Cyclic compound
A cyclic compound (ring compound) is a term for a compound in the field of chemistry in which one or more series of atoms in the compound is connected to form a ring. Rings may vary in size from three to many atoms, and include examples where all the atoms are carbon (i.e., are carbocycles), none of the atoms are carbon (inorganic cyclic compounds), or where both carbon and non-carbon atoms are present (heterocyclic compounds). Depending on the ring size, the bond order of the individual links between ring atoms, and their arrangements within the rings, carbocyclic and heterocyclic compounds may be aromatic or non-aromatic, in the latter case, they may vary from being fully saturated to having varying numbers of multiple bonds between the ring atoms. Because of the tremendous diversity allowed, in combination, by the valences of common atoms and their ability to form rings, the number of possible cyclic structures, even of small size (e.g., <17 total atoms) numbers in the many billions.
- Cyclic compound examples: All-carbon (carbocyclic) and more complex natural cyclic compounds.
-
Ingenol, a complex, terpenoid natural product, related to but simpler than the paclitaxel that follows, which displays a complex ring structure including 3-, 5-, and 7-membered non-aromatic, carbocyclic rings.
-
Cycloalkanes, the simplest carbocycles, including cyclopropane, cyclobutane, cyclopentane, and cyclohexane. Note, elsewhere an organic chemistry shorthand is used where hydrogen atoms are inferred as present to fill the carbon's valence of 4 (rather than their being shown explicitly).
-
Paclitaxel, another complex, plant-derived terpenoid, also a natural product, displaying a complex multi-ring structure including 4-, 6-, and 8-membered rings (carbocyclic and heterocyclic, aromatic and non-aromatic).
Adding to their complexity and number, closing of atoms into rings may lock particular atoms with distinct substitution (by functional groups) such that stereochemistry and chirality of the compound results, including some manifestations that are unique to rings (e.g., configurational isomers). As well, depending on ring size, the three-dimensional shapes of particular cyclic structures—typically rings of 5-atoms and larger—can vary and interconvert such that conformational isomerism is displayed. Indeed, the development of this important chemical concept arose, historically, in reference to cyclic compounds. Finally, cyclic compounds, because of the unique shapes, reactivities, properties, and bioactivities that they engender, are the largest majority of all molecules involved in the biochemistry, structure, and function of living organisms, and in the man-made molecules (e.g., drugs, herbicides, etc.).
Structural introduction
A cyclic compound or ring compound is a compound at least some of whose atoms are connected to form a ring.[1]:unknown Rings vary in size from 3 to many tens or even hundreds of atoms. Examples of ring compounds readily include cases where:
- all the atoms are carbon (i.e., are carbocycles),
- none of the atoms are carbon (inorganic cyclic compounds),[2] or where
- both carbon and non-carbon atoms are present (heterocyclic compounds).
Common atoms can (as a result of their valences) form varying numbers of bonds, and many common atoms readily form rings. In addition, depending on the ring size, the bond order of the individual links between ring atoms, and their arrangements within the rings, cyclic compounds may be aromatic or non-aromatic; in the case of non-aromatic cyclic compounds, they may vary from being fully saturated to having varying numbers of multiple bonds. As a consequence of the constitutional variability that is thermodynamically possible in cyclic structures, the number of possible cyclic structures, even of small size (e.g., <17 atoms) numbers in the many billions.[3]
Moreover, the closing of atoms into rings may lock particular functional group–substituted atoms into place, resulting in stereochemistry and chirality being associated with the compound, including some manifestations that are unique to rings (e.g., configurational isomers);[4] As well, depending on ring size, the three-dimensional shapes of particular cyclic structures—typically rings of 5-atoms and larger—can vary and interconvert such that conformational isomerism is displayed.[4]
Nomenclature
IUPAC nomenclature has extensive rules to comer the naming of cyclic structures, both as core structures, and as substituents appended to alicyclic structures. The term macrocycle is used when a ring-containing compound has a ring of 8 or more atoms.[5][6] The term polycyclic is used when more than one ring appears in a single molecule. Naphthalene is formally a polycyclic, but is more specifically named as a bicyclic compound. Several examples of macrocyclic and polycyclic structures are given in the final gallery below.
Carbocycles
The vast majority of cyclic compounds are organic, and of these, a significant proportion (and a conceptually important portion) are composed of rings made only of carbon atoms (i.e., they are carbocycles).
Inorganic cyclic compounds
Inorganic atoms form cyclic compounds as well. Examples include sulfur, silicon (e.g., in silanes), phosphorus (e.g., in phosphanes and phosphoric acid variants), and boron (e.g., in triboric acid). When carbon in benzene is "replaced" by other elements, e.g., as in borabenzene, silabenzene, germanabenzene, stannabenzene, and phosphorine, aromaticity is retained, and so aromatic inorganic cyclic compounds are known and well-characterized.
Heterocyclic compounds
Cyclic compounds that have both carbon and non-carbon atoms present are termed (heterocyclic compounds); alternatively the name can refer to inorganic cyclic compounds, such as siloxanes and borazines, that have more type of atom in their rings. Hantzsch–Widman nomenclature is recommended by the IUPAC for naming heterocycles, but many common names remain in regular use.
Aromaticity
Cyclic compounds may or may not exhibit aromaticity; benzene is an example of an aromatic cyclic compound, while cyclohexane is non-aromatic.In organic chemistry, the term aromaticity is used to describe a cyclic (ring-shaped), planar (flat) molecule that exhibits unusual stability as compared to other geometric or connective arrangements of the same set of atoms. As a result of their stability, it is very difficult to cause aromatic molecules to break apart and to react with other substances. Organic compounds that are not aromatic are classified as aliphatic compounds—they might be cyclic, but only aromatic rings have especial stability (low reactivity).
Since one of the most commonly encountered aromatic systems of compounds in organic chemistry is based on derivatives of the prototypical aromatic compound benzene (an aromatic hydrocarbon common in petroleum and its distillates), the word “aromatic” is occasionally used to refer informally to benzene derivatives, and this is how it was first defined. Nevertheless, many non-benzene aromatic compounds exist. In living organisms, for example, the most common aromatic rings are the double-ringed bases in RNA and DNA. A functional group or other substituent that is aromatic is called an aryl group.
The earliest use of the term “aromatic” was in an article by August Wilhelm Hofmann in 1855.[1] Hofmann used the term for a class of benzene compounds, many of which do have odors (aromas), unlike pure saturated hydrocarbons. Today, there is no general relationship between aromaticity as a chemical property and the olfactory properties of such compounds (how they smell), although in 1855, before the structure of benzene or organic compounds was understood, chemists like Hofmann were beginning to understand that odiferous molecules from plants, such as terpenes, had chemical properties we recognize today are similar to unsaturated petroleum hydrocarbons like benzene.
In terms of the electronic nature of the molecule, aromaticity describes a conjugated system often made of alternating single and double bonds in a ring. This configuration allows for the electrons in the molecule’s pi system to be delocalized around the ring, increasing the molecule's stability. The molecule cannot be represented by one structure, but rather a resonance hybrid of different structures, such as with the two resonance structures of benzene. These molecules cannot be found in either one of these representations, with the longer single bonds in one location and the shorter double bond in another (See Theory below). Rather, the molecule exhibits bond lengths in between those of single and double bonds. This commonly seen model of aromatic rings, namely the idea that benzene was formed from a six-membered carbon ring with alternating single and double bonds (cyclohexatriene), was developed by August Kekulé (see History section below). The model for benzene consists of two resonance forms, which corresponds to the double and single bonds superimposing to produce six one-and-a-half bonds. Benzene is a more stable molecule than would be expected without accounting for charge delocalization.
Simple, mono-cyclic examples
The following are examples of simple and aromatic carbocycles, inorganic cyclic compounds, and heterocycles:
- Simple mono-cyclic compounds: Carbocyclic, inorganic, and heterocyclic (aromatic and non-aromatic) examples.
-
Cycloheptane, a simple 7-membered carbocyclic compound, methylene hydrogens shown (non-aromatic).
-
Benzene, a 6-membered carbocyclic compound. methine hydrogens shown, and 6 electrons shown as delocalized through drawing of circle (aromatic).
-
Cyclo-octasulfur, an 8-membered inorganic cyclic compound (non-aromatic).
-
Pentazole, a 5-membered inorganic cyclic compound (aromatic).
-
Pyridine, a 6 membered heterocyclic compound, methine hydrogen atoms implied, not shown, and delocalized π-electrons shown as discrete bonds (aromatic).
Stereochemistry
The closing of atoms into rings may lock particular atoms with distinct substitution by functional groups such that the result is stereochemistry and chirality of the compound, including some manifestations that are unique to rings (e.g., configurational isomers).[4]
Conformational isomerism
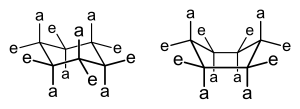
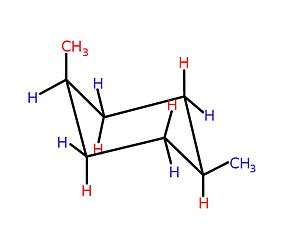
Depending on ring size, the three-dimensional shapes of particular cyclic structures—typically rings of 5-atoms and larger—can vary and interconvert such that conformational isomerism is displayed.[4] Indeed, the development of this important chemical concept arose, historically, in reference to cyclic compounds. For instance, cyclohexanes—six membered carbocycles with no double bonds, to which various substituents might be attached, see image—display an equilibrium between two conformations, the chair and the boat, as shown in the image.
The chair conformation is the favored configuration, because in this conformation, the steric strain, eclipsing strain, and angle strain that are otherwise possible are minimized.[4] Which of the possible chair conformations predominate in cyclohexanes bearing one or more substituents depends on the substiuents, and where they are located on the ring; generally, "bulky" substituents—those groups with large volumes, or groups that are otherwise repulsive in their interactions—prefer to occupy an equatorial location.[4] An example of interactions within a molecule that would lead to steric strain, leading to a shift in equilibrium from boat to chair, is the interaction between the two methyl groups in cis-1,4-dimethylcyclohexane. In this molecule, the two methyl groups are in opposing positions of the ring (1,4-), and their cis stereochemistry projects both of these groups toward the same side of the ring. Hence, if forced into the higher energy boat form, these methyl groups are in steric contact, repel one another, and drive the equilibrium toward the chair conformation.
Macrocycles

The term macrocycle is used for compounds having a rings of 8 or more atoms.[5][6] Macrocycles may be fully carbocyclic, heterocyclic but having limited heteroatoms (e.g., in lactones and lactams), or be rich in heteroatoms and displaying significant symmetry (e.g., in the case of chelating macrocycles). Macrocycles can access a number of stable conformations, with preference to reside in conformations that minimize transannular nonbonded interactions within the ring (e.g., with the chair and chair-boat being more stable than the boat-boat conformation for cyclooctane, because of the interactions depicted by the arcs shown). Medium rings (8-11 atoms) are the most strained, with between 9-13 (kcal/mol) strain energy, and analysis of factors important in the conformations of larger macrocycles can be modeled using medium ring conformations.[7] Conformational analysis of odd-membered rings suggests they tend to reside in less symmetrical forms with smaller energy differences between stable conformations.[8]

Principle uses of cyclic structures
Because of the unique shapes, reactivities, properties, and bioactivities that they engender, are the largest majority of all molecules involved in the biochemistry, structure, and function of living organisms, and in the man-made molecules (e.g., drugs, herbicides, etc.) through which man attempts to exert control over nature and biological systems.
Complex and polycyclic examples
The following are examples of cyclic compounds exhibiting more complex ring systems and stereochemical features:
- Complex cyclic compounds: Macrocyclic and polycyclic examples
-
Naphthalene, technically a polycyclic, more specifically a bicyclic compound, with circles showing delocalization of π-electrons (aromatic).
-
Decalin (decahydronaphthalene), the fully saturated derivative of naphthalene, showing the two stereochemistries possible for "fusing" the two rings together, and how this impacts the shapes available to this bicyclic compound (non-aromatic).
-
Longifolene, a terpene natural product, and an example of a tricyclic molecule (non-aromatic).
-
Paclitaxel, a polycyclic natural product with a tricyclic core: with a heterocyclic, 4-membered D ring, fused to further 6- and 8-membered carbocyclic (A/C and B) rings (non-aromatic), and with three further pendant phenyl-rings on its "tail", and attached to C-2 (abbrev. Ph, C6H5; aromatics).
-
A representative three-dimensional shape adopted by paclitaxel, as a result of its unique cyclic structure.[1]
-
Cholesterol, another terpene natural product, in particular, a steroid, a class of tetracyclic molecules (non-aromatic).
-
Benzo[a]pyrene, a pentacyclic compound both natural and man-made, and delocalized π-electrons shown as discrete bonds (aromatic).
-
Pagodane, a complex, highly symmetric, man-made polycyclic compound (non-aromatic).
-
Brevetoxin A, a natural product with ten rings, all fused, and all heterocyclic, and a toxic component associated with the organisms responsible for red tides. The R group at right refers to one of several possible four-carbon side chains (see main Brevetoxin article; non-aromatic).
- ^ J. Löwe, H. Li, K.H. Downing & E. Nogales, 2001, "Refined structure of alpha beta-tubulin at 3.5 A resolution," J. Mol. Biol. 313:1045-1057, see [1], accessed 7 April 2015.
Synthetic reactions altering rings
Important general reactions for forming rings

There are a variety of specialized reactions whose use is solely the formation of rings, and these will be discussed below. In addition to those, there are a wide variety of general organic reactions that historically have been crucial in the development, first, of understanding the concepts of ring chemistry, and second, of reliable procedures for preparing ring structures in high yield, and with defined orientation of ring substituents (i.e., defined stereochemistry). These general reactions include:
- Acyloin condensation;
- Anodic oxidations; and
- the Dieckmann condensation as applied to ring formation.
Ring-closing reactions
In organic chemistry, a variety of synthetic procures are particularly useful in closing carbocyclic and other rings; these are termed ring-closing reactions. Examples include:
- alkyne trimerisation;
- the Bergman cyclization;
- the Diels-Alder and other cycloaddition reactions;
- the Nazarov cyclization reaction;
- various radical cyclizations;
- ring-closing metathesis reactions, which can be also be used to accomplish a specific type of polymerization;
- the Ruzicka large ring synthesis; and
- the Wenker synthesis of aziridines.
Ring-opening reactions
A variety of further synthetic procedures are particularly useful in opening carbocyclic and other rings, generally which contain a double bound or other functional group "handle" to facilitate chemistry; these are termed ring-opening reactions. Examples include:
- ring opening metathesis, which can be also be used to accomplish a specific type of polymerization.
Ring expansion and ring contraction reactions
Further reading
- Jürgen-Hinrich Fuhrhop & Gustav Penzlin, 1986, "Organic synthesis: concepts, methods, starting materials," Weinheim, BW, DEU:VCH, ISBN 0895732467, see , accessed 19 June 2015.
- Michael B. Smith & Jerry March, 2007, "March's Advanced Organic Chemistry: Reactions, Mechanisms, and Structure," 6th Ed., New York, NY, USA:Wiley & Sons, ISBN 0470084944, see , accessed 19 June 2015.
- Francis A. Carey & Richard J. Sundberg, 2006, "Title Advanced Organic Chemistry: Part A: Structure and Mechanisms," 4th Edn., New York, NY, USA:Springer Science & Business Media, ISBN 0306468565, see , accessed 19 June 2015.
- Michael B. Smith, 2011, "Organic Chemistry: An Acid—Base Approach," Boca Raton, FL, USA:CRC Press, ISBN 1420079212, see , accessed 19 June 2015. [May not be most necessary material for this article, but significant content here is available online.]
- Jonathan Clayden, Nick Greeves & Stuart Warren, 2012, "Organic Chemistry," Oxford, Oxon, GBR:Oxford University Press, ISBN 0199270295, see , accessed 19 June 2015.
- László Kürti & Barbara Czakó, 2005, "Strategic Applications of Named Reactions in Organic Synthesis: Background and Detailed Mechanisms, Amsterdam, NH, NLD:Elsevier Academic Press, 2005ISBN 0124297854, see , accessed 19 June 2015.
See also
External links
- Polycyclic Compounds at the US National Library of Medicine Medical Subject Headings (MeSH)
- Macrocyclic Compounds at the US National Library of Medicine Medical Subject Headings (MeSH)
References
- ↑ March, Jerry (1985), Advanced Organic Chemistry: Reactions, Mechanisms, and Structure (3rd ed.), New York: Wiley, ISBN 0-471-85472-7.
- ↑ I. Halduc, 1961, "Classification of inorganic cyclic compounds," J. Struct. Chem. 2(3):350-358, see , accessed 8 April 2015.
- ↑ Jean-Louis Reymond, 2015, "The Chemical Space Project," Acc. Chem. Res., 48(3), 722-730, DOI 10.1021/ar500432k, see , accessed 7 April 2015.
- 1 2 3 4 5 6 7 William Reusch, 2010, "Stereoisomers Part I," In Virtual Textbook of Organic Chemistry, Michigan State University, see , accessed 7 April 2015.
- 1 2 Still, W. C.; Galynker, I. "Chemical consequences of conformation in macrocyclic compounds: An effective approach to remote asymmetric induction," Tetrahedron 1981, 37, 3981-3996, see , accessed 19 June 2015.
- 1 2 J. D. Dunitz. Perspectives in Structural Chemistry (Edited by J. D. Dunitz and J. A. Ibers), Vol. 2, pp. l-70; Wiley, New York (1968)
- ↑ Eliel, E.L., Wilen, S.H. and Mander, L.S. (1994) Stereochemistry of Organic Compounds, John Wiley and Sons, Inc., New York.
- ↑ Anet, F.A.L.; St. Jacques, M.; Henrichs, P.M.; Cheng, A.K.; Krane, J.; Wong, L. "Conformational analysis of medium-ring ketones," Tetrahedron 1974, 30, 1629-1637, see [www.sciencedirect.com/science/article/pii/S0040402001906854], accessed 19 June 2015.
|