Parasite increased trophic transmission
Parasite increased trophic transmission (PITT) is a mode of parasite transmission in which the parasite manipulates the behavior of its host to increase transmission success to higher trophic levels.[1] Parasites that use PITT have complex life cycles,[2] requiring more than one host species to procreate.[1] Sexual reproduction is typically limited to a definitive host, high in the trophic levels.[3] Parasite progeny sequentially infect one or more intermediate hosts, inside which they may undergo asexual reproduction and physiological changes. Trophic transmission often relies on predator-prey relationships,[4] where the parasite can only be transmitted if the host is eaten by a later stage host. Parasitic manipulation of the intermediate host can involve adaptations that make it an easier prey for predators, thereby increasing the transmission rate of the parasite.[5][6]
Evolutionary aspects
For complex life cycles to emerge in parasites, the addition of intermediate host species must be beneficial, e.g., result in a higher fitness.[7][8] It is probable that most parasites with complex life cycles evolved from simple life cycles.[9] The transfer from simple to complex life cycles has been analyzed theoretically, and it has been shown that trophically transmitted parasites can be favored by the addition of an intermediate prey host if the population density of the intermediate host is higher than that of the definitive host.[9] Additional factors that catalyze this transfer are high predation rates, and a low natural mortality rate of the intermediate host.[9]
Parasites with a single host species are faced with the problem of not being able to survive in higher trophic levels and therefore dying with its prey host. The development of complex life cycles is most likely an adaptation of the parasite to survive in the predator.[6] The development of parasite increased trophic transmission is a further adaptation in relation to a complex life cycle, where the parasite increases its transmission to a definitive host by manipulating its intermediate host.[7] The parasitic manipulation of host behavior is not necessarily an effect of PITT; it could also be a behavioral change induced as a side effect of the infection itself.[10]
Host and parasite
The coexistence of host and parasite can initiate an evolutionary arms race, resulting in the development of new parasitic strategies and new host defenses. The selective force in susceptible hosts to fight off parasitic infections is often very strong, especially when the parasite kills the host. The parasite is also often strongly affected by selective forces, especially to ensure successful transmission to new hosts and overcome the host’s defenses to survive and reproduce.
Parasitic host manipulation
The development of complex life cycles can increase parasite fitness. Some parasites have, by host manipulation, further increased their reproductive success. Host manipulation is a trait shown in some parasites, where they effectively can change the behavior or morphology of their intermediate host in a way that increases the parasite's trophic transmission.[5][6]
Direct and indirect manipulation
Parasite manipulations could be either direct or indirect. Indirect manipulation is the most frequent method used by behavioral altering parasites,[11] while the direct approach is far less common. Direct manipulation is when the parasite itself affects the host and induces a behavioral response, for example by creating neuroactive compounds that stimulate a response in the host’s central nervous system (CNS), a method mostly practiced by parasites that reside within the CNS.[12] Affecting the host's neural system is complicated and manipulation includes initiating immune cascades in the host.[13] However, determination of the causative factor is difficult, especially whether the behavioral change is the result of direct manipulation from the parasite, or an indirect response of the host’s immune system.[12] A direct approach to behavioral manipulation is often very costly for the parasite,[12] which results in a trade-off between the benefits of the manipulation (e.g., fitness increase) and the energy it costs. The more common approach for parasites is to indirectly induce behavioral responses by interacting with the host’s immune system.[11] It can manipulate the host’s immune system to create the necessary neuroactive compounds to induce a desired behavioral response.[12] Parasites can also indirectly affect the behavior of its host by disturbing its metabolism, development or immunity.[12]
Parasites in intermediate and definitive hosts
Parasites with complex life cycles often behave differently in their intermediate and definitive hosts.[10][14] High virulence that results in the death of an intermediate host before it can be preyed upon by a definitive host is maladaptive. The parasite therefore needs to evolve different levels of virulence at different life stages in different hosts. Parasite increased trophic transmission often follows this trend, because the altering of host behavior is strongly affected by the requirements of the parasite. One example has been observed in the interactions of the parasitic tapeworm Echinococcus granulosus and its intermediate host (moose) and its definitive host (wolf).[10] The tapeworm virulence is high in the moose but low in the wolf, because a strong effect that inhibits normal functions in the moose makes it an easier target for the wolf. A high virulence in the wolf is unfavorable if it decreases predator abilities. Thus, the tapeworm’s fitness is increased by a high virulence in the moose and a benign relationship with the wolf.[10]
Host defense
Parasite infections are always to some extent harmful to the host, and sometimes lead to death. Infection by parasites that use PITT is often a death sentence for the intermediate hosts. Hosts possess physiological and immunological defenses,[7] such as physical barriers and innate and adaptive immune systems, which defend against parasites and other pathogens. Some host species have also evolved behavioral defense mechanisms.[7]
One of the most conspicuous examples of behavioral adaptation against parasitic infection is self-induced death. The host may commit suicide or behave abnormally to increase the chance of it being preyed upon. Thus, the host sacrifices itself to prevent the parasite from maturing and therefore reduce its transmission. This subsequently reduces the risk infection to the host’s kin, and therefore increases the host’s inclusive fitness.[7] Another example of a behavioral adaptation is the increase in reproductive output as a response of a parasitic infection,[15] which is consistent with the hypothesis of fecundity compensation.[16] Thus, the host may increase reproductive effort to compensate for the negative effect the parasite may have on its reproductive abilities.
Ecological aspects
To understand the phenomenon of parasite increased trophic transmission, it is important to place it in an ecological context.[12] One factor that strongly affects this kind of parasitic transmission is the fact that manipulated intermediate hosts become viable prey for all predators, not just for those the parasite intends.[2] This raises a problem for parasites that use PITT, because if they end up in a non-host predator, they either die or can't reproduce.
Studies show that some parasites manipulate their intermediate host to reduce the risk of it being eaten by a non-host predator. One example of this is the parasitic trematode Microphallus sp., which uses the snail Potamopyrgus antipodarum as an intermediate host. The parasite manipulates the snail’s foraging behavior to increase the chance of it being preyed upon by the parasite’s definitive hosts (waterfowl). The infected snail forages on the upper side of rocks during the period of the day when waterfowl feed most intensely. During the rest of the day, the snail forages at the bottom of rocks to reduce the risk of being eaten by fish (non-hosts for the parasitic trematode).[17]
Examples of parasite increased trophic transmission
Parasite increased trophic transmission is a way for parasitic organisms that rely on more than one host to increase their transmission between trophic levels. This mode of transmission has been shown to be practiced by most forms of microscopic organisms. Examples can be observed in bacteria, protozoa, viruses, and animals.

Toxoplasma gondii
Toxoplasma gondii is a parasitic protozoan, which has been shown to alter the behavior of rats, its intermediate host. T. gondii is potentially fatal to the rat, not in the sense that it reduces the general health of its host, but rather in that it alters its perception towards an impending predatorial threat, in this case from cats (definitive host of T. gondii). T. gondii manipulates the rat's perception of the odor originating from cats,[18] making the rat less afraid of their predator, thus increasing the chance of the rat being eaten and the protozoan reaching its definitive host.
Dicrocoelium dendriticum
The lancet liver fluke (Dicrocoelium dendriticum) is a parasitic trematode with a complex life cycle. In its adult state it occurs in the liver of its definitive host (ruminants), where it reproduces. The parasite eggs are passed with the feces of the host, which then are eaten by a terrestrial snail (first intermediate host). The fluke matures into a juvenile stage in the snail, which in an attempt to protect itself excretes the parasites in “slime-balls”. The “slime-balls” are then consumed by ants (second intermediate hosts). The fluke manipulates the ant to move up to the top of grass, where they have a higher chance of being eaten by grazing ruminants.[19]
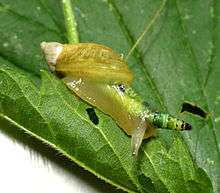
Myrmeconema neotropicum
The parasitic nematode Myrmeconema neotropicum infects the intermediate ant host Cephalotes atratus. The nematode then induces a morphological change in the ant, which turns the gaster color from black to red, making it resemble fruit. This color transition makes the ant susceptible to predation by frugivorous birds, which act as the parasite’s definitive hosts. The parasitic eggs are deposited in the bird’s feces and are eaten by ants, which complete the cycle.[20]
Schistocephalus solidus
Schistocephalus solidus is a parasitic tapeworm with three different hosts, two intermediate and one definitive. In its adult stage the tapeworm resides in the intestine of piscivorous birds, where they reproduce and release eggs through the bird’s feces. Free-swimming larvae hatch from the eggs, which are in turn ingested by copepods (the first intermediate host). The parasite grows and develops in the crustacean into a stage that can infect the second intermediate host, the three-spined stickleback (Gasterosteus aculeatus).[21] The parasite’s definitive host, a bird, then consumes the infected three-spined stickleback and the cycle is complete. It has been observed that S. solidus alters the behavior of the fish in a manner that impedes its escape response when faced with a predatorial bird.[22] This parasite-induced behavioral manipulation effectively increases the chance of it being consumed by its definitive bird host. It has also been observed that the parasite does not induce this behavior until it has reached a developed stage that can survive in the host bird[22] and therefore effectively reduce its own mortality rate, due to premature transmission.
Leucochloridium paradoxum
The trematode Leucochloridium paradoxum reproduces in its adult stage in bird’s intestines. The eggs are released with the feces and are eaten by snails of the genus Succinea. The parasite matures inside the snail into a large, colorful and motile form, which bears a striking resembles to an insect larvae[23] (a common food for the parasite’s definitive host, bird). A bird then consumes the snail or just the parasite, which completes the parasite’s life cycle.
References
- 1 2 Seppala, O., Valtonen, E. and Benesh, D. (2008). Host manipulation by parasites in the world of dead-end predators: adaptation to enhance transmission?. Proceedings of the Royal Society B: Biological Sciences, 275(1643), 1611-1615.
- 1 2 Mouritsen, K. and Poulin, R. (2003). Parasite-induced trophic facilitation exploited by a non-host predator: a manipulator's nightmare. International Journal for Parasitology, 33(10), 1043-1050.
- ↑ Schmidt, G. and Roberts, L. (1985). Foundations of parasitology. St. Louis: Times Mirror/Mosby College Pub.
- ↑ Luong, L., Grear, D. and Hudson, P. (2014). Manipulation of host-resource dynamics impacts transmission of trophic parasites. International Journal for Parasitology, 44(10), 737-742.
- 1 2 Moore, J. (2002). Parasites and the behavior of animals. Oxford: Oxford University Press.
- 1 2 3 Lafferty, K. (1999). The Evolution of Trophic Transmission. Parasitology Today, 15(3), 111-115.
- 1 2 3 4 5 Trail, D. (1980). Behavioral Interactions between Parasites and Hosts: Host Suicide and the Evolution of Complex Life Cycles. The American Naturalist, 116(1), 77-91.
- ↑ Brown, S., Renaud, F., Guégan, J. and Thomas, F. (2001). Evolution of trophic transmission in parasites: the need to reach a mating place?. Journal of Evolutionary Biology, 14(5), 815-820.
- 1 2 3 Choisy, M., Brown, S., Lafferty, K. and Thomas, F. (2003). Evolution of Trophic Transmission in Parasites: Why Add Intermediate Hosts?. Am Nat, 162(2), 172-181.
- 1 2 3 4 Wobeser, G. (2006). Essentials of disease in wild animals. Ames, Iowa: Blackwell Pub.
- 1 2 Adamo, S. (2002). Modulating the Modulators: Parasites, Neuromodulators and Host Behavioral Change. Brain, Behavior and Evolution, 60(6), 370-377.
- 1 2 3 4 5 6 Thomas, F., Adamo, S. and Moore, J. (2005). Parasitic manipulation: where are we and where should we go?. Behavioural Processes, 68(3), 185-199.
- ↑ Tomonaga, K. (2004). Virus-induced neurobehavioral disorders: mechanisms and implications. Trends in Molecular Medicine, 10(2), 71-77.
- ↑ Combes, C. (1991). Ethological Aspects of Parasite Transmission. The American Naturalist, 138(4), 866-880.
- ↑ Schwanz, L. (2008). Chronic parasitic infection alters reproductive output in deer mice. Behav Ecol Sociobiol, 62(8), 1351-1358.
- ↑ Minchella, D. and Loverde, P. (1981). A Cost of Increased Early Reproductive Effort in the Snail Biomphalaria glabrata. The American Naturalist, 118(6), 876-881.
- ↑ Levri, E. (1998). The Influence of Non-Host Predators on Parasite-Induced Behavioral Changes in a Freshwater Snail. Oikos, 81(3), 531-537.
- ↑ Berdoy, M., Webster, J. and Macdonald, D. (2000). Fatal attraction in rats infected with Toxoplasma gondii. Proceedings of the Royal Society B: Biological Sciences, 267(1452), 1591-1594.
- ↑ Otranto, D. and Traversa, D. (2002). A review of dicrocoeliosis of ruminants including recent advances in the diagnosis and treatment. Veterinary Parasitology, 107(4), 317-335.
- ↑ Yanoviak, S., Kaspari, M., Dudley, R. and Poinar, G. (2008). Parasite-Induced Fruit Mimicry in a Tropical Canopy Ant. Am Nat, 171(4), 536-544.
- ↑ Benesh, D. and Hafer, N. (2012). Growth and ontogeny of the tapeworm Schistocephalus solidus in its copepod first host affects performance in its stickleback second intermediate host. Parasites & Vectors, 5(1), 90.
- 1 2 Barber, I., Walker, P. and Svensson, P. (2004). Behavioural Responses to Simulated Avian Predation in Female Three Spined Sticklebacks: The Effect of Experimental Schistocephalus Solidus Infections. Behaviour, 141(11), 1425-1440.
- ↑ Robinson, E. (1947). Notes on the Life History of Leucochloridium fuscostriatum n. sp. provis. (Trematoda: Brachylaemidae). The Journal of Parasitology, 33(6), 467-475.