tg of sulfur was ejected into the atmosphere, most of which would come from Tambora, and was equalized back by natural processes on Earth rather quickly. Another unique factor is that Tambora represents the largest shift in sulfur concentration in the ice cores for the past 5000 years, potentially becoming the single most disruptive event in recorded history. Estimates of the sulfur yield vary from 10 Tg (Black et al. 2012) to 120 Tg (Stothers 2000). The difference between the models are drastic, but many estimates will either average in or agree on a number between 25-30 Tg. The high concentration might explain the stratospheric warming of ~15 °C, resulting in surface cooling that would be a delayed reaction lasting for the next nine years. It is estimated that the stratospheric warming event only lasted four years, but cooler temperatures were documented until 1825 (Cole-Dai et al. 2009). The data presented did not state whether it was a statistically significant difference or just temperatures cooler than "normal." This has been dubbed a "volcanic winter", similar to a nuclear winter, due to the overall decrease and abysmal farming conditions.[10]
Climate data have shown that the variance between daily lows and highs may have played a role in the lower average temperature because the fluctuations were much more subdued. Generally, the mornings were warmer due to nightly cloud cover and the evenings were cooler because the clouds had dissipated. There were documented fluctuations of cloud cover for various locations that suggested it was a nightly occurrence and the sun killed them off, much like a fog[10] The class boundaries between 1810-1830 without volcanically perturbed years was ~7.9 °C. This is contrasted by the volcanically perturbed years (1815-1817) where the delta was only ~2.3 °C. This meant that the mean annual cycle in 1816 was more linear than bell shaped and 1817 endured cooling across the board. Southeastern England, northern France, and the Netherlands experienced the greatest amount of cooling in Europe; complemented by New York, New Hampshire, Delaware, and Rhode Island in North America (Bodenmann et al. 2011).
The documented rainfall was as much as 80 percent more than the calculated normal with regards to 1816, unusually high amounts of snow were found in Switzerland, France, Germany, and Poland. This is again contrasted by the unusually low precipitations in 1818 which caused droughts throughout most of Europe and Asia (Auchmann et al. 2012). Russia had already experienced unseasonably warm and dry summers since 1815 and this continued for the next three years. There are also documented reductions in ocean temperature near the Baltic Sea, North Sea, and Mediterranean. This seems to have been an indicator of shifted oceanic circulation patterns and possibly changed wind direction and speed (Meronen et al. 2012). This is further supported by the recorded observations of a British fleet sent to explore the Arctic Circle; they found large ice sheets miles off the coast of Greenland, where two years prior they had been shoved along the east coast of Greenland. Contemporary scientists attributed the Year Without a Summer to the drifting polar ice sheets rather than the eruption of Tambora because of its proximity to England.[10]
Taking into account the Dalton Minimum, and the presence of famine and droughts predating the eruption, the Tambora volcanic event accelerated or exacerbated the extreme climate conditions of 1815. While other eruptions and other climatological events would have led to a global cooling of about 0.2 °C, Tambora increased that number substantially.[18]
Comparison of selected volcanic eruptions
Eruptions | Country | Location | Year | Column height (km) | Volcanic Explosivity Index | N. Hemisphere summer anomaly (°C) | Fatalities |
---|---|---|---|---|---|---|---|
Mount Vesuvius | Italy | Mediterranean | 79 | 30 | 5 | ? | >2,000 |
Hatepe (Taupo) | New Zealand | Pacific Ring of Fire | 186 | 37 | 7 | ? | ? |
Baekdu | China / North Korea | Pacific Ring of Fire | 969 | 36 | 6–7 | ? | ? |
Huaynaputina | Peru | Pacific Ring of Fire | 1600 | 46 | 6 | −0.8 | ≈1,400 |
Tambora | Dutch East-Indies | Pacific Ring of Fire | 1815 | 43 | 7 | −0.5 | >71,000 |
Krakatoa | Dutch East-Indies | Pacific Ring of Fire | 1883 | 36 | 6 | −0.3 | 36,600 |
Santa María | Guatemala | Pacific Ring of Fire | 1902 | 34 | 6 | no anomaly | 7,000–13,000 |
Novarupta | USA, Alaska | Pacific Ring of Fire | 1912 | 32 | 6 | −0.4 | 2 |
Mt. St. Helens | USA, Washington | Pacific Ring of Fire | 1980 | 19 | 5 | no anomaly | 57 |
El Chichón | Mexico | Pacific Ring of Fire | 1982 | 32 | 4–5 | YES | >2,000 |
Nevado del Ruiz | Colombia | Pacific Ring of Fire | 1985 | 27 | 3 | no anomaly | 23,000 |
Pinatubo | Philippines | Pacific Ring of Fire | 1991 | 49 | 6 | −0.5 | 1,202 |
Source: Oppenheimer (2003),[10] and Smithsonian Global Volcanism Program for VEI.[20]
See also
References
This article incorporates public domain material from websites or documents of the United States Geological Survey.
- ↑ Earth Observatory
- ↑ "Tambora Volcano, Indonesia". United States Geological Survey. 3 June 2002. Retrieved 8 September 2010.
- ↑ Climate Forcing
- 1 2 Foden, J. (1986). "The petrology of Tambora volcano, Indonesia: A model for the 1815 eruption". Journal of Volcanology and Geothermal Research 27 (1–2): 1–41. Bibcode:1986JVGR...27....1F. doi:10.1016/0377-0273(86)90079-X.
- 1 2 3 4 5 6 7 8 9 10 11 12 13 Stothers, Richard B. (1984). "The Great Tambora Eruption in 1815 and Its Aftermath". Science 224 (4654): 1191–1198. Bibcode:1984Sci...224.1191S. doi:10.1126/science.224.4654.1191. PMID 17819476.
- 1 2 3 4 5 6 Raffles, S. 1830: Memoir of the life and public services of Sir Thomas Stamford Raffles, F.R.S. &c., particularly in the government of Java 1811–1816, and of Bencoolen and its dependencies 1817–1824: with details of the commerce and resources of the eastern archipelago, and selections from his correspondence. London: John Murray, cited by Oppenheimer (2003).
- 1 2 Briffa, K.R.; Jones, P.D.; Schweingruber, F.H.; Osborn, T.J. (1998). "Influence of volcanic eruptions on Northern Hemisphere summer temperature over 600 years". Nature 393 (6684): 450–455. Bibcode:1998Natur.393..450B. doi:10.1038/30943.
- ↑ Stothers, Richard B. (2004). "Density of fallen ash after the eruption of Tambora in 1815". Journal of Volcanology and Geothermal Research 134 (4): 343–345. Bibcode:2004JVGR..134..343S. doi:10.1016/j.jvolgeores.2004.03.010.
- ↑ Monk, K.A.; Fretes, Y.; Reksodiharjo-Lilley, G. (1996). The Ecology of Nusa Tenggara and Maluku. Hong Kong: Periplus Editions Ltd. p. 60. ISBN 962-593-076-0.
- 1 2 3 4 5 6 7 8 9 10 11 12 13 14 15 16 Oppenheimer, Clive (2003). "Climatic, environmental and human consequences of the largest known historic eruption: Tambora volcano (Indonesia) 1815". Progress in Physical Geography 27 (2): 230–259. doi:10.1191/0309133303pp379ra.
- ↑ USGS account of historical volcanic induced tsunamis. Hvo.wr.usgs.gov. Retrieved 26 April 2012.
- ↑ Zollinger (1855): Besteigung des Vulkans Tamboro auf der Insel Sumbawa und Schilderung der Eruption desselben im Jahre 1815, Winterthur: Zurcher and Fürber, Wurster and Co., cited by Oppenheimer (2003).
- ↑ Petroeschevsky (1949): A contribution to the knowledge of the Gunung Tambora (Sumbawa). Tijdschrift van het K. Nederlandsch Aardrijkskundig Genootschap, Amsterdam Series 2 66, 688–703, cited by Oppenheimer (2003).
- 1 2 Tanguy, J.-C.; Scarth, A.; Ribière, C.; Tjetjep, W. S. (1998). "Victims from volcanic eruptions: a revised database". Bulletin of Volcanology 60 (2): 137–144. Bibcode:1998BVol...60..137T. doi:10.1007/s004450050222.
- ↑ Anthony Reid, 'Lessons of Tambora ignored, 200 years on', 25 April 2015, East Asia Forum, Australian National University, accessed 27 April 2015.
- ↑ http://climate.envsci.rutgers.edu/pdf/ROG2000.pdf
- ↑ http://www.academia.edu/2961986/Extreme_climate_not_extreme_weather_the_summer_of_1816_in_Geneva_Switzerland
- 1 2 3 4 Dai, Jihong; Mosley-Thompson, Ellen; Thompson, Lonnie G. (1991). "Ice core evidence for an explosive tropical volcanic eruption six years preceding Tambora". Journal of Geophysical Research: Atmospheres 96: 17, 361–17, 366. Bibcode:1991JGR....9617361D. doi:10.1029/91jd01634.
- ↑ Peterson, Doug LAS News (Spring 2010) University of Illinois at Urbana-Champaign p. 11.
- ↑ "Large Holocene Eruptions". Global Volcanism Program. Smithsonian Institution. Retrieved 7 November 2006.