Printed electronics

Printed electronics is a set of printing methods used to create electrical devices on various substrates. Printing typically uses common printing equipment suitable for defining patterns on material, such as screen printing, flexography, gravure, offset lithography, and inkjet. By electronic industry standards, these are low cost processes. Electrically functional electronic or optical inks are deposited on the substrate, creating active or passive devices, such as thin film transistors; capacitors; coils; resistors. Printed electronics is expected to facilitate widespread, very low-cost, low-performance electronics for applications such as flexible displays, smart labels, decorative and animated posters, and active clothing that do not require high performance.[1]
The term printed electronics is often related to organic electronics or plastic electronics, in which one or more inks are composed of carbon-based compounds. These other terms refer to the ink material, which can be deposited by solution-based, vacuum-based or other processes. Printed electronics, in contrast, specifies the process, and, subject to the specific requirements of the printing process selected, can utilize any solution-based material. This includes organic semiconductors, inorganic semiconductors, metallic conductors, nanoparticles, nanotubes, etc.
For the preparation of printed electronics nearly all industrial printing methods are employed. Similar to conventional printing, printed electronics applies ink layers one atop another.[2] So the coherent development of printing methods and ink materials are the field's essential tasks.
The most important benefit of printing is low-cost volume fabrication. The lower cost enables use in more applications.[3] An example is RFID-systems, which enable contactless identification in trade and transport. In some domains, such as light-emitting diodes printing does not impact performance.[2] Printing on flexible substrates allows electronics to be placed on curved surfaces, for example, putting solar cells on vehicle roofs. More typically, conventional semiconductors justify their much higher costs by providing much higher performance.
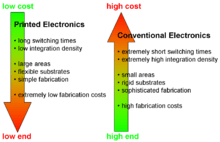
Resolution, registration, thickness, holes, materials
The maximum required resolution of structures in conventional printing is determined by the human eye. Feature sizes smaller than approximately 20 µm cannot be distinguished by the human eye and consequently exceed the capabilities of conventional printing processes.[4] In contrast, higher resolution and smaller structures are necessary in much electronics printing, because they directly affect circuit density and functionality (especially transistors). A similar requirement holds for the precision with which layers are printed on top of each other (layer to layer registration).
Control of thickness, holes, and material compatibility (wetting, adhesion, solvation) are essential, but matter in conventional printing only if the eye can detect them. Conversely, the visual impression is irrelevant for printed electronics.[5]
Printing technologies
The attraction of printing technology for the fabrication of electronics mainly results from the possibility of preparing stacks of micro-structured layers (and thereby thin-film devices) in a much simpler and cost-effective way compared to conventional electronics.[6] Also, the ability to implement new or improved functionalities (e.g. mechanical flexibility) plays a role. The selection of the printing method used is determined by requirements concerning printed layers, by the properties of printed materials as well as economic and technical considerations of the final printed products.
Printing technologies divide between sheet-based and roll-to-roll-based approaches. Sheet-based inkjet and screen printing are best for low-volume, high-precision work. Gravure, offset and flexographic printing are more common for high-volume production, such as solar cells, reaching 10.000 square meters per hour (m²/h).[4][6] While offset and flexographic printing are mainly used for inorganic[7][8] and organic[9][10] conductors (the latter also for dielectrics),[11] gravure printing is especially suitable for quality-sensitive layers like organic semiconductors and semiconductor/dielectric-interfaces in transistors, due to high layer quality.[11] If high resolution is needed, gravure is also suitable for inorganic[12] and organic[13] conductors. Organic field-effect transistors and integrated circuits can be prepared completely by means of mass-printing methods.[11]
Inkjets are flexible and versatile, and can be set up with relatively low effort.[14] However, inkjets offer lower throughput of around 100 m2/h and lower resolution (ca. 50 µm).[4] It is well suited for low-viscosity, soluble materials like organic semiconductors. With high-viscosity materials, like organic dielectrics, and dispersed particles, like inorganic metal inks, difficulties due to nozzle clogging occur. Because ink is deposited via droplets, thickness and dispersion homogeneity is reduced. Using many nozzles simultaneously and pre-structuring the substrate allows improvements in productivity and resolution, respectively. However, in the latter case non-printing methods must be employed for the actual patterning step.[15] Inkjet printing is preferable for organic semiconductors in organic field-effect transistors (OFETs) and organic light-emitting diodes (OLEDs), but also OFETs completely prepared by this method have been demonstrated.[16] Frontplanes[17] and backplanes[18] of OLED-displays, integrated circuits,[19] organic photovoltaic cells (OPVCs)[20] and other devices can be prepared with inkjets.
Screen printing is appropriate for fabricating electrics and electronics due to its ability to produce patterned, thick layers from paste-like materials. This method can produce conducting lines from inorganic materials (e.g. for circuit boards and antennas), but also insulating and passivating layers, whereby layer thickness is more important than high resolution. Its 50 m²/h throughput and 100 µm resolution are similar to inkjets.[4] This versatile and comparatively simple method is used mainly for conductive and dielectric layers,[21][22] but also organic semiconductors, e.g. for OPVCs,[23] and even complete OFETs[17] can be printed.
Aerosol Jet Printing (also known as Maskless Mesoscale Materials Deposition or M3D)[24] is another material deposition technology for printed electronics. The Aerosol Jet process begins with atomization of an ink, which can be heated up to 80 °C, producing droplets on the order of one to two micrometres in diameter. The atomized droplets are entrained in a gas stream and delivered to the print head. Here, an annular flow of clean gas is introduced around the aerosol stream to focus the droplets into a tightly collimated beam of material. The combined gas streams exit the print head through a converging nozzle that compresses the aerosol stream to a diameter as small as 10 µm. The jet of droplets exits the print head at high velocity (~50 meters/second) and impinges upon the substrate. Electrical interconnects, passive and active components[25] are formed by moving the print head, equipped with a mechanical stop/start shutter, relative to the substrate. The resulting patterns can have features ranging from 10 µm wide, with layer thicknesses from tens of nanometers to >10 µm.[26] A wide nozzle print head enables efficient patterning of millimeter size electronic features and surface coating applications. All printing occurs without the use of vacuum or pressure chambers and at room temperature. The high exit velocity of the jet enables a relatively large separation between the print head and the substrate, typically 2–5 mm. The droplets remain tightly focused over this distance, resulting in the ability to print conformal patterns over three dimensional substrates. Despite the high velocity, the printing process is gentle; substrate damage does not occur and there is generally no splatter or overspray from the droplets.[27] Once patterning is complete, the printed ink typically requires post treatment to attain final electrical and mechanical properties. Post-treatment is driven more by the specific ink and substrate combination than by the printing process. A wide range of materials has been successfully deposited with the Aerosol Jet process, including diluted thick film pastes, thermosetting polymers such as UV-curable epoxies, and solvent-based polymers like polyurethane and polyimide, and biologic materials.[28]
Evaporation printing uses a combination of high precision screen printing with material vaporization to print features to 5 µm. This method uses techniques such as thermal, e-beam, sputter and other traditional production technologies to deposit materials through a high precision shadow mask (or stencil) that is registered to the substrate to better than 1 micrometer. By layering different mask designs and/or adjusting materials, reliable, cost-effective circuits can be built additively, without the use of photolithography.
Other methods with similarities to printing, among them microcontact printing and nano-imprint lithography are of interest.[29] Here, µm- and nm-sized layers, respectively, are prepared by methods similar to stamping with soft and hard forms, respectively. Often the actual structures are prepared subtractively, e.g. by deposition of etch masks or by lift-off processes. For example electrodes for OFETs can be prepared.[30][31] Sporadically pad printing is used in a similar manner.[32] Occasionally so-called transfer methods, where solid layers are transferred from a carrier to the substrate, are considered printed electronics.[33] Electrophotography is currently not used in printed electronics.
Materials
Both organic and inorganic materials are used for printed electronics. Ink materials must be available in liquid form, for solution, dispersion or suspension.[34] They must function as conductors, semiconductors, dielectrics, or insulators. Material costs must be fit for the application.
Electronic functionality and printability can interfere with each other, mandating careful optimization.[5] For example, a higher molecular weight in polymers enhances conductivity, but diminishes solubility. For printing, viscosity, surface tension and solid content must be tightly controlled. Cross-layer interactions such as wetting, adhesion, and solubility as well as post-deposition drying procedures affect the outcome. Additives often used in conventional printing inks are unavailable, because they often defeat electronic functionality.
Material properties largely determine the differences between printed and conventional electronics. Printable materials provide decisive advantages beside printability, such as mechanical flexibility and functional adjustment by chemical modification (e.g. light color in OLEDs).[35]
Printed conductors offer lower conductivity and charge carrier mobility.[36]
With a few exceptions, inorganic ink materials are dispersions of metallic or semiconducting micro- and nano-particles. Semiconducting nanoparticles used include silicon[37] and oxide semiconductors.[38] Silicon is also printed as an organic precursor[39] which is then converted by pyrolisis and annealing into crystalline silicon.
PMOS but not CMOS is possible in printed electronics.[40]
Organic materials
Organic printed electronics integrates knowledge and developments from printing, electronics, chemistry, and materials science, especially from organic and polymer chemistry. Organic materials in part differ from conventional electronics in terms of structure, operation and functionality,[41] which influences device and circuit design and optimization as well as fabrication method.[42]
The discovery of conjugated polymers[36] and their development into soluble materials provided the first organic ink materials. Materials from this class of polymers variously possess conducting, semiconducting, electroluminescent, photovoltaic and other properties. Other polymers are used mostly as insulators and dielectrics.
In most organic materials, hole transport is favored over electron transport.[43] Recent studies indicate that this is a specific feature of organic semiconductor/dielectric-interfaces, which play a major role in OFETs.[44] Therefore p-type devices should dominate over n-type devices. Durability (resistance to dispersion) and lifetime is less than conventional materials.[40]
Organic semiconductors include the conductive polymers poly(3,4-ethylene dioxitiophene), doped with poly(styrene sulfonate), (PEDOT:PSS) and poly(aniline) (PANI). Both polymers are commercially available in different formulations and have been printed using inkjet,[45] screen[21] and offset printing[9] or screen,[21] flexo[10] and gravure[13] printing, respectively.
Polymer semiconductors are processed using inkjet printing, such as poly(thiopene)s like poly(3-hexylthiophene) (P3HT)[46] and poly(9,9-dioctylfluorene co-bithiophen) (F8T2).[47] The latter material has also been gravure printed.[11] Different electroluminescent polymers are used with inkjet printing,[15] as well as active materials for photovoltaics (e.g. blends of P3HT with fullerene derivatives),[48] which in part also can be deposited using screen printing (e.g. blends of poly(phenylene vinylene) with fullerene derivatives).[23]
Printable organic and inorganic insulators and dielectrics exist, which can be processed with different printing methods.[49]
Inorganic materials
Inorganic electronics provides highly ordered layers and interfaces that organic and polymer materials cannot provide.
Silver nanoparticles are used with flexo,[8] offset[50] and inkjet.[51] Gold particles are used with inkjet.[52]
A.C. electroluminescent (EL) multi-color displays can cover many tens of square meters, or be incorporated in watch faces and instrument displays. They involve six to eight printed inorganic layers, including a copper doped phosphor, on a plastic film substrate.[53]
CIGS cells can be printed directly onto molybdenum coated glass sheets.
A printed gallium arsenide germanium solar cell demonstrated 40.7% conversion efficiency, eight times that of the best organic cells, approaching the best performance of crystalline silicon.[53]
Substrates
Printed electronics allows the use of flexible substrates, which lowers production costs and allows fabrication of mechanically flexible circuits. While inkjet and screen printing typically imprint rigid substrates like glass and silicon, mass-printing methods nearly exclusively use flexible foil and paper. Poly(ethylene terephthalate)-foil (PET) is a common choice, due to its low cost and moderately high temperature stability. Poly(ethylene naphthalate)- (PEN) and poly(imide)-foil (PI) are higher performance, higher cost alternatives. Paper's low costs and manifold applications make it an attractive substrate, however, its high roughness and large absorbency make it problematic for electronics.[50]
Other important substrate criteria are low roughness and suitable wettability, which can be tuned pre-treatment by use of coating or Corona discharge. In contrast to conventional printing, high absorbency is usually disadvantageous.
Applications
Printed electronics are in use or under consideration for:
- Radio-frequency identification (RFID) tags
- Monitoring
- Data storage
- Display and visual effects
- Toys
Norwegian company ThinFilm successfully demonstrated roll-to-roll printed organic memory in 2009.[54][55][56][57]
Standards development and activities
Technical standards and roadmapping initiatives are intended to facilitate value chain development (for sharing of product specifications, characterization standards, etc.) This strategy of standards development mirrors the approach used by silicon-based electronics over the past 50 years. Initiatives include:
- The IEEE Standards Association has published IEEE 1620-2004[58] and IEEE 1620.1-2006.[59]
- Similar to the well-established International Technology Roadmap for Semiconductors (ITRS), the International Electronics Manufacturing Initiative (iNEMI)[60] has published a roadmap for printed and other organic electronics.
IPC—Association Connecting Electronics Industries has published three standards for printed electronics. All three have been published in cooperation with the Japan Electronic Packaging and Circuits Association (JPCA):
- IPC/JPCA-4921, Requirements for Printed Electronics Base Materials
- IPC/JPCA-4591, Requirements for Printed Electronics Functional Conductive Materials
- IPC/JPCA-2291, Design Guideline for Printed Electronics
These standards, and others in development, are part of IPC’s Printed Electronics Initiative.
See also
References
- ↑ Coatanéa, E., Kantola, V., Kulovesi, J., Lahti, L., Lin, R., & Zavodchikova, M. (2009). Printed Electronics, Now and Future. In Neuvo, Y., & Ylönen, S. (eds.), Bit Bang – Rays to the Future. Helsinki University of Technology (TKK), MIDE, Helsinki University Print, Helsinki, Finland, 63-102. ISBN 978-952-248-078-1. http://lib.tkk.fi/Reports/2009/isbn9789522480781.pdf
- 1 2 H.-K. Roth et al., Materialwissenschaft und Werkstofftechnik 32 (2001) 789.
- ↑ J.M. Xu, Synthetic Metals 115 (2000) 1.
- 1 2 3 4 A. Blayo and B. Pineaux, Joint sOC-EUSAI Conference, Grenoble, 2005.
- 1 2 U. Fügmann et al., mstNews 2 (2006) 13.
- 1 2 J.R. Sheats, Journal of Materials Research 19 (2004) 1974.
- ↑ P.M. Harrey et al., Sensors and Actuators B 87 (2002) 226.
- 1 2 J. Siden et al., Polytronic Conference, Wroclaw, 2005.
- 1 2 D. Zielke et al., Applied Physics Letters 87 (2005) 123580.
- 1 2 T. Mäkelä et al., Synthetic Metals 153 (2005) 285.
- 1 2 3 4 A. Hübler et al., Organic Electronics 8 (2007) 480.
- ↑ S. Leppavuori et al., Sensors and Actuators 41-42 (1994) 593.
- 1 2 T. Mäkelä et al., Synthetic Metals 135 (2003) 41.
- ↑ R. Parashkov et al., Proceedings IEEE 93 (2005) 1321.
- 1 2 B.-J. de Gans et al., Advanced Materials 16 (2004) 203.
- ↑ V. Subramanian et al., Proceedings IEEE 93 (2005) 1330.
- 1 2 S. Holdcroft, Advanced Materials 13 (2001) 1753.
- ↑ A.C. Arias et al., Applied Physics Letters 85 (2004) 3304.
- ↑ H. Sirringhaus et al., Science 290 (2000) 2123.
- ↑ V.G. Shah and D.B. Wallace, IMAPS Conference, Long Beach, 2004.
- 1 2 3 K. Bock et al., Proceedings IEEE 93 (2005) 1400.
- ↑ Z. Bao et al., Chemistry of Materials 9 (1997) 1299.
- 1 2 S.E. Shaheen et al., Applied Physics Letters 79 (2001) 2996.
- ↑ M. Renn, US Patent number 7,485,345 B2. Page 3.
- ↑ J.H. Cho et al, Nature Materials, 19 October 2008.
- ↑ B. Kahn, Organic and Printed Electronics, Volume 1, Issue 2 (2007).
- ↑ B. H. King et al, Photovoltaic Specialists Conference (PVSC), 2009 34th IEEE.
- ↑ Ingo Grunwald et al 2010 Biofabrication 2 014106).
- ↑ B.D. Gate et al., Chemical Reviews 105 (2005) 1171.
- ↑ D. Li and L.J. Guo, Applied Physics Letters 88 (2006) 063513.
- ↑ G. Leising et al., Microelectronics Engineering 83 (2006) 831.
- ↑ A. Knobloch et al., Journal of Applied Physics 96 (2004) 2286.
- ↑ D.R. Hines et al., Journal of Applied Physics 101 (2007) 024503.
- ↑ Z. Bao, Advanced Materials 12 (2000) 227.
- ↑ Moliton and R.C. Hiorns, Polymer International 53 (2004) 1397.
- 1 2 http://nobelprize.org/nobel_prizes/chemistry/laureates/2000/chemadv.pdf Nobel prize in chemistry, 2000
- ↑ U. Maennl et al, Japanese Journal of Applied Physics 2013, http://dx.doi.org/10.7567/JJAP.52.05DA11
- ↑ H. Faber et al, Advanced Materials 29(2009)3099.
- ↑ T. Shimoda et al, Nature 440 (2006) 783 - 786.
- 1 2 D.M. de Leeuw et al., Synthetic Metals 87 (1997) 53.
- ↑ Z.V. Vardeny et al., Synthetic Metals 148 (2005) 1.
- ↑ H. Kempa et al., it 3 (2008) 167.
- ↑ Fachetti, Materials Today 10 (2007) 38.
- ↑ J. Zaumseil and H. Sirringhaus, Chemical Reviews 107 (2007) 1296.
- ↑ J. Bharathan and Y. Yang, Applied Physics Letters 72 (2006) 2660.
- ↑ S.P. Speakman et al., Organic Electronics 2 (2001) 65.
- ↑ K.E. Paul et al., Applied Physics Letters 83 (2003) 2070.
- ↑ T. Aernouts et al., Applied Physics Letters 92 (2008) 033306.
- ↑ "Ion Gel Insulator". Archived from the original on November 14, 2011.
- 1 2 P.M. Harrey et al., Journal of Electronics Manufacturing 10 (2000) 69.
- ↑ J. Perelaer et al., Advanced Materials 18 (2006) 2101.
- ↑ Y.-Y. Noh et al., Nature Nanotechnology 2 (2007) 784.
- 1 2 Mflex UK (formerly Pelikon) and elumin8, both in the UK, Emirates Technical Innovation Centre in Dubai, Schreiner in Germany and others are involved in EL displays. Spectrolab already offers commercially flexible solar cells based on various inorganic compounds.
- ↑ Thinfilm and InkTec awarded IDTechEx' Technical Development Manufacturing Award IDTechEx, April 15th 2009
- ↑ PolyIC, ThinFilm announce pilot of volume printed plastic memories EETimes, September 22nd 2009
- ↑ All set for high-volume production of printed memories Printed Electronics World, April 12th 2010
- ↑ Thin Film Electronics Plans to Provide ‘Memory Everywhere’ Printed Electronics Now, May 2010
- ↑ http://grouper.ieee.org/groups/1620/
- ↑ http://grouper.ieee.org/groups/1620/1/
- ↑ http://www.inemi.org/
External links
- For a history of the field, see Printed Organic and Molecular Electronics, edited by D. Gamota, P. Brazis, K. Kalyanasundaram, and J. Zhang (Kluwer Academic Publishers: New York, 2004). ISBN 1-4020-7707-6
- Cleaner Electronics Research Group - Brunel University
- Encyclopedia of Printed Electronics (IDTechEx)
- Printed Electronics conference/exhibition Asia USA
- Come Back Inorganic Electronics - All Is Forgiven
- New Nano Silver Powder Enables Flexible Printed Circuits (Ferro Corporation)
- Western Michigan University's Center for Advancement of Printed Electronics (CAPE) includes AccuPress gravure printer
- Major Trends in Gravure Printed Electronics June 2010
- Printed Electronics – avistando el futuro. Printed Electronics en Español
- Organic Solar Cells - Theory and Practice (Coursera)