Polyhydroxyalkanoates
-3-hydroxybutyrat.svg.png)

Polyhydroxyalkanoates or PHAs are linear polyesters produced in nature by bacterial fermentation of sugar or lipids. They are produced by the bacteria to store carbon and energy. More than 150 different monomers can be combined within this family to give materials with extremely different properties.[1] These plastics are biodegradeable and are used in the production of bioplastics.
They can be either thermoplastic or elastomeric materials, with melting points ranging from 40 to 180 °C.
The mechanical and biocompatibility of PHA can also be changed by blending, modifying the surface or combining PHA with other polymers, enzymes and inorganic materials, making it possible for a wider range of applications.[2]
Biosynthesis
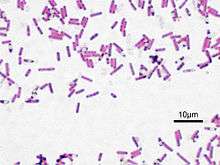
To produce PHA, a culture of a micro-organism such as Alcaligenes eutrophus is placed in a suitable medium and fed appropriate nutrients so that it multiplies rapidly. Once the population has reached a substantial level, the nutrient composition is changed to force the micro-organism to synthesize PHA. The yield of PHA obtained from the intracellular inclusions can be as high as 80% of the organism's dry weight.
The biosynthesis of PHA is usually caused by certain deficiency conditions (e.g. lack of macro elements such as phosphorus, nitrogen, trace elements, or lack of oxygen) and the excess supply of carbon sources.
Polyesters are deposited in the form of highly refractive granules in the cells. Depending upon the microorganism and the cultivation conditions, homo- or copolyesters with different hydroxyalkanic acids are generated. PHA granules are then recovered by disrupting the cells.[3] Recombinants Bacillus subtilis str. pBE2C1 and Bacillus subtilis str. pBE2C1AB were used in production of polyhydroxyalkanoates (PHA) and it was shown that they could use malt waste as carbon source for lower cost of PHA production.
PHA synthases are the key enzymes of PHA biosynthesis. They use the coenzyme A - thioester of (r)-hydroxy fatty acids as substrates. The two classes of PHA synthases differ in the specific use of hydroxy fatty acids of short or medium chain length.
The resulting PHA is of the two types:
- Poly (HA SCL) from hydroxy fatty acids with short chain lengths including three to five carbon atoms are synthesized by numerous bacteria, including Ralstonia eutropha and Alcaligenes latus (PHB).
- Poly (HA MCL) from hydroxy fatty acids with middle chain lengths including six to 14 carbon atoms, can be made for example, by Pseudomonas putida.
A few bacteria, including Aeromonas hydrophila and Thiococcus pfennigii, synthesize copolyester from the above two types of hydroxy fatty acids, or at least possess enzymes that are capable of part of this synthesis.
Another even larger scale synthesis can be done with the help of soil organisms. For lack of nitrogen and phosphorus they produce a kilogram of PHA per three kilograms of sugar.
The simplest and most commonly occurring form of PHA is the fermentative production of poly-beta-hydroxybutyrate (poly-3-hydroxybutyrate, P3HB), which consists of 1000 to 30000 hydroxy fatty acid monomers.
Industrial production
In the industrial production of PHA, the polyester is extracted and purified from the bacteria by optimizing the conditions of microbial fermentation of sugar or glucose.
In the 1980s, Imperial Chemical Industries developed poly(3-hydroxybutyrate-co-3-hydroxyvalerate) obtained via fermentation that was named "Biopol". It was sold under the name "Biopol" and distributed in the U.S. by Monsanto and later Metabolix.[4]
As raw material for the fermentation, carbohydrates such as glucose and sucrose can be used, but also vegetable oil or glycerine from biodiesel production. Researchers in industry are working on methods with which transgenic crops will be developed that express PHA synthesis routes from bacteria and so produce PHA as energy storage in their tissues. Several companies are working to develop methods of producing PHA from waste water, including start-up Micromidas[5] and Veolia subsidiary Anoxkaldnes.[6]
PHAs are processed mainly via injection molding, extrusion and extrusion bubbles into films and hollow bodies.
Material properties
PHA polymers are thermoplastic, can be processed on conventional processing equipment, and are, depending on their composition, ductile and more or less elastic. They differ in their properties according to their chemical composition (homo-or copolyester, contained hydroxy fatty acids).
They are UV stable, in contrast to other bioplastics from polymers such as polylactic acid, partial ca. temperatures up to 180 °C, and show a low permeation of water. The crystallinity can lie in the range of a few to 70%. Processability, impact strength and flexibility improves with a higher percentage of valerate in the material. PHAs are soluble in halogenated solvents such chloroform, dichloromethane or dichloroethane.[7]
PHB is similar in its material properties to polypropylene (PP), has a good resistance to moisture and aroma barrier properties. Polyhydroxybutyric acid synthesized from pure PHB is relatively brittle and stiff. PHB copolymers, which may include other fatty acids such as beta-hydroxyvaleriate acid, may be elastic.
Applications
-
Structure of poly-3-hydroxyvalerate (PHV)
-
Structure of poly-4-hydroxybutyrate (P4HB)
A PHA copolymer called PHBV (poly(3-hydroxybutyrate-co-3-hydroxyvalerate)) is less stiff and tougher, and it may be used as packaging material.
In June 2005, a US company (Metabolix, Inc.) received the US Presidential Green Chemistry Challenge Award (small business category) for their development and commercialisation of a cost-effective method for manufacturing PHAs.
There are potential applications for PHA produced by micro-organisms[1] within the medical and pharmaceutical industries, primarily due to their biodegradability.
Fixation and orthopaedic applications have included sutures, suture fasteners, meniscus repair devices, rivets, tacks, staples, screws (including interference screws), bone plates and bone plating systems, surgical mesh, repair patches, slings, cardiovascular patches, orthopedic pins (including bone.lling augmentation material), adhesion barriers, stents, guided tissue repair/regeneration devices, articular cartilage repair devices, nerve guides, tendon repair devices, atrial septal defect repair devices, pericardial patches, bulking and filling agents, vein valves, bone marrow scaffolds, meniscus regeneration devices, ligament and tendon grafts, ocular cell implants, spinal fusion cages, skin substitutes, dural substitutes, bone graft substitutes, bone dowels, wound dressings, and hemostats.[8]
References
- 1 2 Doi, Y; Steinbuchel, A. (2002). Biopolymers. Weinheim, Germany: Wiley-VCH.
- ↑ Polyhydroxyalkanoates for tissue engineering
- ↑ Jacquel, N.; et al. (2008). "Isolation and purification of bacterial poly(3-hydroxyalkanoates)". Biochem. Eng. J. 39 (1): 15–27. doi:10.1016/j.bej.2007.11.029.
- ↑ Ewa Rudnik (3 January 2008). Compostable Polymer Materials. Elsevier. p. 21. ISBN 978-0-08-045371-2. Retrieved 10 July 2012.
- ↑ Martin Lamonica (May 27, 2010). "Micromidas to test sludge-to-plastic tech". CNET. Retrieved October 23, 2015.
- ↑ Seb Egerton-Read (September 9, 2015). "A New Way to Make Plastic". Circulate. Retrieved October 23, 2015.
- ↑ Jacquel, N.; et al. (2007). "Solubility of polyhydroxyalkanoates by experiment and thermodynamic correlations". AlChE J. 53 (10): 2704–2714. doi:10.1002/aic.11274.
- ↑ Chen, GQ; Wu, Q (2005). "The application of polyhydroxyalkanoates as tissue engineering materials". Biomaterials 26 (33): 6565–78. doi:10.1016/j.biomaterials.2005.04.036. PMID 15946738.