Non-coding RNA
A non-coding RNA (ncRNA) is an RNA molecule that is not translated into a protein. Less-frequently used synonyms are non-protein-coding RNA (npcRNA), non-messenger RNA (nmRNA) and functional RNA (fRNA). The DNA sequence from which a functional non-coding RNA is transcribed is often called an RNA gene.
Non-coding RNA genes include highly abundant and functionally important RNAs such as transfer RNAs (tRNAs) and ribosomal RNAs (rRNAs), as well as RNAs such as snoRNAs, microRNAs, siRNAs, snRNAs, exRNAs, piRNAs and scaRNAs and the long ncRNAs that include examples such as Xist and HOTAIR (see here for a more complete list of ncRNAs). The number of ncRNAs encoded within the human genome is unknown; however, recent transcriptomic and bioinformatic studies suggest the existence of thousands of ncRNAs.[1][2][3][4], but see [5] Since many of the newly identified ncRNAs have not been validated for their function, it is possible that many are non-functional.[6] It is also likely that many ncRNAs are non functional (sometimes referred to as Junk RNA), and are the product of spurious transcription.[7]
History and discovery
Nucleic acids were first discovered in 1868 by Friedrich Miescher[8] and by 1939 RNA had been implicated in protein synthesis.[9] Two decades later, Francis Crick predicted a functional RNA component which mediated translation; he reasoned that RNA is better suited to base-pair with an mRNA transcript than a pure polypeptide.[10]

The first non-coding RNA to be characterised was an alanine tRNA found in baker's yeast, its structure was published in 1965.[11] To produce a purified alanine tRNA sample, Robert W. Holley et al. used 140kg of commercial baker's yeast to give just 1g of purified tRNAAla for analysis.[12] The 80 nucleotide tRNA was sequenced by first being digested with Pancreatic ribonuclease (producing fragments ending in Cytosine or Uridine) and then with takadiastase ribonuclease Tl (producing fragments which finished with Guanosine). Chromatography and identification of the 5' and 3' ends then helped arrange the fragments to establish the RNA sequence.[12] Of the three structures originally proposed for this tRNA,[11] the 'cloverleaf' structure was independently proposed in several following publications.[13][14][15][16] The cloverleaf secondary structure was finalised following X-ray crystallography analysis performed by two independent research groups in 1974.[17][18]
Ribosomal RNA was next to be discovered, followed by URNA in the early 1980s. Since then, the discovery of new non-coding RNAs has continued with snoRNAs, Xist, CRISPR and many more.[19] Recent notable additions include riboswitches and miRNA; the discovery of the RNAi mechanism associated with the latter earned Craig C. Mello and Andrew Fire the 2006 Nobel Prize in Physiology or Medicine.[20]
Biological roles of ncRNA
Noncoding RNAs belong to several groups and are involved in many cellular processes. These range from ncRNAs of central importance that are conserved across all or most cellular life through to more transient ncRNAs specific to one or a few closely related species. The more conserved ncRNAs are thought to be molecular fossils or relics from LUCA and the RNA world and their current roles remain mostly in regulation of information flow from DNA to protein.[21][22][23]
ncRNAs in translation

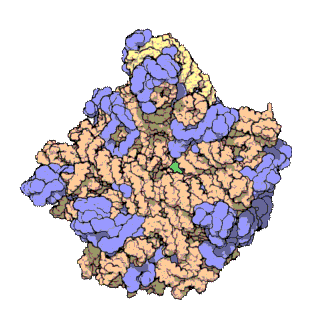
Many of the conserved, essential and abundant ncRNAs are involved in translation. Ribonucleoprotein (RNP) particles called ribosomes are the 'factories' where translation takes place in the cell. The ribosome consists of more than 60% ribosomal RNA; these are made up of 3 ncRNAs in prokaryotes and 4 ncRNAs in eukaryotes. Ribosomal RNAs catalyse the translation of nucleotide sequences to protein. Another set of ncRNAs, Transfer RNAs, form an 'adaptor molecule' between mRNA and protein. The H/ACA box and C/D box snoRNAs are ncRNAs found in archaea and eukaryotes. RNase MRP is restricted to eukaryotes. Both groups of ncRNA are involved in the maturation of rRNA. The snoRNAs guide covalent modifications of rRNA, tRNA and snRNAs; RNase MRP cleaves the internal transcribed spacer 1 between 18S and 5.8S rRNAs. The ubiquitous ncRNA, RNase P, is an evolutionary relative of RNase MRP.[25] RNase P matures tRNA sequences by generating mature 5'-ends of tRNAs through cleaving the 5'-leader elements of precursor-tRNAs. Another ubiquitous RNP called SRP recognizes and transports specific nascent proteins to the endoplasmic reticulum in eukaryotes and the plasma membrane in prokaryotes. In bacteria Transfer-messenger RNA (tmRNA) is an RNP involved in rescuing stalled ribosomes, tagging incomplete polypeptides and promoting the degradation of aberrant mRNA.
ncRNAs in RNA splicing

In eukaryotes the spliceosome performs the splicing reactions essential for removing intron sequences, this process is required for the formation of mature mRNA. The spliceosome is another RNP often also known as the snRNP or tri-snRNP. There are two different forms of the spliceosome, the major and minor forms. The ncRNA components of the major spliceosome are U1, U2, U4, U5, and U6. The ncRNA components of the minor spliceosome are U11, U12, U5, U4atac and U6atac.
Another group of introns can catalyse their own removal from host transcripts; these are called self-splicing RNAs. There are two main groups of self-splicing RNAs: group I catalytic intron and group II catalytic intron. These ncRNAs catalyze their own excision from mRNA, tRNA and rRNA precursors in a wide range of organisms.
In mammals it has been found that snoRNAs can also regulate the alternative splicing of mRNA, for example snoRNA HBII-52 regulates the splicing of serotonin receptor 2C.[26]
In nematodes, the SmY ncRNA appears to be involved in mRNA trans-splicing.
ncRNAs in DNA replication

Y RNAs are stem loops, necessary for DNA replication through interactions with chromatin and initiation proteins (including the origin recognition complex).[28][29] They are also components of the Ro60 ribonucleoprotein particle[30] which is a target of autoimmune antibodies in patients with systemic lupus erythematosus.[31]
ncRNAs in gene regulation
The expression of many thousands of genes are regulated by ncRNAs. This regulation can occur in trans or in cis.
Trans-acting ncRNAs
In higher eukaryotes microRNAs regulate gene expression. A single miRNA can reduce the expression levels of hundreds of genes. The mechanism by which mature miRNA molecules act is through partial complementary to one or more messenger RNA (mRNA) molecules, generally in 3' UTRs. The main function of miRNAs is to down-regulate gene expression.
The ncRNA RNase P has also been shown to influence gene expression. In the human nucleus RNase P is required for the normal and efficient transcription of various ncRNAs transcribed by RNA polymerase III. These include tRNA, 5S rRNA, SRP RNA, and U6 snRNA genes. RNase P exerts its role in transcription through association with Pol III and chromatin of active tRNA and 5S rRNA genes.[32]
It has been shown that 7SK RNA, a metazoan ncRNA, acts as a negative regulator of the RNA polymerase II elongation factor P-TEFb, and that this activity is influenced by stress response pathways.
The bacterial ncRNA, 6S RNA, specifically associates with RNA polymerase holoenzyme containing the sigma70 specificity factor. This interaction represses expression from a sigma70-dependent promoter during stationary phase.
Another bacterial ncRNA, OxyS RNA represses translation by binding to Shine-Dalgarno sequences thereby occluding ribosome binding. OxyS RNA is induced in response to oxidative stress in Escherichia coli.
The B2 RNA is a small noncoding RNA polymerase III transcript that represses mRNA transcription in response to heat shock in mouse cells. B2 RNA inhibits transcription by binding to core Pol II. Through this interaction, B2 RNA assembles into preinitiation complexes at the promoter and blocks RNA synthesis.[33]
A recent study has shown that just the act of transcription of ncRNA sequence can have an influence on gene expression. RNA polymerase II transcription of ncRNAs is required for chromatin remodelling in the Schizosaccharomyces pombe. Chromatin is progressively converted to an open configuration, as several species of ncRNAs are transcribed.[34]
Cis-acting ncRNAs
A number of ncRNAs are embedded in the 5' UTRs (Untranslated Regions) of protein coding genes and influence their expression in various ways. For example, a riboswitch can directly bind a small target molecule; the binding of the target affects the gene's activity.
RNA leader sequences are found upstream of the first gene of amino acid biosynthetic operons. These RNA elements form one of two possible structures in regions encoding very short peptide sequences that are rich in the end product amino acid of the operon. A terminator structure forms when there is an excess of the regulatory amino acid and ribosome movement over the leader transcript is not impeded. When there is a deficiency of the charged tRNA of the regulatory amino acid the ribosome translating the leader peptide stalls and the antiterminator structure forms. This allows RNA polymerase to transcribe the operon. Known RNA leaders are Histidine operon leader, Leucine operon leader, Threonine operon leader and the Tryptophan operon leader.
Iron response elements (IRE) are bound by iron response proteins (IRP). The IRE is found in UTRs of various mRNAs whose products are involved in iron metabolism. When iron concentration is low, IRPs bind the ferritin mRNA IRE leading to translation repression.
Internal ribosome entry sites (IRES) are RNA structures that allow for translation initiation in the middle of a mRNA sequence as part of the process of protein synthesis.
ncRNAs and genome defense
Piwi-interacting RNAs (piRNAs) expressed in mammalian testes and somatic cells form RNA-protein complexes with Piwi proteins. These piRNA complexes (piRCs) have been linked to transcriptional gene silencing of retrotransposons and other genetic elements in germ line cells, particularly those in spermatogenesis.
Clustered Regularly Interspaced Short Palindromic Repeats (CRISPR) are repeats found in the DNA of many bacteria and archaea. The repeats are separated by spacers of similar length. It has been demonstrated that these spacers can be derived from phage and subsequently help protect the cell from infection.
ncRNAs and chromosome structure
Telomerase is an RNP enzyme that adds specific DNA sequence repeats ("TTAGGG" in vertebrates) to telomeric regions, which are found at the ends of eukaryotic chromosomes. The telomeres contain condensed DNA material, giving stability to the chromosomes. The enzyme is a reverse transcriptase that carries Telomerase RNA, which is used as a template when it elongates telomeres, which are shortened after each replication cycle.
Xist (X-inactive-specific transcript) is a long ncRNA gene on the X chromosome of the placental mammals that acts as major effector of the X chromosome inactivation process forming Barr bodies. An antisense RNA, Tsix, is a negative regulator of Xist. X chromosomes lacking Tsix expression (and thus having high levels of Xist transcription) are inactivated more frequently than normal chromosomes. In drosophilids, which also use an XY sex-determination system, the roX (RNA on the X) RNAs are involved in dosage compensation.[35] Both Xist and roX operate by epigenetic regulation of transcription through the recruitment of histone-modifying enzymes.
Bifunctional RNA
Bifunctional RNAs, or dual-function RNAs, are RNAs that have two distinct functions.[36][37] The majority of the known bifunctional RNAs are mRNAs that encode both a protein and ncRNAs. However, a growing number of ncRNAs fall into two different ncRNA categories; e.g., H/ACA box snoRNA and miRNA.[38][39]
Two well known examples of bifunctional RNAs are SgrS RNA and RNAIII. However, a handful of other bifunctional RNAs are known to exist (e.g., steroid receptor activator/SRA,[40] VegT RNA,[41][42] Oskar RNA,[43] ENOD40,[44] p53 RNA[45] and SR1 RNA.[46] Bifunctional RNAs have recently been the subject of a special issue of Biochimie.[47]
ncRNAs and disease
As with proteins, mutations or imbalances in the ncRNA repertoire within the body can cause a variety of diseases.
Cancer
Many ncRNAs show abnormal expression patterns in cancerous tissues. These include miRNAs,[48] long mRNA-like ncRNAs,[49][50] GAS5,[51] SNORD50,[52] telomerase RNA and Y RNAs.[53] The miRNAs are involved in the large scale regulation of many protein coding genes,[54][55] the Y RNAs are important for the initiation of DNA replication,[28] telomerase RNA that serves as a primer for telomerase, an RNP that extends telomeric regions at chromosome ends (see telomeres and disease for more information). The direct function of the long mRNA-like ncRNAs is less clear.
Germ-line mutations in miR-16-1 and miR-15 primary precursors have been shown to be much more frequent in patients with chronic lymphocytic leukemia compared to control populations.[56][57]
It has been suggested that a rare SNP (rs11614913) that overlaps has-mir-196a2 has been found to be associated with non-small cell lung carcinoma.[58] Likewise, a screen of 17 miRNAs that have been predicted to regulate a number of breast cancer associated genes found variations in the microRNAs miR-17 and miR-30c-1of patients; these patients were noncarriers of BRCA1 or BRCA2 mutations, lending the possibility that familial breast cancer may be caused by variation in these miRNAs.[59]
The p53 tumor suppressor is arguably the most important player in preventing tumor formation and progression. The p53 protein functions as a transcription factor with a crucial role in orchestrating the cellular stress response. In addition to its crucial role in cancer, p53 has been implicated in other diseases including diabetes, cell death after ischemia, and various neurodegenerative diseases such as Huntington, Parkinson, and Alzheimer. Studies have suggested that p53 expression is subject to regulation by non-coding RNA.[4]
Prader–Willi syndrome
The deletion of the 48 copies of the C/D box snoRNA SNORD116 has been shown to be the primary cause of Prader–Willi syndrome.[60][61][62][63] Prader–Willi is a developmental disorder associated with over-eating and learning difficulties. SNORD116 has potential target sites within a number of protein-coding genes, and could have a role in regulating alternative splicing.[64]
Autism
The chromosomal locus containing the small nucleolar RNA SNORD115 gene cluster has been duplicated in approximately 5% of individuals with autistic traits.[65][66] A mouse model engineered to have a duplication of the SNORD115 cluster displays autistic-like behaviour.[67] A recent small study of post-mortem brain tissue demonstrated altered expression of long non-coding RNAs in the prefrontal cortex and cerebellum of autistic brains as compared to controls.[68]
Cartilage-hair hypoplasia
Mutations within RNase MRP have been shown to cause cartilage-hair hypoplasia, a disease associated with an array of symptoms such as short stature, sparse hair, skeletal abnormalities and a suppressed immune system that is frequent among Amish and Finnish.[69][70][71] The best characterised variant is an A-to-G transition at nucleotide 70 that is in a loop region two bases 5' of a conserved pseudoknot. However, many other mutations within RNase MRP also cause CHH.
Alzheimer's disease
The antisense RNA, BACE1-AS is transcribed from the opposite strand to BACE1 and is upregulated in patients with Alzheimer's disease.[72] BACE1-AS regulates the expression of BACE1 by increasing BACE1 mRNA stability and generating additional BACE1 through a post-transcriptional feed-forward mechanism. By the same mechanism it also raises concentrations of beta amyloid, the main constituent of senile plaques. BACE1-AS concentrations are elevated in subjects with Alzheimer's disease and in amyloid precursor protein transgenic mice.
miR-96 and hearing loss
Variation within the seed region of mature miR-96 has been associated with autosomal dominant, progressive hearing loss in humans and mice. The homozygous mutant mice were profoundly deaf, showing no cochlear responses. Heterozygous mice and humans progressively lose the ability to hear.[73][74][75]
Distinction between functional RNA (fRNA) and ncRNA
Several publications[76][77][78] have started using the term functional RNA (fRNA), as opposed to ncRNA, to describe regions functional at the RNA level that may or may not be stand-alone RNA transcripts. According to this terminology, there exist fRNA (such as riboswitches, SECIS elements, and other cis-regulatory regions) that are not ncRNA. Yet the term fRNA could also include mRNA as this is RNA coding for protein and hence is functional. Additionally artificially evolved RNAs also fall under the fRNA umbrella term. Some publications[19] state that the terms ncRNA and fRNA are nearly synonymous, however others have pointed out that a large proportion of annotated ncRNAs likely have no function.[7]
See also
- Extracellular RNA
- List of RNAs
- Nucleic acid structure
- Rfam
- Riboswitch
- Ribozyme
- RNAs present in environmental samples
References
- ↑ Cheng J, Kapranov P, Drenkow J, Dike S, Brubaker S, Patel S, Long J, Stern D, Tammana H, Helt G, Sementchenko V, Piccolboni A, Bekiranov S, Bailey DK, Ganesh M, Ghosh S, Bell I, Gerhard DS, Gingeras TR; Kapranov; Drenkow; Dike; Brubaker; Patel; Long; Stern; Tammana; Helt; Sementchenko; Piccolboni; Bekiranov; Bailey; Ganesh; Ghosh; Bell; Gerhard; Gingeras (2005). "Transcriptional maps of 10 human chromosomes at 5-nucleotide resolution". Science 308 (5725): 1149–54. Bibcode:2005Sci...308.1149C. doi:10.1126/science.1108625. PMID 15790807.
- ↑ ENCODE Project Consortium; Birney, E; Stamatoyannopoulos, JA; Dutta, A; Guigó, R; Gingeras, TR; Margulies, EH; Weng, Z; Snyder, M; Dermitzakis, John A.; Thurman, Robert E.; Kuehn, Michael S.; Taylor, Christopher M.; Neph, Shane; Koch, Christoph M.; Asthana, Saurabh; Malhotra, Ankit; Adzhubei, Ivan; Greenbaum, Jason A.; Andrews, Robert M.; Flicek, Paul; Boyle, Patrick J.; Cao, Hua; Carter, Nigel P.; Clelland, Gayle K.; Davis, Sean; Day, Nathan; Dhami, Pawandeep; Dillon, Shane C.; et al. (2007). "Identification and analysis of functional elements in 1% of the human genome by the ENCODE pilot project". Nature 447 (7146): 799–816. Bibcode:2007Natur.447..799B. doi:10.1038/nature05874. PMC 2212820. PMID 17571346.
- ↑ Washietl S, Pedersen JS, Korbel JO, Stocsits C, Gruber AR, Hackermüller J, Hertel J, Lindemeyer M, Reiche K, Tanzer A, Ucla C, Wyss C, Antonarakis SE, Denoeud F, Lagarde J, Drenkow J, Kapranov P, Gingeras TR, Guigó R, Snyder M, Gerstein MB, Reymond A, Hofacker IL, Stadler PF; Pedersen; Korbel; Stocsits; Gruber; Hackermüller; Hertel; Lindemeyer; Reiche; Tanzer; Ucla; Wyss; Antonarakis; Denoeud; Lagarde; Drenkow; Kapranov; Gingeras; Guigó; Snyder; Gerstein; Reymond; Hofacker; Stadler (2007). "Structured RNAs in the ENCODE selected regions of the human genome". Genome Res 17 (6): 852–64. doi:10.1101/gr.5650707. PMC 1891344. PMID 17568003.
- 1 2 Morris, KV (editor) (2012). Non-coding RNAs and Epigenetic Regulation of Gene Expression: Drivers of Natural Selection. Caister Academic Press. ISBN 978-1-904455-94-3.
- ↑ van Bakel H, Nislow C, Blencowe BJ, Hughes TR; Nislow; Blencowe; Hughes (2010). Eddy, Sean R., ed. "Most "dark matter" transcripts are associated with known genes". PLoS Biol 8 (5): e1000371. doi:10.1371/journal.pbio.1000371. PMC 2872640. PMID 20502517.
- ↑ Hüttenhofer A, Schattner P, Polacek N; Schattner; Polacek (2005). "Non-coding RNAs: hope or hype?". Trends Genet 21 (5): 289–97. doi:10.1016/j.tig.2005.03.007. PMID 15851066.
- 1 2 Palazzo, Alexander F.; Lee, Eliza S. (2015). "Non-coding RNA: what is functional and what is junk?". Frontiers in Genetics 6: 2. doi:10.3389/fgene.2015.00002. ISSN 1664-8021. PMID 25674102.
- ↑ Dahm R (February 2005). "Friedrich Miescher and the discovery of DNA". Dev. Biol. 278 (2): 274–88. doi:10.1016/j.ydbio.2004.11.028. PMID 15680349.
- ↑ Caspersson T, Schultz J; Schultz (1939). "Pentose nucleotides in the cytoplasm of growing tissues". Nature 143 (3623): 602–3. Bibcode:1939Natur.143..602C. doi:10.1038/143602c0.
- ↑ CRICK FH (1958). "On protein synthesis". Symp. Soc. Exp. Biol. 12: 138–63. PMID 13580867.
- 1 2 HOLLEY RW, APGAR J, EVERETT GA; et al. (March 1965). "STRUCTURE OF A RIBONUCLEIC ACID". Science 147 (3664): 1462–5. Bibcode:1965Sci...147.1462H. doi:10.1126/science.147.3664.1462. PMID 14263761.
- 1 2 "The Nobel Prize in Physiology or Medicine 1968". Nobel Foundation. Retrieved 2007-07-28.
- ↑ Madison JT, Everett GA, Kung H; Everett; Kung (1966). "Nucleotide sequence of a yeast tyrosine transfer RNA". Science 153 (3735): 531–4. Bibcode:1966Sci...153..531M. doi:10.1126/science.153.3735.531. PMID 5938777.
- ↑ Zachau HG, Dütting D, Feldmann H, Melchers F, Karau W; Dütting; Feldmann; Melchers; Karau (1966). "Serine specific transfer ribonucleic acids. XIV. Comparison of nucleotide sequences and secondary structure models". Cold Spring Harb. Symp. Quant. Biol. 31: 417–24. doi:10.1101/SQB.1966.031.01.054. PMID 5237198.
- ↑ Dudock BS, Katz G, Taylor EK, Holley RW; Katz; Taylor; Holley (March 1969). "Primary structure of wheat germ phenylalanine transfer RNA". Proc. Natl. Acad. Sci. U.S.A. 62 (3): 941–5. Bibcode:1969PNAS...62..941D. doi:10.1073/pnas.62.3.941. PMC 223689. PMID 5257014.
- ↑ Cramer F, Doepner H, Haar F VD, Schlimme E, Seidel H; Doepner; Haar f; Schlimme; Seidel (December 1968). "On the conformation of transfer RNA". Proc. Natl. Acad. Sci. U.S.A. 61 (4): 1384–91. Bibcode:1968PNAS...61.1384C. doi:10.1073/pnas.61.4.1384. PMC 225267. PMID 4884685.
- ↑ Ladner JE, Jack A, Robertus JD; et al. (November 1975). "Structure of yeast phenylalanine transfer RNA at 2.5 A resolution". Proc. Natl. Acad. Sci. U.S.A. 72 (11): 4414–8. Bibcode:1975PNAS...72.4414L. doi:10.1073/pnas.72.11.4414. PMC 388732. PMID 1105583.
- ↑ Kim SH, Quigley GJ, Suddath FL; et al. (1973). "Three-dimensional structure of yeast phenylalanine transfer RNA: folding of the polynucleotide chain". Science 179 (4070): 285–8. Bibcode:1973Sci...179..285K. doi:10.1126/science.179.4070.285. PMID 4566654.
- 1 2 Eddy SR (December 2001). "Non-coding RNA genes and the modern RNA world". Nat. Rev. Genet. 2 (12): 919–29. doi:10.1038/35103511. PMID 11733745.
- ↑ Daneholt, Bertil. "Advanced Information: RNA interference". The Nobel Prize in Physiology or Medicine 2006. Archived from the original on 2007-01-20. Retrieved 2007-01-25.
- ↑ Jeffares DC, Poole AM, Penny D; Poole; Penny (1998). "Relics from the RNA world". J Mol Evol 46 (1): 18–36. doi:10.1007/PL00006280. PMID 9419222.
- ↑ Poole AM, Jeffares DC, Penny D; Jeffares; Penny (1998). "The path from the RNA world". J Mol Evol 46 (1): 1–17. doi:10.1007/PL00006275. PMID 9419221.
- ↑ Poole A, Jeffares D, Penny D; Jeffares; Penny (1999). "Early evolution: prokaryotes, the new kids on the block". BioEssays 21 (10): 880–9. doi:10.1002/(SICI)1521-1878(199910)21:10<880::AID-BIES11>3.0.CO;2-P. PMID 10497339.
- ↑ Ban N, Nissen P, Hansen J, Moore P, Steitz T; Nissen; Hansen; Moore; Steitz (2000). "The complete atomic structure of the large ribosomal subunit at 2.4 ångström resolution". Science 289 (5481): 905–20. Bibcode:2000Sci...289..905B. doi:10.1126/science.289.5481.905. PMID 10937989.
- ↑ Zhu Y, Stribinskis V, Ramos KS, Li Y; Stribinskis; Ramos; Li (2006). "Sequence analysis of RNase MRP RNA reveals its origination from eukaryotic RNase P RNA". RNA 12 (5): 699–706. doi:10.1261/rna.2284906. PMC 1440897. PMID 16540690.
- ↑ Kishore S, Stamm S; Stamm (2006). "The snoRNA HBII-52 regulates alternative splicing of the serotonin receptor 2C". Science 311 (5758): 230–231. Bibcode:2006Sci...311..230K. doi:10.1126/science.1118265. PMID 16357227.
- ↑ Stein, AJ; Fuchs G; Fu C; Wolin SL; Reinisch KM (2005). "Structural insights into RNA quality control: The Ro autoantigen binds misfolded RNAs via its central cavity". Cell 121 (4): 529–537. doi:10.1016/j.cell.2005.03.009. PMC 1769319. PMID 15907467.
- 1 2 Christov CP, Gardiner TJ, Szüts D, Krude T; Gardiner; Szüts; Krude (2006). "Functional Requirement of Noncoding Y RNAs for Human Chromosomal DNA Replication". Mol. Cell. Biol. 26 (18): 6993–7004. doi:10.1128/MCB.01060-06. PMC 1592862. PMID 16943439.
- ↑ Zhang, AT; Langley, AR; Christov, CP; Kheir, E; Shafee, T; Gardiner, TJ; Krude, T (Jun 15, 2011). "Dynamic interaction of Y RNAs with chromatin and initiation proteins during human DNA replication.". Journal of Cell Science 124 (Pt 12): 2058–69. doi:10.1242/jcs.086561. PMC 3104036. PMID 21610089.
- ↑ Hall, Adam E.; Turnbull, Carly; Dalmay, Tamas (2013). "Y RNAs: recent developments". Biomolecular Concepts 4 (2): 103–110. doi:10.1515/bmc-2012-0050.
- ↑ Lerner, MR; Boyle JA; Hardin JA; Steitz JA (1981). "Two novel classes of small ribonucleoproteins detected by antibodies associated with lupus erythematosus". Science 211 (4480): 400–402. Bibcode:1981Sci...211..400L. doi:10.1126/science.6164096. PMID 6164096.
- ↑ Reiner R, Ben-Asouli Y, Krilovetzky I, Jarrous N; Ben-Asouli; Krilovetzky; Jarrous (2006). "A role for the catalytic ribonucleoprotein RNase P in RNA polymerase III transcription". Genes Dev 20 (12): 1621–35. doi:10.1101/gad.386706. PMC 1482482. PMID 16778078.
- ↑ Espinoza CA, Allen TA, Hieb AR, Kugel JF, Goodrich JA; Allen; Hieb; Kugel; Goodrich (2004). "B2 RNA binds directly to RNA polymerase II to repress transcript synthesis". Nat Struct Mol Biol 11 (9): 822–9. doi:10.1038/nsmb812. PMID 15300239.
- ↑ Hirota K, Miyoshi T, Kugou K, Hoffman CS, Shibata T, Ohta K; Miyoshi; Kugou; Hoffman; Shibata; Ohta (2008). "Stepwise chromatin remodelling by a cascade of transcription initiation of non-coding RNAs". Nature 456 (7218): 130–4. Bibcode:2008Natur.456..130H. doi:10.1038/nature07348. PMID 18820678.
- ↑ Park Y, Kelley RL, Oh H, Kuroda MI, Meller VH; Kelley; Oh; Kuroda; Meller (2002). "Extent of chromatin spreading determined by roX RNA recruitment of MSL proteins". Science 298 (5598): 1620–3. Bibcode:2002Sci...298.1620P. doi:10.1126/science.1076686. PMID 12446910.
- ↑ Wadler CS, Vanderpool CK; Vanderpool (2007). "A dual function for a bacterial small RNA: SgrS performs base pairing-dependent regulation and encodes a functional polypeptide". Proc Natl Acad Sci USA 104 (51): 20454–9. Bibcode:2007PNAS..10420454W. doi:10.1073/pnas.0708102104. PMC 2154452. PMID 18042713.
- ↑ Dinger ME, Pang KC, Mercer TR, Mattick JS; Pang; Mercer; Mattick (2008). McEntyre, Johanna, ed. "Differentiating protein-coding and noncoding RNA: challenges and ambiguities". PLoS Comput Biol 4 (11): e1000176. Bibcode:2008PLSCB...4E0176D. doi:10.1371/journal.pcbi.1000176. PMC 2518207. PMID 19043537.
- ↑ Saraiya AA, Wang CC; Wang (2008). Goldberg, Daniel Eliot, ed. "snoRNA, a novel precursor of microRNA in Giardia lamblia". PLoS Pathog 4 (11): e1000224. doi:10.1371/journal.ppat.1000224. PMC 2583053. PMID 19043559.
- ↑ Ender C, Krek A, Friedländer MR, Beitzinger M, Weinmann L, Chen W, Pfeffer S, Rajewsky N, Meister G; Krek; Friedländer; Beitzinger; Weinmann; Chen; Pfeffer; Rajewsky; Meister (2008). "A human snoRNA with microRNA-like functions". Mol Cell 32 (4): 519–28. doi:10.1016/j.molcel.2008.10.017. PMID 19026782.
- ↑ Leygue E (2007). "Steroid receptor RNA activator (SRA1): unusual bifaceted gene products with suspected relevance to breast cancer". Nucl Recept Signal 5: e006. doi:10.1621/nrs.05006. PMC 1948073. PMID 17710122.
- ↑ Zhang J, King ML; King (1996). "Xenopus VegT RNA is localized to the vegetal cortex during oogenesis and encodes a novel T-box transcription factor involved in mesodermal patterning". Development 122 (12): 4119–29. PMID 9012531.
- ↑ Kloc M, Wilk K, Vargas D, Shirato Y, Bilinski S, Etkin LD; Wilk; Vargas; Shirato; Bilinski; Etkin (2005). "Potential structural role of non-coding and coding RNAs in the organization of the cytoskeleton at the vegetal cortex of Xenopus oocytes". Development 132 (15): 3445–57. doi:10.1242/dev.01919. PMID 16000384.
- ↑ Jenny A, Hachet O, Závorszky P, Cyrklaff A, Weston MD, Johnston DS, Erdélyi M, Ephrussi A; Hachet; Závorszky; Cyrklaff; Weston; Johnston; Erdélyi; Ephrussi (2006). "A translation-independent role of oskar RNA in early Drosophila oogenesis". Development 133 (15): 2827–33. doi:10.1242/dev.02456. PMID 16835436.
- ↑ Gultyaev AP, Roussis A; Roussis (2007). "Identification of conserved secondary structures and expansion segments in enod40 RNAs reveals new enod40 homologues in plants". Nucleic Acids Res 35 (9): 3144–52. doi:10.1093/nar/gkm173. PMC 1888808. PMID 17452360.
- ↑ Candeias MM, Malbert-Colas L, Powell DJ, Daskalogianni C, Maslon MM, Naski N, Bourougaa K, Calvo F, Fahraeus R; Malbert-Colas; Powell; Daskalogianni; Maslon; Naski; Bourougaa; Calvo; Fåhraeus (2008). "p53 mRNA controls p53 activity by managing Mdm2 functions". Nature Cell Biology 10 (9): 1098–1105. doi:10.1038/ncb1770. PMID 19160491.
- ↑ Gimpel, M; Preis, H; Barth, E; Gramzow, L; Brantl, S (Oct 13, 2012). "SR1--a small RNA with two remarkably conserved functions". Nucleic Acids Research 40 (22): 11659–72. doi:10.1093/nar/gks895. PMC 3526287. PMID 23034808.
- ↑ Francastel, Claire; Hubé, Florent (2011). "Coding or non-coding: Need they be exclusive?". Biochimie 93 (11): vi–vii. doi:10.1016/S0300-9084(11)00322-1. PMID 21963143.
- ↑ Mraz, M.; Pospisilova, S. (2012). "MicroRNAs in chronic lymphocytic leukemia: From causality to associations and back". Expert Review of Hematology 5 (6): 579–581. doi:10.1586/ehm.12.54. PMID 23216588.
- ↑ Pibouin L, Villaudy J, Ferbus D, Muleris M, Prospéri MT, Remvikos Y, Goubin G; Villaudy; Ferbus; Muleris; Prospéri; Remvikos; Goubin (2002). "Cloning of the mRNA of overexpression in colon carcinoma-1: a sequence overexpressed in a subset of colon carcinomas". Cancer Genet Cytogenet 133 (1): 55–60. doi:10.1016/S0165-4608(01)00634-3. PMID 11890990.
- ↑ Fu X, Ravindranath L, Tran N, Petrovics G, Srivastava S; Ravindranath; Tran; Petrovics; Srivastava (2006). "Regulation of apoptosis by a prostate-specific and prostate cancer-associated noncoding gene, PCGEM1". DNA Cell Biol 25 (3): 135–41. doi:10.1089/dna.2006.25.135. PMID 16569192.
- ↑ Mourtada-Maarabouni M, Pickard MR, Hedge VL, Farzaneh F, Williams GT; Pickard; Hedge; Farzaneh; Williams (2009). "GAS5, a non-protein-coding RNA, controls apoptosis and is downregulated in breast cancer". Oncogene 28 (2): 195–208. doi:10.1038/onc.2008.373. PMID 18836484.
- ↑ Dong XY, Guo P, Boyd J, Sun X, Li Q, Zhou W, Dong JT; Guo; Boyd; Sun; Li; Zhou; Dong (2009). "Implication of snoRNA U50 in human breast cancer". J Genet Genomics 36 (8): 447–54. doi:10.1016/S1673-8527(08)60134-4. PMC 2854654. PMID 19683667.
- ↑ Christov CP, Trivier E, Krude T; Trivier; Krude (2008). "Noncoding human Y RNAs are overexpressed in tumours and required for cell proliferation". Br J Cancer 98 (5): 981–8. doi:10.1038/sj.bjc.6604254. PMC 2266855. PMID 18283318.
- ↑ Farh KK, Grimson A, Jan C, Lewis BP, Johnston WK, Lim LP, Burge CB, Bartel DP; Grimson; Jan; Lewis; Johnston; Lim; Burge; Bartel (2005). "The widespread impact of mammalian MicroRNAs on mRNA repression and evolution". Science 310 (5755): 1817–21. Bibcode:2005Sci...310.1817F. doi:10.1126/science.1121158. PMID 16308420.
- ↑ Lim LP, Lau NC, Garrett-Engele P, Grimson A, Schelter JM, Castle J, Bartel DP, Linsley PS, Johnson JM; Lau; Garrett-Engele; Grimson; Schelter; Castle; Bartel; Linsley; Johnson (2005). "Microarray analysis shows that some microRNAs downregulate large numbers of target mRNAs". Nature 433 (7027): 769–73. Bibcode:2005Natur.433..769L. doi:10.1038/nature03315. PMID 15685193.
- ↑ Calin GA, Ferracin M, Cimmino A; et al. (October 2005). "A MicroRNA signature associated with prognosis and progression in chronic lymphocytic leukemia". N. Engl. J. Med. 353 (17): 1793–801. doi:10.1056/NEJMoa050995. PMID 16251535.
- ↑ Calin, GA; Dumitru CD, Shimizu M, Bichi R, Zupo S, Noch E, Aldler H, Rattan S, Keating M, Rai K, Rassenti L, Kipps T, Negrini M, Bullrich F, Croce CM; Shimizu, Masayoshi; Bichi, Roberta; Zupo, Simona; Noch, Evan; Aldler, Hansjuerg; Rattan, Sashi; Keating, Michael; Rai, Kanti; Rassenti, Laura; Kipps, Thomas; Negrini, Massimo; Bullrich, Florencia; Croce, Carlo M. (2002). "Frequent deletions and down-regulation of micro-RNA genes miR15 and miR16 at 13q14 in chronic lymphocytic leukemia". Proc Natl Acad Sci USA 99 (24): 15524–15529. Bibcode:2002PNAS...9915524C. doi:10.1073/pnas.242606799. PMC 137750. PMID 12434020.
- ↑ Hu Z, Chen J, Tian T, Zhou X, Gu H, Xu L, Zeng Y, Miao R, Jin G, Ma H, Chen Y, Shen H; Chen; Tian; Zhou; Gu; Xu; Zeng; Miao; Jin; Ma; Chen; Shen (2008). "Genetic variants of miRNA sequences and non-small cell lung cancer survival". J Clin Invest 118 (7): 2600–8. doi:10.1172/JCI34934. PMC 2402113. PMID 18521189.
- ↑ Shen J, Ambrosone CB, Zhao H; Ambrosone; Zhao (2009). "Novel genetic variants in microRNA genes and familial breast cancer". Int J Cancer 124 (5): 1178–82. doi:10.1002/ijc.24008. PMID 19048628.
- ↑ Sahoo T, del Gaudio D, German JR, Shinawi M, Peters SU, Person RE, Garnica A, Cheung SW, Beaudet AL; Del Gaudio; German; Shinawi; Peters; Person; Garnica; Cheung; Beaudet (2008). "Prader–Willi phenotype caused by paternal deficiency for the HBII-85 C/D box small nucleolar RNA cluster". Nat Genet 40 (6): 719–21. doi:10.1038/ng.158. PMC 2705197. PMID 18500341.
- ↑ Skryabin BV, Gubar LV, Seeger B, Pfeiffer J, Handel S, Robeck T, Karpova E, Rozhdestvensky TS, Brosius J; Gubar; Seeger; Pfeiffer; Handel; Robeck; Karpova; Rozhdestvensky; Brosius (2007). "Deletion of the MBII-85 snoRNA gene cluster in mice results in postnatal growth retardation". PLoS Genet 3 (12): e235. doi:10.1371/journal.pgen.0030235. PMC 2323313. PMID 18166085.
- ↑ Ding F, Li HH, Zhang S, Solomon NM, Camper SA, Cohen P, Francke U; Li; Zhang; Solomon; Camper; Cohen; Francke (2008). Akbarian, Schahram, ed. "SnoRNA Snord116 (Pwcr1/MBII-85) deletion causes growth deficiency and hyperphagia in mice". PLoS ONE 3 (3): e1709. Bibcode:2008PLoSO...3.1709D. doi:10.1371/journal.pone.0001709. PMC 2248623. PMID 18320030.
- ↑ Ding F, Prints Y, Dhar MS, Johnson DK, Garnacho-Montero C, Nicholls RD, Francke U; Prints; Dhar; Johnson; Garnacho-Montero; Nicholls; Francke (2005). "Lack of Pwcr1/MBII-85 snoRNA is critical for neonatal lethality in Prader–Willi syndrome mouse models". Mamm Genome 16 (6): 424–31. doi:10.1007/s00335-005-2460-2. PMID 16075369.
- ↑ Bazeley PS, Shepelev V, Talebizadeh Z, Butler MG, Fedorova L, Filatov V, Fedorov A; Shepelev; Talebizadeh; Butler; Fedorova; Filatov; Fedorov (2008). "snoTARGET shows that human orphan snoRNA targets locate close to alternative splice junctions". Gene 408 (1–2): 172–9. doi:10.1016/j.gene.2007.10.037. PMID 18160232.
- ↑ Bolton PF, Veltman MW, Weisblatt E; et al. (September 2004). "Chromosome 15q11-13 abnormalities and other medical conditions in individuals with autism spectrum disorders". Psychiatr. Genet. 14 (3): 131–7. doi:10.1097/00041444-200409000-00002. PMID 15318025.
- ↑ Cook EH, Scherer SW; Scherer (October 2008). "Copy-number variations associated with neuropsychiatric conditions". Nature 455 (7215): 919–23. Bibcode:2008Natur.455..919C. doi:10.1038/nature07458. PMID 18923514.
- ↑ Nakatani J, Tamada K, Hatanaka F; et al. (June 2009). "Abnormal behavior in a chromosome-engineered mouse model for human 15q11-13 duplication seen in autism". Cell 137 (7): 1235–46. doi:10.1016/j.cell.2009.04.024. PMC 3710970. PMID 19563756.
- ↑ Ziats, Mark N.; Rennert, Owen M. (2012). "Aberrant Expression of Long Noncoding RNAs in Autistic Brain". Journal of Molecular Neuroscience 49 (3): 589–93. doi:10.1007/s12031-012-9880-8. PMC 3566384. PMID 22949041.
- ↑ Ridanpää M, van Eenennaam H, Pelin K, Chadwick R, Johnson C, Yuan B, vanVenrooij W, Pruijn G, Salmela R, Rockas S, Mäkitie O, Kaitila I, de la Chapelle A; Van Eenennaam; Pelin; Chadwick; Johnson; Yuan; Vanvenrooij; Pruijn; Salmela; Rockas; Mäkitie; Kaitila; de la Chapelle (2001). "Mutations in the RNA component of RNase MRP cause a pleiotropic human disease, cartilage-hair hypoplasia". Cell 104 (2): 195–203. doi:10.1016/S0092-8674(01)00205-7. PMID 11207361.
- ↑ Martin AN, Li Y; Li (2007). "RNase MRP RNA and human genetic diseases". Cell Res 17 (3): 219–26. doi:10.1038/sj.cr.7310120. PMID 17189938.
- ↑ Kavadas FD, Giliani S, Gu Y, Mazzolari E, Bates A, Pegoiani E, Roifman CM, Notarangelo LD; Giliani; Gu; Mazzolari; Bates; Pegoiani; Roifman; Notarangelo (2008). "Variability of clinical and laboratory features among patients with ribonuclease mitochondrial RNA processing endoribonuclease gene mutations". J Allergy Clin Immunol 122 (6): 1178–84. doi:10.1016/j.jaci.2008.07.036. PMID 18804272.
- ↑ Faghihi MA, Modarresi F, Khalil AM, Wood DE, Sahagan BG, Morgan TE, Finch CE, St Laurent G, Kenny PJ, Wahlestedt C; Modarresi; Khalil; Wood; Sahagan; Morgan; Finch; St Laurent g; Kenny; Wahlestedt (2008). "Expression of a noncoding RNA is elevated in Alzheimer's disease and drives rapid feed-forward regulation of beta-secretase". Nat Med 14 (7): 723–30. doi:10.1038/nm1784. PMC 2826895. PMID 18587408.
- ↑ Mencía A, Modamio-Høybjør S, Redshaw N, Morín M, Mayo-Merino F, Olavarrieta L, Aguirre LA, del Castillo I, Steel KP, Dalmay T, Moreno F, Moreno-Pelayo MA; Modamio-Høybjør; Redshaw; Morín; Mayo-Merino; Olavarrieta; Aguirre; Del Castillo; Steel; Dalmay; Moreno; Moreno-Pelayo (2009). "Mutations in the seed region of human miR-96 are responsible for nonsyndromic progressive hearing loss". Nat Genet 41 (5): 609–13. doi:10.1038/ng.355. PMID 19363479.
- ↑ Lewis MA, Quint E, Glazier AM, Fuchs H, De Angelis MH, Langford C, van Dongen S, Abreu-Goodger C, Piipari M, Redshaw N, Dalmay T, Moreno-Pelayo MA, Enright AJ, Steel KP; Quint; Glazier; Fuchs; De Angelis; Langford; Van Dongen; Abreu-Goodger; Piipari; Redshaw; Dalmay; Moreno-Pelayo; Enright; Steel (2009). "An ENU-induced mutation of miR-96 associated with progressive hearing loss in mice". Nat Genet 41 (5): 614–8. doi:10.1038/ng.369. PMC 2705913. PMID 19363478.
- ↑ Soukup GA (2009). "Little but loud: Small RNAs have a resounding affect on ear development". Brain Res 1277: 104–14. doi:10.1016/j.brainres.2009.02.027. PMC 2700218. PMID 19245798.
- ↑ Richard J. Carter, Inna Dubchak, Stephen R. Holbrook; Dubchak; Holbrook (2001). "A computational approach to identify genes for functional RNAs in genomic sequences". Nucleic Acids Research 29 (19): 3928–3938. doi:10.1093/nar/29.19.3928 (inactive 2015-02-01). PMC 60242. PMID 11574674.
- ↑ Jakob Skou Pedersen, Gill Bejerano, Adam Siepel, Kate Rosenbloom, Kerstin Lindblad-Toh, Eric S. Lander, Jim Kent, Webb Miller, David Haussler; Bejerano; Siepel; Rosenbloom; Lindblad-Toh; Lander; Kent; Miller; Haussler (2006). "Identification and Classification of Conserved RNA Secondary Structures in the Human Genome". PLOS Computational Biology 2 (4): e33. Bibcode:2006PLSCB...2...33P. doi:10.1371/journal.pcbi.0020033. PMC 1440920. PMID 16628248.
- ↑ Tomas Babak, Benjamin J Blencowe, Timothy R Hughes; Horspool; Brown; Tcherepanov; Upton (2007). "Considerations in the identification of functional RNA structural elements in genomic alignments". BMC Bioinformatics 8: 33. doi:10.1186/1471-2105-8-21. PMC 1783863. PMID 17244370.
External links
![]() |
Wikimedia Commons has media related to Non-coding RNA. |
- Comprehensive database of mammalian ncRNAs
- The Rfam Database — a curated list of hundreds of families of related ncRNAs
- NONCODE.org — a free database of all kinds of noncoding RNAs (except tRNAs and rRNAs)
- RNAcon Prediction and classification of ncRNA BMC Genomics 2014, 15:127
- ENCODE threads explorer Non-coding RNA characterization. Nature (journal)
- The Non-coding RNA Databases Resource (NRDR) — a curated source of data related to over non-coding RNA databases available over the internet
|