T cell
T cell | |
---|---|
![]() Scanning electron micrograph of a human T cell | |
![]() Scanning electron micrograph of T lymphocyte (right), a platelet (center) and a red blood cell (left) | |
Details | |
System | Immune system |
Identifiers | |
Latin | lymphocytus T |
Code | TH H2.00.04.1.02007 |

T cells (also known as Thymocytes cells) or T lymphocytes are a type of lymphocyte (in turn, a type of white blood cell) that play a central role in cell-mediated immunity. They can be distinguished from other lymphocytes, such as B cells and natural killer cells (NK cells), by the presence of a T-cell receptor (TCR) on the cell surface. They are called T cells because they mature in the thymus[1] (although some also mature in the tonsils[2]). The several subsets of T cells each have a distinct function. The majority of human T cells rearrange their alpha/beta T cell receptors and are termed alpha beta T cells and are part of adaptive immune system. Specialized gamma delta T cells, which comprise a minority of T cells in the human body (more frequent in ruminants), have invariant TCR (with limited diversity), can effectively present antigens to other T cells[3] and are considered to be part of the innate immune system.
Types
Helper
T helper cells (TH cells) assist other white blood cells in immunologic processes, including maturation of B cells into plasma cells and memory B cells, and activation of cytotoxic T cells and macrophages. These cells are also known as CD4+ T cells because they express the CD4 glycoprotein on their surfaces. Helper T cells become activated when they are presented with peptide antigens by MHC class II molecules, which are expressed on the surface of antigen-presenting cells (APCs). Once activated, they divide rapidly and secrete small proteins called cytokines that regulate or assist in the active immune response. These cells can differentiate into one of several subtypes, including TH1, TH2, TH3, TH17, Th9, or TFH, which secrete different cytokines to facilitate different types of immune responses. Signalling from the APC directs T cells into particular subtypes.[4]
Cytotoxic
Cytotoxic T cells (TC cells, or CTLs) destroy virus-infected cells and tumor cells, and are also implicated in transplant rejection. These cells are also known as CD8+ T cells since they express the CD8 glycoprotein at their surfaces. These cells recognize their targets by binding to antigen associated with MHC class I molecules, which are present on the surface of all nucleated cells. Through IL-10, adenosine, and other molecules secreted by regulatory T cells, the CD8+ cells can be inactivated to an anergic state, which prevents autoimmune diseases.
Memory
Memory T cells are a subset of antigen-specific T cells that persist long-term after an infection has resolved. They quickly expand to large numbers of effector T cells upon re-exposure to their cognate antigen, thus providing the immune system with "memory" against past infections. Memory T cells comprise three subtypes: central memory T cells (TCM cells), effector memory T cells (TEM cells and TEMRA cells)[5] and resident memory T cells (TRM).[6] Memory cells may be either CD4+ or CD8+. Memory T cells typically express the cell surface protein CD45RO.[7]
Suppressor
Suppressor T cells are crucial for the maintenance of immunological tolerance. Their major role is to shut down T cell-mediated immunity toward the end of an immune reaction and to suppress autoreactive T cells that escaped the process of negative selection in the thymus. Suppressor T cells along with Helper T cells can collectively be called Regulatory T cells due to their regulatory functions.[8]
Two major classes of CD4+ Treg cells have been described — FOXP3+ Treg cells and FOXP3− Treg cells.
Regulatory T cells can develop either during normal development in the thymus, and are then known as thymic Treg cells, or can be induced peripherally and are called peripherally derived Treg cells. These two subsets were previously called "naturally occurring", and "adaptive" or "induced", respectively.[9] Both subsets require the expression of the transcription factor FOXP3 which can be used to identify the cells. Mutations of the FOXP3 gene can prevent regulatory T cell development, causing the fatal autoimmune disease IPEX.
Several other types of T cell have suppressive activity, but do not express FOXP3. These include Tr1 cells and Th3 cells, which are thought to originate during an immune response and act by producing suppressive molecules. Tr1 cells are associated with IL-10, and Th3 cells are associated with TGF-beta. Recently, Treg17 cells have been added to this list.[10]
Natural killer
Natural killer T cells (NKT cells – not to be confused with natural killer cells of the innate immune system) bridge the adaptive immune system with the innate immune system. Unlike conventional T cells that recognize peptide antigens presented by major histocompatibility complex (MHC) molecules, NKT cells recognize glycolipid antigen presented by a molecule called CD1d. Once activated, these cells can perform functions ascribed to both Th and Tc cells (i.e., cytokine production and release of cytolytic/cell killing molecules). They are also able to recognize and eliminate some tumor cells and cells infected with herpes viruses.
Mucosal associated invariant
Mucosal associated invariant T cells are a special type of T cell.
Gamma delta T cells
Gamma delta T cells (γδ T cells) represent a small subset of T cells that possess a distinct T cell receptor (TCR) on their surfaces. A majority of T cells have a TCR composed of two glycoprotein chains called α- and β- TCR chains. However, in γδ T cells, the TCR is made up of one γ-chain and one δ-chain. This group of T cells is much less common in humans and mice( about 2% of total T cells); and are found in the highest abundance in the gut mucosa, within a population of lymphocytes known as intraepithelial lymphocytes. In rabbits, sheep, and chickens, the number of γδ T cells can be as high as 60% of total T cells. The antigenic molecules that activate γδ T cells are still widely unknown. However, γδ T cells are not MHC-restricted and seem to be able to recognize whole proteins rather than requiring peptides to be presented by MHC molecules on APCs. Some murine γδ T cells recognize MHC class IB molecules, though. Human Vγ9/Vδ2 T cells, which constitute the major γδ T cell population in peripheral blood, are unique in that they specifically and rapidly respond to a set of nonpeptidic phosphorylated isoprenoid precursors, collectively named phosphoantigens, which are produced by virtually all living cells. The most common phosphoantigens from animal and human cells (including cancer cells) are isopentenyl pyrophosphate (IPP) and its isomer dimethylallyl pyrophosphate (DMPP). Many microbes produce the highly active compound hydroxy-DMAPP (HMB-PP) and corresponding mononucleotide conjugates, in addition to IPP and DMAPP. Plant cells produce both types of phosphoantigens. Drugs activating human Vγ9/Vδ2 T cells comprise synthetic phosphoantigens and aminobisphosphonates, which upregulate endogenous IPP/DMAPP.
Development in the thymus
All T cells originate from haematopoietic stem cells in the bone marrow. Haematopoietic progenitors (lymphoid progenitor cells) from haematopoietic stem cells populate the thymus and expand by cell division to generate a large population of immature thymocytes.[11] The earliest thymocytes express neither CD4 nor CD8, and are therefore classed as double-negative (CD4−CD8−) cells. As they progress through their development, they become double-positive thymocytes (CD4+CD8+), and finally mature to single-positive (CD4+CD8− or CD4−CD8+) thymocytes that are then released from the thymus to peripheral tissues.
About 98% of thymocytes die during the development processes in the thymus by failing either positive selection or negative selection, whereas the other 2% survive and leave the thymus to become mature immunocompetent T cells. Increasing evidence indicates microRNAs, which are small noncoding regulatory RNAs, could impact the clonal selection process during thymic development. For example, miR-181a was found to play a role in the positive selection of T lymphocytes.[12]
The thymus contributes fewer cells as a person ages. As the thymus shrinks by about 3%[13] a year throughout middle age, a corresponding fall in the thymic production of naive T cells occurs, leaving peripheral T cell expansion to play a greater role in protecting older subjects.
Beta selection
Common lymphoid precursor cells that migrate to the thymus become known as T-cell precursors (or thymocytes) and do not express a T cell receptor. Broadly speaking, the double negative (DN) stage is focused on producing a functional β-chain whereas the double positive (DP) stage is focused on producing a functional α-chain, ultimately producing a functional αβ T cell receptor. As the developing thymocyte progresses through the four DN stages (DN1, DN2, DN3, and DN4), the T cell expresses an invariant α-chain but rearranges the β-chain locus. If the rearranged β-chain successfully pairs with the invariant α-chain, signals are produced which cease rearrangement of the β-chain (and silence the alternate allele) and result in proliferation of the cell.[14] Although these signals require this pre-TCR at the cell surface, they are independent of ligand binding to the pre-TCR. These thymocytes will then express both CD4 and CD8 and progresses to the double positive (DP) stage where selection of the α-chain takes place. If a rearranged β-chain does not lead to any signalling (e.g. as a result of an inability to pair with the invariant α-chain), the cell may die by neglect (lack of signalling).
Positive selection
Positive selection "selects for" T cells capable of interacting with MHC. Double-positive thymocytes (CD4+/CD8+) move deep into the thymic cortex, where they are presented with self-antigens. These self-antigens are expressed by thymic cortical epithelial cells on MHC molecules on the surface of cortical epithelial cells. Only those thymocytes that interact with MHC-I or MHC-II appropriately (i.e., not too strongly or too weakly) will receive a vital "survival signal". All that cannot (i.e., if they do not interact strongly enough, or if they bind too strongly) will die by "death by neglect" (no survival signal). This process ensures that the selected T-cells will have an MHC affinity that can serve useful functions in the body (i.e., the cells must be able to interact with MHC and peptide complexes to effect immune responses). The vast majority of all thymocytes end up dying during this process.
A thymocyte's fate is determined during positive selection. Double-positive cells (CD4+/CD8+) that interact well with MHC class II molecules will eventually become CD4+ cells, whereas thymocytes that interact well with MHC class I molecules mature into CD8+ cells. A T cell becomes a CD4+ cell by down-regulating expression of its CD8 cell surface receptors. If the cell does not lose its signal, it will continue downregulating CD8 and become a CD4+, single positive cell. But, if there is a signal interruption, the cell stops downregulating CD8 and switches over to downregulating CD4 molecules, instead, eventually becoming a CD8+, single positive cell.
This process does not remove thymocytes that may cause autoimmunity. The potentially autoimmune cells are removed by the process of negative selection, which occurs in the thymic medulla (discussed below).
Negative selection
Negative selection removes thymocytes that are capable of strongly binding with "self" MHC peptides. Thymocytes that survive positive selection migrate towards the boundary of the cortex and medulla in the thymus. While in the medulla, they are again presented with a self-antigen presented on the MHC complex of medullary thymic epithelial cells (mTECs).[15] mTECs must be AIRE+ to properly express self-antigens from all tissues of the body on their MHC class I peptides. Some mTECs are phagocytosed by thymic dendritic cells; this allows for presentation of self-antigens on MHC class II molecules (positively selected CD4+ cells must interact with MHC class II molecules, thus APCs, which possess MHC class II, must be present for CD4+ T-cell negative selection). Thymocytes that interact too strongly with the self-antigen receive an apoptotic signal that leads to cell death. However, some of these cells are selected to become Treg cells. The remaining cells exit the thymus as mature naive T cells (also known as recent thymic emigrants). This process is an important component of central tolerance and serves to prevent the formation of self-reactive T cells that are capable of inducing autoimmune diseases in the host.
In summary, β-selection is the first checkpoint, where the T cells that are able to form a functional pre-TCR with an invariant alpha chain and a functional beta chain are allowed to continue development in the thymus. Next, positive selection checks that T cells have successfully rearranged their TCRα locus and are capable of recognizing peptide-MHC complexes with appropriate affinity. Negative selection in the medulla then obliterates T cells that bind too strongly to self-antigens expressed on MHC molecules. These selection processes allow for tolerance of self by the immune system. Typical T-cells that leave the thymus (via the corticomedullarly junction) are self-restricted, self-tolerant, and singly positive.
Activation
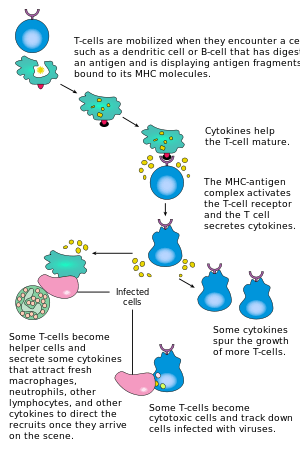
Activation of CD4+ T cells occurs through the simultaneous engagement of the T cell receptor and a costimulatory molecule (like CD28, or ICOS) on the T cell by the major histocompatibility complex (MHCII) peptide and costimulatory molecules on the APC. Both are required for production of an effective immune response; in the absence of costimulation, T-cell receptor signalling alone results in anergy. The signalling pathways downstream from costimulatory molecules usually engages the PI3K pathway generating PIP3 at the plasma membrane and recruiting PH domain containing signaling molecules like PDK1 that are essential for the activation of PKCθ, and eventual IL-2 production.
The first signal is provided by binding of the T cell receptor to its cognate peptide presented on MHCII on an APC. MHCII is restricted to so-called professional APCs, like dendritic cells, B cells, and macrophages, to name a few. The peptides presented to CD8+ T cells by MHC class I molecules are 8–9 amino acids in length; the peptides presented to CD4+ cells by MHC class II molecules are longer, usually 12–25 amino acids in length,[17] as the ends of the binding cleft of the MHC class II molecule are open.
The second signal comes from costimulation, in which surface receptors on the APC are induced by a relatively small number of stimuli, usually products of pathogens, but sometimes breakdown products of cells, such as necrotic-bodies or heat shock proteins. The only costimulatory receptor expressed constitutively by naïve T cells is CD28, so costimulation for these cells comes from the CD80 and CD86 proteins, which together constitute the B7 protein, (B7.1 and B7.2, respectively) on the APC. Other receptors are expressed upon activation of the T cell, such as OX40 and ICOS, but these largely depend upon CD28 for their expression. The second signal licenses the T cell to respond to an antigen. Without it, the T cell becomes anergic, and it becomes more difficult for it to activate in future. This mechanism prevents inappropriate responses to self, as self-peptides will not usually be presented with suitable costimulation. Once a T cell has been appropriately activated (i.e. has received signal one and signal two) it alters its cell surface expression of a variety of proteins. Markers of T cell activation include CD69, CD71 and CD25 (also a marker for Treg cells), and HLA-DR (a marker of human T cell activation). CTLA-4 expression is also up-regulated on activated T cells, which in turn outcompetes CD28 for binding to the B7 proteins. This is a checkpoint mechanism to prevent over activation of the T cell. Activated T cells also change their cell surface glycosylation profile.[18]
The T cell receptor exists as a complex of several proteins. The actual T cell receptor is composed of two separate peptide chains, which are produced from the independent T cell receptor alpha and beta (TCRα and TCRβ) genes. The other proteins in the complex are the CD3 proteins: CD3εγ and CD3εδ heterodimers and, most important, a CD3ζ homodimer, which has a total of six ITAM motifs. The ITAM motifs on the CD3ζ can be phosphorylated by Lck and in turn recruit ZAP-70. Lck and/or ZAP-70 can also phosphorylate the tyrosines on many other molecules, not least CD28, LAT and SLP-76, which allows the aggregation of signalling complexes around these proteins.
Phosphorylated LAT recruits SLP-76 to the membrane, where it can then bring in PLC-γ, VAV1, Itk and potentially PI3K. PLC-γ cleaves PI(4,5)P2 on the inner leaflet of the membrane to create the active intermediaries diacylglycerol (DAG), inositol-1,4,5-trisphosphate (IP3); PI3K also acts on PIP2, phosphorylating it to produce phosphatidlyinositol-3,4,5-trisphosphate (PIP3). DAG binds and activates some PKCs. Most important in T cells is PKCθ, critical for activating the transcription factors NF-κB and AP-1. IP3 is released from the membrane by PLC-γ and diffuses rapidly to activate calcium channel receptors on the ER, which induces the release of calcium into the cytosol. Low calcium in the endoplasmic reticulum causes STIM1 clustering on the ER membrane and leads to activation of cell membrane CRAC channels that allows additional calcium to flow into the cytosol from the extracellular space. This aggregated cytosolic calcium binds calmodulin, which can then activate calcineurin. Calcineurin, in turn, activates NFAT, which then translocates to the nucleus. NFAT is a transcription factor that activates the transcription of a pleiotropic set of genes, most notable, IL-2, a cytokine that promotes long-term proliferation of activated T cells.
PLCγ can also initiate the NF-κB pathway. DAG activates PKCθ, which then phosphorylates CARMA1, causing it to unfold and function as a scaffold. The cytosolic domains bind an adapter BCL10 via CARD (Caspase activation and recruitment domains) domains; that then binds TRAF6, which is ubiquitinated at K63.:513–523[19] This form of ubiquitination does not lead to degradation of target proteins. Rather, it serves to recruit NEMO, IKKα and -β, and TAB1-2/ TAK1.[20] TAK 1 phosphorylates IKK-β, which then phosphorylates IκB allowing for K48 ubiquitination: leads to proteosomal degradation. Rel A and p50 can then enter the nucleus and bind the NF-κB response element. This coupled with NFAT signaling allows for complete activation of the IL-2 gene.[19]
While in most cases activation is dependent on TCR recognition of antigen, alternative pathways for activation have been described. For example, cytotoxic T cells have been shown to become activated when targeted by other CD8 T cells leading to tolerization of the latter.[21]
In spring 2014, the T-Cell Activation in Space (TCAS) experiment was launched to the International Space Station on the SpaceX CRS-3 mission to study how "deficiencies in the human immune system are affected by a microgravity environment"[22]
Antigen discrimination
A unique feature of T cells is their ability to discriminate between healthy and abnormal (e.g. infected or cancerous) cells in the body.[23] Healthy cells typically express a large number of self derived pMHC on their cell surface and although the T cell antigen receptor can interact with at least a subset of these self pMHC, the T cell generally ignores these healthy cells. However, when these very same cells contain even minute quantities of pathogen derived pMHC, T cells are able to become activated and initiate immune responses. The ability of T cells to ignore healthy cells but respond when these same cells contain pathogen (or cancer) derived pMHC is known as antigen discrimination. The molecular mechanisms that underlie this process are controversial.[23][24]
Genetic engineering
In 2015, a team of researchers led by Dr. Alexander Marson[25]at the University of California, San Francisco successfully edited the genome of human T cells using a Cas9 ribonucleoprotein delivery method.[26] This advancement has potential for applications in treating "cancer immunotherapies and cell-based therapies for HIV, primary immune deficiencies, and autoimmune diseases".[26]
Disorders
Deficiency
Causes of T cell deficiency include lymphocytopenia of T cells and/or defects on function of individual T cells. Complete insufficiency of T cell function can result from hereditary conditions such as severe combined immunodeficiency (SCID), Omenn syndrome, and Cartilage-hair hypoplasia.[27] Causes of partial insufficiencies of T cell function include acquired immune deficiency syndrome (AIDS), and hereditary conditions such as DiGeorge syndrome (DGS), chromosomal breakage syndromes (CBSs), and B-cell and T-cell combined disorders such as ataxia telangiectasia (AT) and Wiskott-Aldrich syndrome (WAS).[27]
The main pathogens of concern in T cell deficiencies are intracellular pathogens, including Herpes simplex virus, Mycobacterium and Listeria.[28] Also, fungal infections are also more common and severe in T cell deficiencies.[28]
Cancer
Cancer of T cells is termed T-cell lymphoma, and accounts for perhaps one in ten cases of non-Hodgkin lymphoma.[29] The main forms of T cell lymphoma are:
- Extranodal T cell lymphoma
- Cutaneous T cell lymphomas: Sézary syndrome and Mycosis fungoides
- Anaplastic large cell lymphoma
- Angioimmunoblastic T cell lymphoma
T-cell exhaustion
T-cell exhaustion is the progressive loss of T-cell function. It can occur during sepsis and after other acute or chronic infections.[30][31]
T-cell exhaustion is mediated by several inhibitory receptors including programmed cell death protein 1 (PD1), TIM3, and lymphocyte activation gene 3 protein (LAG3).[32]
( See also Immunosenescence#T-cell_functional_dysregulation_as_a_biomarker_for_immunosenescence )
See also
References
- ↑ Alberts B, Johnson A, Lewis J, Raff M, Roberts k, Walter P (2002) Molecular Biology of the Cell. Garland Science: New York, NY pg 1367. "T cells and B cells derive their names from the organs in which they develop. T cells develop in the thymus, and B cells, in mammals, develop in the bone marrow in adults or the liver in fetuses."
- ↑ McClory, Susan; Hughes, Tiffany; Freud, Aharon G.; Briercheck, Edward L.; Martin, Chelsea; Trimboli, Anthony J.; Yu, Jianhua; Zhang, Xiaoli; Leone, Gustavo (Apr 2012). "Evidence for a stepwise program of extrathymic T cell development within the human tonsil". The Journal of Clinical Investigation 122 (4): 1403–1415. doi:10.1172/JCI46125. ISSN 1558-8238. PMC 3314444. PMID 22378041.
- ↑ Vantourout, Pierre; Hayday, Adrian (Feb 2013). "Six-of-the-best: unique contributions of γδ T cells to immunology". Nature Reviews. Immunology 13 (2): 88–100. doi:10.1038/nri3384. ISSN 1474-1741. PMC 3951794. PMID 23348415.
- ↑ Gutcher I, Becher B (2007). "APC-derived cytokines and T cell polarization in autoimmune inflammation". J. Clin. Invest. 117 (5): 1119–27. doi:10.1172/JCI31720. PMC 1857272. PMID 17476341.
- ↑ Willinger T, Freeman T, Hasegawa H, McMichael AJ, Callan MF (2005). "Molecular signatures distinguish human central memory from effector memory CD8 T cell subsets.". Journal of Immunology 175 (9): 5895–903. doi:10.4049/jimmunol.175.9.5895. PMID 16237082.
- ↑ Shin, Haina; Iwasaki, Akiko (2013-09-01). "Tissue-resident memory T cells". Immunological Reviews 255 (1): 165–181. doi:10.1111/imr.12087. ISSN 1600-065X. PMC 3748618. PMID 23947354.
- ↑ Akbar AN, Terry L, Timms A, Beverley PC, Janossy G (April 1988). "Loss of CD45R and gain of UCHL1 reactivity is a feature of primed T cells". J. Immunol. 140 (7): 2171–8. PMID 2965180.
- ↑ Textbook of Medical Physiology by Guyton and Hall, edition 6, pg. 448, Suppressor T cells paragraph.
- ↑ Abbas AK, Benoist C, Bluestone JA, Campbell DJ, Ghosh S, Hori S, Jiang S, Kuchroo VK, Mathis D, Roncarolo MG, Rudensky A, Sakaguchi S, Shevach EM, Vignali DA, Ziegler SF (2013). "Regulatory T cells: recommendations to simplify the nomenclature". Nat. Immunol. 14 (4): 307–8. doi:10.1038/ni.2554. PMID 23507634.
- ↑ Singh B, Schwartz JA, Sandrock C, Bellemore SM, Nikoopour E (2013). "Modulation of autoimmune diseases by interleukin (IL)-17 producing regulatory T helper (Th17) cells". Indian J. Med. Res. 138 (5): 591–4. PMC 3928692. PMID 24434314.
- ↑ Schwarz BA, Bhandoola A (February 2006). "Trafficking from the bone marrow to the thymus: a prerequisite for thymopoiesis". Immunol. Rev. 209: 47–57. doi:10.1111/j.0105-2896.2006.00350.x. PMID 16448533.
- ↑ Li QJ, Chau J, Ebert PJ, Sylvester G, Min H, Liu G, Braich R, Manoharan M, Soutschek J, Skare P, Klein LO, Davis MM, Chen CZ (2007). "miR-181a is an intrinsic modulator of T cell sensitivity and selection". Cell 129 (1): 147–61. doi:10.1016/j.cell.2007.03.008. PMID 17382377.
- ↑ Haynes BF, Markert ML, Sempowski GD, Patel DD, Hale LP (2000). "The role of the thymus in immune reconstitution in aging, bone marrow transplantation, and HIV-1 infection". Annu. Rev. Immunol. 18: 529–60. doi:10.1146/annurev.immunol.18.1.529. PMID 10837068.
- ↑ Janeway, Charles (2012). Immunobiology. Garland Science. pp. 301–305. ISBN 9780815342434.
- ↑ Hinterberger M, Aichinger M, Prazeres da Costa O, Voehringer D, Hoffmann R, Klein L (June 2010). "Autonomous role of medullary thymic epithelial cells in central CD4(+) T cell tolerance". Nat. Immunol. 11 (6): 512–9. doi:10.1038/ni.1874. PMID 20431619.
- ↑ The NIAID resource booklet "Understanding the Immune System (pdf)".
- ↑ Jennifer Rolland and Robyn O'Hehir, "Turning off the T-cells: Peptides for treatment of allergic Diseases," Today's life science publishing, 1999, Page 32
- ↑ Maverakis E, Kim K, Shimoda M, Gershwin M, Patel F, Wilken R, Raychaudhuri S, Ruhaak LR, Lebrilla CB (2015). "Glycans in the immune system and The Altered Glycan Theory of Autoimmunity". J Autoimmun 57 (6): 1–13. doi:10.1016/j.jaut.2014.12.002. PMID 25578468.
- 1 2 Tatham P, Gomperts BD, Kramer IM (2003). Signal transduction. Amsterdam: Elsevier Academic Press. ISBN 0-12-289632-7.
- ↑ Wu H, Arron JR (November 2003). "TRAF6, a molecular bridge spanning adaptive immunity, innate immunity and osteoimmunology". BioEssays 25 (11): 1096–105. doi:10.1002/bies.10352. PMID 14579250.
- ↑ Milstein O, Hagin D, Lask A, Reich-Zeliger S, Shezen E, Ophir E, Eidelstein Y, Afik R, Antebi YE, Dustin ML, Reisner Y (January 2011). "CTLs respond with activation and granule secretion when serving as targets for T-cell recognition". Blood 117 (3): 1042–52. doi:10.1182/blood-2010-05-283770. PMC 3035066. PMID 21045195.
- ↑ Graham, William (2014-04-14). "SpaceX ready for CRS-3 Dragon launch and new milestones". NASAspaceflight.com. Retrieved 2014-04-14.
- 1 2 Feinerman O, Germain RN, Altan-Bonnet G (2008). "Quantitative challenges in understanding ligand discrimination by alphabeta T cells". Mol. Immunol. 45 (3): 619–31. doi:10.1016/j.molimm.2007.03.028. PMC 2131735. PMID 17825415.
- ↑ Dushek O, van der Merwe PA (2014). "An induced rebinding model of antigen discrimination". Trends Immunol. 35 (4): 153–8. doi:10.1016/j.it.2014.02.002. PMC 3989030. PMID 24636916.
- ↑ http://www.independent.co.uk/news/science/crispr-breakthrough-announced-in-technique-of-editing-dna-to-fight-off-deadly-illnesses-10420050.html
- 1 2 Schumann, Kathrin; Lin, Steven; Boyer, Eric; Simeonov, Dimitre R.; Subramaniam, Meena; Gate, Rachel E.; Haliburton, Genevieve E.; Ye, Chun J.; Bluestone, Jeffrey A. (2015-08-18). "Generation of knock-in primary human T cells using Cas9 ribonucleoproteins". Proceedings of the National Academy of Sciences of the United States of America 112 (33): 10437–10442. doi:10.1073/pnas.1512503112. ISSN 1091-6490. PMC 4547290. PMID 26216948.
- 1 2 Medscape > T-cell Disorders. Author: Robert A Schwartz, MD, MPH; Chief Editor: Harumi Jyonouchi, MD. Updated: May 16, 2011
- 1 2 Jones J, Bannister BA, Gillespie SH, ed. (2006). Infection: Microbiology and Management. Wiley-Blackwell. p. 435. ISBN 1-4051-2665-5.
- ↑ "The Lymphomas" (PDF). The Leukemia & Lymphoma Society. May 2006. p. 2. Retrieved 2008-04-07.
- ↑ John S Yi, Maureen A Cox, and Allan J Zajac (Apr 2010). "T-cell exhaustion: characteristics, causes and conversion". Immunology 129 (4): 474–481. doi:10.1111/j.1365-2567.2010.03255.x. PMC 2842494.
- ↑ Hotchkiss RS, Monneret G, Payen D. (Mar 2013). "Immunosuppression in sepsis: a novel understanding of the disorder and a new therapeutic approach". Lancet Infect Dis 13 (3): 260–8. doi:10.1016/S1473-3099(13)70001-X.
- ↑ Mahoney KM, Rennert PD, Freeman GJ (2015). "Combination cancer immunotherapy and new immunomodulatory targets". Nat Rev Drug Discov 14 (8): 561–84. doi:10.1038/nrd4591. PMID 26228759.
External links
- Immunobiology, 5th Edition
- niaid.nih.gov – The Immune System
- T-cell Group – Cardiff University
- (Successful!) Treatment of Metastatic Melanoma with Autologous CD4+ T Cells against NY-ESO-1.
- Davies AJ (1993). "The tale of T cells". Immunology Today 14 (3): 137–139. doi:10.1016/0167-5699(93)90216-8. PMID 8466629.
|
|