Renin inhibitor
Renin inhibitor | |
---|---|
Drug class | |
![]() | |
Class identifiers | |
Use | Hypertension |
ATC code | C09XA |
Biological target | Renin |
Clinical data | |
AHFS/Drugs.com | Drug Classes |
Renin inhibitors are a group of pharmaceutical drugs used primarily in treatment of essential hypertension (high blood pressure).
These drugs inhibit the first and rate-limiting step of the renin–angiotensin–aldosterone system (RAAS), namely the conversion of angiotensinogen to angiotensin I.This leads to a totality in absence of Angiotensin II based on the rationale that renin only acts to inhibit this step unlike Angiotensin Converting Enzyme which is also involved in other biochemical reactions. Since the 1970s, scientists have been trying to develop potent inhibitors with acceptable oral bioavailability.[2][3] The process was difficult and took about three decades. The first and second generations faced problems such as poor bioavailability and lack of potency. Finally, the third generation was discovered. These compounds were nonpeptidic renin inhibitors, had acceptable oral bioavailability and were potent enough for clinical use. The first drug in this class was aliskiren, which received a marketing approval in 2007.[2] As of January 2012, it is the only renin inhibitor on the market.
History
In 1896, the Finnish physiologist Robert Tigerstedt and the Swedish physician Per Bergman did an experiment on kidneys and the circulatory system in rabbits. They observed that blood pressure rose in the rabbits when extracts of the kidneys were injected into their jugular veins.[4][5] They also discovered this substance responsible for higher blood pressure was produced in the renal cortex, and they named it renin.[5] Although this experiment laid the foundation for future investigations into the RAAS pathway, it had little impact on the scientific community at that time.[4][6] In 1934, when Goldblatt published his work in renal ischaemia, renin came into focus again. The importance of renin in the pathogenesis of cardiovascular disease was, however, not fully understood until in the 1970s, and 20 years later the first renin inhibitors went to clinical trials.[2]
Pepstatin, which was described in 1972, was the first synthetic renin inhibitor, but poor pharmacokinetic properties prevented it from entering in vivo investigations.[3][7] The first generation of renin inhibitors, such as H-142, were peptide analogues of angiotensinogen.[8] However, these inhibitors had also limited drug-like properties.[2][7] Hopes of breakthrough appeared in 1982 when development of the second generation renin inhibitors began.[2] This generation consisted of peptide-like compounds, such as remikiren, enalkiren and zanikiren.[6] They had more drug-like rather than substrate-like properties, and in 1990 they went to clinical trials. The second generation had its limitations and never completed clinical trials.[2]
Aliskiren, the only renin inhibitor to go into phase III clinical trials, is not structurally related to peptides, which makes it a third-generation renin inhibitor.[2][9] The first clinical trial was performed in 2000 in healthy volunteers.[10] In 2007, aliskiren was approved by the US Food and Drug Administration and the European Medicines Agency as a treatment for hypertension.[2] A systematic review by the Cochrane Hypertension group found the maximum recommended dose of aliskiren produced an appreciable decline in blood pressure over placebo.[11]
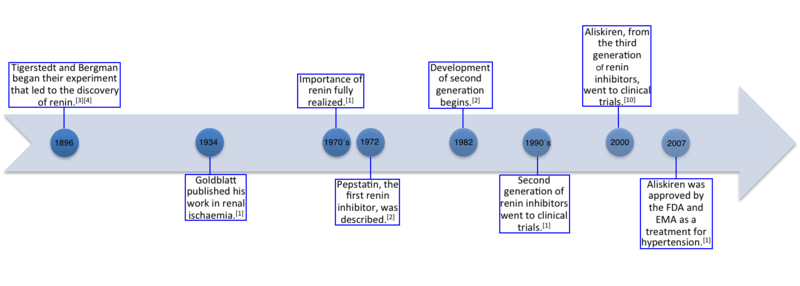
The renin–angiotensin–aldosterone system

The renin–angiotensin–aldosterone system (RAAS) plays a key role in the pathology of cardiovascular disease, hypertension, diabetic kidney disease and heart failure.[12] Under normal conditions, stimulation of the RAAS occurs in response to threats that compromise blood pressure stability, such as hypotension, blood loss and excessive loss of sodium and water. Blood pressure depends on total peripheral resistance and cardiac output.
The highly selective aspartic protease renin is secreted from the juxtaglomerular apparatus, which is the only source of active renin,[13] although its precursor, prorenin, can be secreted by other tissues, such as the salivary glands, brain, heart and blood vessels.[13][14][15] Renin is a circulating enzyme that acts on a circulating peptide, angiotensinogen.[16] Renin cleaves the peptide at the Leu10–Val11 bond, and this reaction is the rate-determining step of the RAAS.[17] This leads to the product angiotensin I (Ang I) which is a decapeptide. Ang I is broken down by the angiotensin-converting enzyme (ACE) to the active octapeptide angiotensin II (Ang II), which is the principal effector of the RAAS.[16] Ang II stimulates renal sodium retention; promotes aldosterone secretion; causes vasoconstriction, and increases sympathetic nervous system activity.[15][18] Ang II also provides a negative feedback to the system by inhibiting renin release by the juxtaglomerular apparatus.[18] Ang II interacts with at least two classes of Ang II receptors, AT1 and AT2.[15] This mechanism, which runs from renin through Ang II and to aldosterone, as well as the negative feedback that Ang II has on renin secretion, is known as RAAS.[18] The net effect is to increase blood pressure, which in normal physiology is necessary in order to maintain homeostasis.
It is suspected that essential hypertension, a heterogeneous disorder whose long-term effects can be end organ damage, can involve at least in some cases an overactivity of this system, which several types of medications attempt to counter.[16] Renin concentration in blood plasma tends to be higher in younger people with hypertension when vasoconstriction may be the main reason for high blood pressure. Conversely, renin is lower in older people or in people of African American or African Caribbean ethnicity when salt retention may contribute more to elevated blood pressure.[16] However, the role of plasma renin levels in the etiology and management of hypertension is disputed.[19]
Mechanism of action
Renin inhibitors bind to the active site of renin and inhibit the binding of renin to angiotensinogen, which is the rate-determining step of the RAAS cascade.[16] Consequently, renin inhibitors prevent the formation of Ang I and Ang II. Renin inhibitors may also prevent Ang-(1-7), Ang-(1-9) and Ang-(1-5) formation,[20] although it is not known if this is clinically important. Renin is highly selective for its only naturally occurring substrate which is angiotensinogen, and the incidence of unwanted side effects with a renin inhibitor is infrequent.[21] and similar to angiotensin II receptor antagonists.[22] Ang II also functions within the RAAS as a negative feedback to suppress further release of renin. A reduction in Ang II levels or blockade of angiotensin receptors will suppress the feedback loop and lead to increased plasma renin concentrations (PRC) and plasma renin activity (PRA). This can be problematic for ACE inhibitor and angiotensin II receptor antagonist therapy since increased PRA could partially overcome the pharmacologic inhibition of the RAAS cascade. Because renin inhibitors directly affect renin activity, decrease of PRA despite the increased PRC (from loss of the negative feedback) may be clinically advantageous.[23]
Drug discovery and development
Pepstatin – the first renin inhibitor
Pepstatin was the first synthetic renin inhibitor. It is of microbial origin and is an N-acyl-pentapeptide, more accurately: isovaleryl-L-valyl-L-valyl-statyl-L-alanyl-statine.[3][24] Pepstatin was found to be a potent competitive inhibitor of most aspartic proteases, but a weak inhibitor of renin.[25] Originally, it was thought to be effective in the treatment of duodenal ulcers, and went through clinical trials, but had no success.[26][27] Statine, an amino acid, is thought to be responsible for the inhibitory activity of pepstatin, because it mimics the tetrahedral transition state of the peptide catalysis.[28] Because of hydrophobic properties of statine, pepstatin has very low solubility in physiological media.[29] Since it had low potency and poor solubility, it did not enter in vivo studies.
First generation: peptide analogues
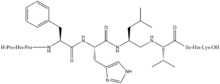
This generation consists of two groups of compounds, either peptide analogues of the prosegment of renin[30] or peptide analogues of the amino-terminal part of the substrate angiotensinogen.[8][31][32] The drugs in the latter group seemed to be effective in inhibiting renin activity and lowering blood pressure in both animals and humans.[33] Unfortunately, they had to be given parenterally because of poor bioavailability. They also turned out to have short durations of action, low potencies and their ability to lower blood pressure was inadequate. None of these drugs completed clinical investigations.[23]
Second generation: peptide mimetics
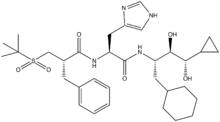
Compounds in this generation were more potent, more stable and had longer durations of action. One of these, CGP2928, a peptidomimetic compound, was the first renin inhibitor proven effective when taken orally. Tested on marmosets, it was only active at high doses.[7] Development of new drugs in the second generation continued to improve pharmacokinetic properties. Remikiren, enalkiren and zankiren were then discovered. These were peptidomimetic inhibitors with improved structures that made them more specific, potent and stable. Unfortunately, clinical development was terminated because the drugs had poor oral bioavailability (poorly absorbed and rapidly metabolized) and lowering blood pressure activity still remained low.[2][17][23]
Third generation: non-peptides
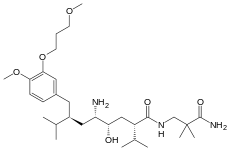
Aliskiren, an orally active non-peptide renin inhibitor, was the first drug in its class on the market. It is used to treat hypertension as monotherapy or in combination with other antihypertensive agents.[2] The key to the discovery of aliskiren was crystallography and molecular modeling techniques. Now, a solution has been found to the problem that impeded the development of the renin inhibitors of the previous generations. Non-peptide substances were known to be able to solve the problems of poor pharmacokinetic properties and low specificity. This led to the design of small molecules, non-peptide inhibitors, which were very potent and specific of human renin.[17][34]
However, caused by their chemical structure even third-generation renin inhibitors are difficult to resorb by the human body and their oral bioavailability is often below 2%.
Binding and structure activity relationship of renin inhibitors
The renin molecule is a monospecific enzyme that belongs to the aspartic protease family.[35] Its structure is complex and consists of two homologous lobes that fold mainly in a β-sheet conformation.[17] Between the two lobes, deep within the enzyme, resides the active site, and its catalytic activity is due to two aspartic acid residues (Asp32 and Asp 215, one from each lobe in the renin molecule).[36] A flexible flap made from amino acids formed in a β-hairpin closes the active site by covering the cleft.[37] The renin molecule contains both hydrophobic and hydrophilic amino acids. The hydrophilic ones tend to be on the outside of the molecule, while the hydrophobic ones tend to be more on the inside and form the active site, a large hydrophobic cavity[38] that can accommodate a ligand with at least seven residues. The principal connection between a ligand and the enzyme is by hydrogen bonding. The residues are named after their places in the ligand, the residues closest to the cleavage site are named P1 and P1′ and they bind into the S1 and S1′ pockets, respectively. There are four S pockets, and three S′ pockets (table 1). The pockets alternate on either side of the backbone in the ligand. This alternation affects the orientation of the pockets, making the S3 and S1 pockets arrange together and the S2 pocket close to both S4 and S1′ pockets.[37] Evidence suggests the closely arranged S1 and S3 pockets merge to form a spacious superpocket.[39] Ligands that fill the superpocket have greater potency than those which do not, occupying increases potency 200-fold. These ligands can be structurally diverse and form van der Waals bonds to the surface of the superpocket.[6] From the S3 pocket stretches a binding site distinct for renin, the S3sp subpocket.[35] The S3sp subpocket can accommodate both hydrophobic and polar residues, the pocket can accommodate three water molecules, but has also lipophilic nature. The S3sp subpocket is not conformationally flexible, so the residues occupying the pocket must have certain characteristics. They can not be sterically demanding and must have reasonably high number of rotatable bonds and be able to connect with hydrogen bonds. The S2 pocket is large, bipartite and hydrophobic, but can accommodate both hydrophobic and polar ligands. This diversity of possible polarity offers the P2 residue opportunity of variation in its connection to the enzyme. The S3-S1 and the S3sp subpockets have been the main target of drug design, but recent discoveries have indicated other sites of interest. Interactions to the pockets on the S′ site have been proven to be critical for affinity, especially the S1′ and S2′, and in vitro tests have indicated the interaction with the flap region could be important to affinity.[6]
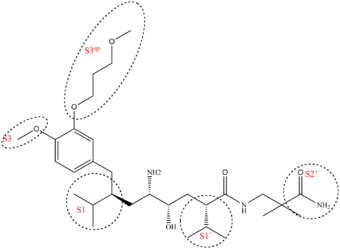
Characteristics[6] | Subsite | Importance to binding[6][40] | |
---|---|---|---|
S4 | Hydrophobic | P4 | Relatively important for binding |
S3 | Hydrophobic | P3 | Very important for binding |
S3sp | Equally hydrophobic/-philic | P3 side chain | Dramatically enhances binding affinity |
S2 | Large and hydrophobic | P2 | Important for binding |
S1 | Large and hydrophobic | P1 | NA |
S1′ | Primarily hydrophobic | P1′ | Critical for tight binding |
S2′ | Polar | P2′ | Critical for tight binding |
S3′ | NA | P3′ | Structure and presence is not as important |
Interaction with both aspartic acids in the active site results in a higher affinity. Higher affinity also results by occupying more active site pockets. However, some pockets contribute more to the affinity than others. A hydrophobic interaction with the S3sp subpocket, S1 and S3 contribute to higher potency and affinity.[41] By having a large and aromatic residue in P3 increases inhibitory activity.[42] Occupation of the S3sp subpocket can increase potency by 50-fold and results in tight binding.[6]
Example of binding to the renin inhibitor: Aliskiren is a peptide-like renin inhibitor and, unlike most, it is rather hydrophilic. It blocks the catalytic function of the enzyme by occupying the S3 to S2′ pockets, except the S2 pocket. Aliskiren also binds to the S3sp subpocket and because that pocket is distinct for renin, aliskiren does not inhibit other aspartic proteases, such as cathepsin D and pepsin.[40] The side chain of aliskiren binds the S3sp subpocket ideally, and leads to its quality as an inhibitor of human renin.[6] The hydroxyl group in aliskiren forms a hydrogen bond with both oxygen atoms of the Asp32. The amine group forms a hydrogen bond with the carboxylic acid group of Gly217 and the oxygen atom of the Asp32. The methoxy group on the aromatic ring fills the S3 pocket and may possibly form a hydrogen bond with a secondary amine group of Tyr14. The amide group forms a hydrogen bond with a secondary amine group of Ser76.[41] The S1 and S1′ pockets are occupied by the two propyl groups in positions P1 and P1′.[39] The terminal amide in position P2′ anchors the amide tail in the active site by forming a hydrogen bond with Arg74 in the S2′ pocket.[43]
Current status
Aliskiren is effective in lowering blood pressure,[2][23] but as of 20 April 2012 the US Food and Drug Administration (FDA) issued a warning of possible risks when using aliskiren or blood pressure medicines containing aliskiren with ACE inhibitors and angiotensin receptor blockers (ARBs) in patients with diabetes or kidney (renal) impairment. They advised that such drug combinations should not be used in patients with diabetes because of the risk of causing renal impairment, hypotension, and hyperkalemia and that aliskiren should not be used with ARBs or ACE inhibitors in patients with moderate to severe renal impairment (i.e., where glomerular filtration rate [GFR] < 60 mL/min). However, they also recommend that patients should not stop taking aliskiren without talking to a healthcare professional.[44]
Aliskiren in combination with hydrochlorothiazide was approved by the FDA in 2008 under the tradename Tekturna HCT.[45][46]
In 2007, Actelion/Merck and Speedel companies announced they had the next generation of renin inhibitors in clinical research. The lead compound from Actelion/Merck has entered phase II trials. One compound from Speedel, SPP635, has completed phase IIa. The results showed it was safe and well-tolerated over a four-week period, and it reduced blood pressure by 9,8 to 17,9 mmHg. In 2008, SPP635 was continuing phase II development for hypertension in diabetic patients. More renin inhibitors from Speedel are in clinical trials. Two of them, SPP1148 and SPP676, have entered phase I. Other are in preclinical phases, the compound SPP1234 and compounds from the SPP800 series.[45]
The next generation of renin inhibitors have shown potential improvements over previous generations where bioavailability has increased up to 30% in humans, and they have better tissue distribution.[45]
See also
- ACE inhibitor
- ACE inhibitors drug design
- Angiotensin
- Angiotensin II receptor antagonist
- Beta blocker
- Circulatory system
- Discovery and development of angiotensin receptor blockers
References
- ↑ Gradman AH, Schmieder RE, Lins RL, Nussberger J, Chiang Y, Bedigian MP (March 2005). "Aliskiren, a novel orally effective renin inhibitor, provides dose-dependent antihypertensive efficacy and placebo-like tolerability in hypertensive patients". Circulation 111 (8): 1012–8. doi:10.1161/01.CIR.0000156466.02908.ED. PMID 15723979.
- 1 2 3 4 5 6 7 8 9 10 11 Jensen, C.; Herold, P.; Brunner, H. R. (2008). "Aliskiren: The first renin inhibitor for clinical treatment". Nature Reviews Drug Discovery 7 (5): 399–410. doi:10.1038/nrd2550. PMID 18340340.
- 1 2 3 Gross, F.; Lazar, J.; Orth, H. (1972). "Inhibition of the renin-angiotensinogen reaction by pepstatin". Science 175 (22): 656. doi:10.1126/science.175.4022.656. PMID 4109853.
- 1 2 Ferrario, C. M.; Iyer, S. N. (1998). "Angiotensin-(1-7): A bioactive fragment of the renin-angiotensin system". Regulatory peptides 78 (1–3): 13–18. doi:10.1016/s0167-0115(98)00134-7. PMID 9879742.
- 1 2 Phillips, M. I.; Schmidt-Ott, K. M. (1999). "The Discovery of Renin 100 Years Ago". News in physiological sciences : an international journal of physiology produced jointly by the International Union of Physiological Sciences and the American Physiological Society 14: 271–274. PMID 11390864.
- 1 2 3 4 5 6 7 8 Webb, R. L.; Schiering, N.; Sedrani, R.; Maibaum, J. R. (2010). "Direct Renin Inhibitors as a New Therapy for Hypertension". Journal of Medicinal Chemistry 53 (21): 7490–7520. doi:10.1021/jm901885s. PMID 20731374.
- 1 2 3 Wood, J. M.; Gulati, N.; Forgiarini, P.; Fuhrer, W.; Hofbauer, K. G. (1985). "Effects of a specific and long-acting renin inhibitor in the marmoset". Hypertension 7 (5): 797–803. doi:10.1161/01.hyp.7.5.797. PMID 3928488.
- 1 2 Szelke, M.; Leckie, B.; Hallett, A.; Jones, D. M.; Sueiras, J.; Atrash, B.; Lever, A. F. (1982). "Potent new inhibitors of human renin". Nature 299 (5883): 555–557. doi:10.1038/299555a0. PMID 6750410.
- ↑ Segall, L.; Covic, A.; Goldsmith, D. J. A. (2007). "Direct renin inhibitors: The dawn of a new era, or just a variation on a theme?". Nephrology Dialysis Transplantation 22 (9): 2435–2439. doi:10.1093/ndt/gfm363. PMID 17556409.
- ↑ Nussberger, J.; Wuerzner, G.; Jensen, C.; Brunner, H. R. (2002). "Angiotensin II suppression in humans by the orally active renin inhibitor Aliskiren (SPP100): Comparison with enalapril". Hypertension 39 (1): E1–E8. doi:10.1161/hy0102.102293. PMID 11799102.
- ↑ Musini, VM; Fortin, PM; Bassett, K; Wright, JM (2008). "Blood pressure lowering efficacy of renin inhibitors for primary hypertension". Cochrane Database of Systematic Reviews 4: CD007066. doi:10.1002/14651858.CD007066.pub2.
- ↑ Weir MR (September 2007). "Effects of renin-angiotensin system inhibition on end-organ protection: can we do better?". Clin Ther 29 (9): 1803–24. doi:10.1016/j.clinthera.2007.09.019. PMID 18035185.
- 1 2 Castrop H, Höcherl K, Kurtz A, Schweda F, Todorov V, Wagner C (April 2010). "Physiology of kidney renin". Physiol. Rev. 90 (2): 607–73. doi:10.1152/physrev.00011.2009. PMID 20393195.
- ↑ Tice, C. M.; Xu, Z.; Yuan, J.; Simpson, R. D.; Cacatian, S. T.; Flaherty, P. T.; Zhao, W.; Guo, J.; Ishchenko, A.; Singh, S. B.; Wu, Z.; Scott, B. B.; Bukhtiyarov, Y.; Berbaum, J.; Mason, J.; Panemangalore, R.; Cappiello, M. G.; Müller, D.; Harrison, R. K.; McGeehan, G. M.; Dillard, L. W.; Baldwin, J. J.; Claremon, D. A. (2009). "Design and optimization of renin inhibitors: Orally bioavailable alkyl amines". Bioorganic & Medicinal Chemistry Letters 19 (13): 3541–3545. doi:10.1016/j.bmcl.2009.04.140. PMID 19457666.
- 1 2 3 Ferrario, C. M. (2006). "Role of angiotensin II in cardiovascular disease therapeutic implications of more than a century of research". Journal of the renin-angiotensin-aldosterone system : JRAAS 7 (1): 3–14. doi:10.3317/jraas.2006.003. PMID 17083068.
- 1 2 3 4 5 Brown, M. J. (2006). "Direct renin inhibition — a new way of targeting the renin system". Journal of Renin-Angiotensin-Aldosterone System 7 (2 suppl): S7–S11.
- 1 2 3 4 Rahuel, J.; Rasetti, V.; Maibaum, J.; Rüeger, H.; Göschke, R.; Cohen, N. C.; Stutz, S.; Cumin, F.; Fuhrer, W.; Wood, J. M.; Grütter, M. G. (2000). "Structure-based drug design: The discovery of novel nonpeptide orally active inhibitors of human renin". Chemistry & Biology 7 (7): 493–504. doi:10.1016/S1074-5521(00)00134-4. PMID 10903938.
- 1 2 3 Hsueh, W. A.; Wyne, K. (2011). "Renin-Angiotensin-Aldosterone System in Diabetes and Hypertension". The Journal of Clinical Hypertension 13 (4): 224–237. doi:10.1111/j.1751-7176.2011.00449.x. PMID 21466617.
- ↑ Moser M, Izzo JL (2003). "Plasma renin measurement in the management of hypertension: the V and R hypothesis". J Clin Hypertens (Greenwich) 5 (6): 373–6. doi:10.1111/j.1524-6175.2003.02870.x. PMID 14688491.
- ↑ Müller, D. N.; Derer, W.; Dechend, R. (2008). "Aliskiren—mode of action and preclinical data". Journal of Molecular Medicine 86 (6): 659–662. doi:10.1007/s00109-008-0330-6. PMID 18443751.
- ↑ Weir, M.; Bush, C.; Anderson, D.; Zhang, J.; Keefe, D.; Satlin, A. (2007). "Antihypertensive efficacy, safety, and tolerability of the oral direct renin inhibitor aliskiren in patients with hypertension: A pooled analysis". Journal of the American Society of Hypertension 1 (4): 264–277. doi:10.1016/j.jash.2007.04.004. PMID 20409858.
- ↑ Gao D, Ning N, Niu X, Wei J, Sun P, Hao G (May 2011). "Aliskiren vs. angiotensin receptor blockers in hypertension: meta-analysis of randomized controlled trials". Am. J. Hypertens. 24 (5): 613–21. doi:10.1038/ajh.2011.3. PMID 21293386.
- 1 2 3 4 Staessen, J. A.; Li, Y.; Richart, T. (2006). "Oral renin inhibitors". The Lancet 368 (9545): 1449–1456. doi:10.1016/S0140-6736(06)69442-7. PMID 17055947.
- ↑ Umezawa, H.; Aoyagi, T.; Morishima, H.; Matsuzaki, M.; Hamada, M.; Takeuchi, T. (1970). "Pepstatin, a new pepsin inhibitor produced by Actinomycetes". The Journal of antibiotics 23 (5): 259–262. doi:10.7164/antibiotics.23.259. PMID 4912600.
- ↑ Fisher, N. D. L.; Hollenberg, N. K. (2005). "Renin Inhibition: What Are the Therapeutic Opportunities?". Journal of the American Society of Nephrology 16 (3): 592–599. doi:10.1681/ASN.2004100874. PMID 15703270.
- ↑ Bonnevie, O.; Svendsen, L. B.; Holst-Christensen, J.; Johansen, T. S.; Søltoft, J.; Christiansen, P. M. (1979). "Double-blind randomised clinical trial of a pepsin-inhibitory pentapeptide (pepstatin) in the treatment of duodenal ulcer". Gut 20 (7): 624–628. doi:10.1136/gut.20.7.624. PMC 1412504. PMID 385457.
- ↑ Svendsen, L. B.; Christiansen, P. M.; Bonnevie, O. (1979). "Gastric ulcer therapy with a pepsin-inactivating peptide, pepstatin: A double-blind randomized clinical trial". Scandinavian journal of gastroenterology 14 (8): 929–932. PMID 394302.
- ↑ Marciniszyn Jr, J.; Hartsuck, J. A.; Tang, J. (1976). "Mode of inhibition of acid proteases by pepstatin". The Journal of Biological Chemistry 251 (22): 7088–7094. PMID 993206.
- ↑ Eid, M.; Evin, G.; Castro, B.; Menard, J.; Corvol, P. (1981). "New renin inhibitors homologous with pepstatin". The Biochemical Journal 197 (2): 465–471. doi:10.1042/bj1970465. PMC 1163147. PMID 7034718.
- ↑ Cumin, F.; Evin, G.; Fehrentz, J. A.; Seyer, R.; Castro, B.; Menard, J.; et al. (1985). "Inhibition of human renin by synthetic peptides derived from its prosegment". J Biol Chem 260 (16): 9154–9157.
- ↑ Szelke, M.; Leckie, B. J.; Tree, M.; Brown, A.; Grant, J.; Hallett, A.; et al. (1982). "H-77: a potent new renin inhibitor. In vitro and in vivo studies". Hypertension 4 (3 Pt 2): 59–69.
- ↑ Tree, M.; Atrash, B.; Donovan, B.; Gamble, J.; Hallett, A.; Hughes, M.; Jones, D. M.; Leckie, B.; Lever, A. F.; Morton, J. J.; Szelke, M. (1983). "New inhibitors of human renin tested in vitro and in vivo in the anaesthetized baboon". Journal of hypertension 1 (4): 399–403. doi:10.1097/00004872-198312000-00013. PMID 6398331.
- ↑ Webb, D. J.; Manhem, P. J.; Ball, S. G.; Inglis, G.; Leckie, B. J.; Lever, A. F.; Morton, J. J.; Robertson, J. I.; Murray, G. D.; Ménard, J.; Hallett, A.; Jones, D. M.; Szelke, M. (1985). "A study of the renin inhibitor H142 in man". Journal of hypertension 3 (6): 653–658. doi:10.1097/00004872-198512000-00013. PMID 3910726.
- ↑ Claude Cohen, N. (2007). "Structure-Based Drug Design and the Discovery of Aliskiren (Tekturna): Perseverance and Creativity to Overcome a R&D Pipeline Challenge". Chemical Biology & Drug Design 70 (6): 557–565. doi:10.1111/j.1747-0285.2007.00599.x. PMID 17999663.
- 1 2 Winiecka, I.; Dudkiewicz-Wilczyńska, J.; Roman, I.; Paruszewski, R. (2010). "New potential renin inhibitors with dipeptide replacements in the molecule". Acta poloniae pharmaceutica 67 (4): 367–374. PMID 20635532.
- ↑ Gradman, A. H.; Kad, R. (2008). "Renin inhibition in hypertension. [Review]". J Am Coll Cardiol 51 (5): 519–528.
- 1 2 Lunney, E. A.; Hamilton, H. W.; Hodges, J. C.; Kaltenbronn, J. S.; Repine, J. T.; Badasso, M.; Cooper, J. B.; Dealwis, C.; Wallace, B. A.; Lowther, W. T. (1993). "Analyses of ligand binding in five endothiapepsin crystal complexes and their use in the design and evaluation of novel renin inhibitors". Journal of Medical Chemistry 36 (24): 3809–3820. doi:10.1021/jm00076a008. PMID 8254610.
- ↑ Matter, H.; Scheiper, B.; Steinhagen, H.; Böcskei, Z.; Fleury, V. R.; McCort, G. (2011). "Structure-based design and optimization of potent renin inhibitors on 5- or 7-azaindole-scaffolds". Bioorganic & Medicinal Chemistry Letters 21 (18): 5487–5492. doi:10.1016/j.bmcl.2011.06.112. PMID 21840215.
- 1 2 Yuan, J.; Simpson, R. D.; Zhao, W.; Tice, C. M.; Xu, Z.; Cacatian, S.; Jia, L.; Flaherty, P. T.; Guo, J.; Ishchenko, A.; Wu, Z.; McKeever, B. M.; Scott, B. B.; Bukhtiyarov, Y.; Berbaum, J.; Panemangalore, R.; Bentley, R.; Doe, C. P.; Harrison, R. K.; McGeehan, G. M.; Singh, S. B.; Dillard, L. W.; Baldwin, J. J.; Claremon, D. A. (2011). "Biphenyl/diphenyl ether renin inhibitors: Filling the S1 pocket of renin via the S3 pocket". Bioorganic & Medicinal Chemistry Letters 21 (16): 4836–4843. doi:10.1016/j.bmcl.2011.06.043. PMID 21741239.
- 1 2 Wood, J. M.; Maibaum, J.; Rahuel, J.; Grütter, M. G.; Cohen, N. C.; Rasetti, V.; Rüger, H.; Göschke, R.; Stutz, S.; Fuhrer, W.; Schilling, W.; Rigollier, P.; Yamaguchi, Y.; Cumin, F.; Baum, H. P.; Schnell, C. R.; Herold, P.; Mah, R.; Jensen, C.; O'Brien, E.; Stanton, A.; Bedigian, M. P. (2003). "Structure-based design of aliskiren, a novel orally effective renin inhibitor". Biochemical and Biophysical Research Communications 308 (4): 698–705. doi:10.1016/S0006-291X(03)01451-7. PMID 12927775.
- 1 2 Politi, A.; Durdagi, S.; Moutevelis-Minakakis, P.; Kokotos, G.; Mavromoustakos, T. (2010). "Development of accurate binding affinity predictions of novel renin inhibitors through molecular docking studies". Journal of Molecular Graphics and Modelling 29 (3): 425–435. doi:10.1016/j.jmgm.2010.08.003. PMID 20855222.
- ↑ Akahane, K.; Umeyama, H.; Nakagawa, S.; Moriguchi, I.; Hirose, S.; Iizuka, K.; Murakami, K. (1985). "Three-dimensional structure of human renin". Hypertension 7 (1): 3–12. doi:10.1161/01.hyp.7.1.3. PMID 3884499.
- ↑ Wu, Y.; Shi, C.; Sun, X.; Wu, X.; Sun, H. (2011). "Synthesis, biological evaluation and docking studies of octane-carboxamide based renin inhibitors with extended segments toward S3′ site of renin". Bioorganic & Medicinal Chemistry 19 (14): 4238–4249. doi:10.1016/j.bmc.2011.05.059. PMID 21708467.
- ↑ http://www.fda.gov/Safety/MedWatch/SafetyInformation/SafetyAlertsforHumanMedicalProducts/ucm301120.htm
- 1 2 3 Speedel Acquiring an additional 51.7% stake and announcing plans for mandatory public tender offer.TRANSACTION OVERVIEW. (2008). From Novartis: http://www.novartis.com/downloads/investors/presentations-events/other-events/2008/2008-07_speedel-backgrounder.pdf
- ↑ Tekturna HCT (aliskiren; hydrochlorothiazide) tablets. (2011). From US Food and Drug Administration: http://www.accessdata.fda.gov/drugsatfda_docs/label/2011/022107s009lbl.pdf
External links
![]() |
Wikimedia Commons has media related to renin inhibitors. |
- Renin inhibitor aliskiren leads to dose-dependent blood pressure reductions - medicalnewstoday.com.
|
|