Inverse Warburg Effect
The term Inverse Warburg Effect was introduced by Lloyd Demetrius and colleagues (1,2) to describe the metabolic reprogramming observed in cortical regions of the brain during the prodromal phase of Alzheimer’s disease (3,4) and Parkinson’s disease (5,6). This reprogramming refers to the up regulation of oxidative phosphorylation (OxPhos) in mitochondria of certain neurons – a process which induces a hypermetabolic state in these neurons in an age dependent manner. The aging process is driven, in part, by an increase in molecular disorder and a concomitant decrease in enzymatic activity (7) leading to an impairment in the capacity of cells to appropriate energy from the external environment and to convert this energy into biosynthetic work. Consequently, the capacity of neurons to convert food derived fuel sources into ATP and to use this energy to maintain neuronal viability is susceptible to decline with age. However, age-induced mitochondrial dysfunction will induce a compensatory increase in OxPhos in certain sub-populations of neurons in order to maintain adequate energy production, and thereby ensure synaptic function and cell survival (8). Within neurons of the aging brain an increase in OxPhos activity in intact mitochondria, and a redistribution of these mitochondria to sites which require high energy production has been documented (9). Lloyd Demetrius and colleagues (1,2) have proposed this compensatory change as the fundamental and initiating event in the progression towards neurodegenerative diseases.

The Inverse Warburg effect is postulated as the primary event in a cascade of metabolic changes (The Inverse Warburg Hypothesis) which ultimately leads to neuronal loss. The main energy currency in living organisms is ATP which must be continually generated to maintain cell viability. The energy is derived from two types of processes – OxPhos which provides ~80% of the total energy, and glycolysis which contributes the remaining ~ 20% (10) (Figure 1).

In oncology, most cancer cells predominantly produce energy by the up-regulation of glycolysis. Malignant, rapidly growing tumor cells have glycolytic rates higher than those of their normal tissue of origin (Figure 2). This mode of metabolic reprogramming is now called the Warburg effect, after the Nobel Laureate, Otto Warburg, who proposed that up-regulation of glycolysis is a primary initiating event in the progression towards cancer (11). Elevated glycolysis fuels anabolic processes that contribute to the rapid growth and cellular division associated with cancer (12).
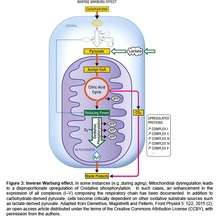
Epidemilogical studies have shown that cancer is inversely comorbid with both Alzheimer’s disease (AD) and Parkinson’s disease (PD) (13,14). The molecular and bioenergetic basis for this inverse relation can be explained by invoking the Warburg effect as the fundamental cause of cancer and the Inverse Warburg effect as the fundamental cause of AD and PD (15). Elevated glycolysis in cancer cells leads to the heightened production of lactate, the end product of glycolysis. Lactate has emerged as a key cerebral energy source that can provide energy via OxPhos (16). Therefore, neurons with elevated OxPhos become critically dependent on an oxidative substrate, such as lactate-derived pyruvate, a key feature of the Inverse Warburg Effect (Figure 3). Elevated lactate production, associated with cancer, may therefore fuel the heightened metabolic demands of neurons within the brains of elderly patients. A hypermetabolic state, as predicted by the Inverse Warburg Effect, has been detected in the brains of individuals during the presymptomatic phase of AD and PD (3-6). However, the inability to switch to the Warburg effect not only restricts the development of cancer, but also curtails a potential source of lactate for hypermetabolic neurons within the brain. Under this scenario, neurons are starved and neurodegeneration ensues. Thus the Inverse Warburg Effect provides a holistic model which defines the role of aging as a risk factor for AD and PD, and also provides an explanation for the inverse relationship between cancer and neurodegenerative disorders.
References
1. Demetrius LA & Simon DK. An inverse-Warburg effect and the origin of Alzheimer's disease. Biogerontology. 13(6):583-94 (2012).
2. Demetrius LA, Magistretti PJ & Pellerin L. Alzheimer's disease: the amyloid hypothesis and the Inverse Warburg effect. Front Physiol 5: 522 (2015).
3. Ashraf A, Fan Z, Brooks DJ, Edison P. Cortical hypermetabolism in MCI subjects: a compensatory mechanism? Eur J Nucl Med Mol Imaging. 42(3):447-58 (2015).
4. Ossenkoppele R, Madison C, Oh H, Wirth M, van Berckel BN, Jagust WJ. Is verbal episodic memory in elderly with amyloid deposits preserved through altered neuronal function? Cereb Cortex. 24(8):2210-8 (2014).
5. Tang CS, Poston KL, Dhawan V & Eidelberg D. Abnormalities in Metabolic Network Activity Precede the Onset of Motor Symptoms in Parkinson's Disease. J Neurosci, 30(3): 1049-1056 (2010).
6. Pacelli C, Giguère N, Bourque M, Lévesque M,. Slack RS & Trudeau L. Elevated Mitochondrial Bioenergetics and Axonal Arborization Size Are Key Contributors to the Vulnerability of Dopamine Neurons. Current Biology, 25(18) R:797 (2015)
7. Hayflick,L. Biological aging is no longer an unsolved problem. Ann. N.Y. Acad. Sci. 1100: 1–13 (2007).
8. Forester BP, Berlow YA, Harper DG, Jensen JE, Lange N, Froimowitz MP, Ravichandran C, Iosifescu DV, Lukas SE, Renshaw PF, Cohen BM. Age-related changes in brain energetics and phospholipid metabolism. NMR Biomed. 23(3):242-50 (2010)
9. Chang DT, Reynolds IJ. Mitochondrial trafficking and morphology in healthy and injured neurons. Prog Neurobiol. 80(5):241-68 (2006)
10. Jose C, Bellance N, Rossignol R. Choosing between glycolysis and oxidative phosphorylation: A tumor's dilemma? Biochimica et Biophysica Acta (BBA) – Bioenergetics. 1807( 6) 552–561 (2011).
11. Warburg O. On the origin of cancer cells. Science 123 (3191): 309–314 (1956)
12. Vander Heiden MG, Cantley LC, Thompson CB, Understanding the Warburg Effect: The Metabolic Requirements of Cell Proliferation. Science. 324(5930): 1029–1033 (2009).
13. Driver JA, Beiser A, Au R, Kreger BE, Splansky GL, Kurth T, Kiel DP, Lu KP, Seshadri S & Wolf PA. Inverse association between cancer and Alzheimer's disease: results from the Framingham Heart Study. BMJ. 344:e1442 (2012)
14. Beckera C, Brobert GP, Johansson S, d,. Jick SS & Meiera CR. Cancer risk in association with Parkinson disease: A population-based study. Parkinsonism Relat Disord. (3): 186–190 (2009).
15. Harris RA, Tindale L & Cumming RC. Age-dependent metabolic dysregulation in cancer and Alzheimer's disease. Biogerontology. 15(6):559-77 (2014).
16. Zilberter,Y., Zilberter,T.,and Bregestovski,P. Neuronal activity in vitro and the in vivo reality: the role of energy homeostasis. Trends Pharmacol.Sci. 31, 394–401 (2010)