Thermal shift assay
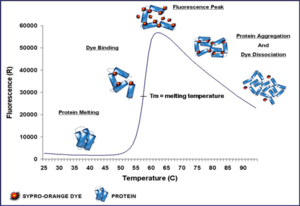
A thermal shift assay, also called differential scanning fluorimetry (DSF) is a thermal-denaturation assay that measures the thermal stability of a target protein and a subsequent increase in protein melting temperature upon binding of a ligand to the protein.[1]
The binding of low molecular weight ligands can increase the thermal stability of a protein, as described by Koshland (1958)[2] and Linderstrom-Lang and Schellman (1959).[3] The thermal stability change is measured by performing a thermal denaturation curve in the presence of a fluorescent dye, such as Sypro Orange.[4] Sypro orange binds nonspecifically to hydrophobic surfaces, and water strongly quenches the fluorescence of Sypro Orange.[5] When the protein unfolds, the exposed hydrophobic surfaces bind the dye, resulting in an increase in fluorescence. The stability curve and its midpoint value (melting temperature, Tm) are obtained by gradually increasing the temperature to unfold the protein and measuring the fluorescence at each point. Curves are measured for protein only and protein + ligand, and ΔTm is calculated.
A fluorescence-based thermal shift assay can be performed on instruments that combine sample temperature control and dye fluorescence detection, such as readily available real-time polymerase chain reaction (RT-PCR) machines.[5] This assay allows high-throughput screening of ligands to the target protein and it is widely used in the early stages of drug discovery in the pharmaceutical industry, structural genomics efforts, and high-throughput protein engineering.[6]
Thermal shift assays can also be used to identify optimal protein buffer conditions. [7]
A related method to screen for protein buffer conditions is nanoDSF. It measures protein stability in thermal and chemical denaturation experiments by detecting changes in intrinsic tryptophan fluorescence upon unfolding.[8] [9]
A typical assay
- Materials: A fluorometer equipped with temperature control or similar instrumentation (RT-PCR machines); suitable fluorescent dye; a suitable assay plate, such as 96 well RT-PCR plate.[5]
- Compound solutions: Test ligands are prepared at a 50- to 100-fold concentrated solution, generally in the 10-100 mM range. For titration, a typical experimental protocol employs a set of 12 well, comprising 11 different concentrations of a test compound with a single negative control well.[5]
- Protein solution: Typically, target protein is diluted from a concentrated stock to a working concentration of ~0.5-5 μM protein with dye into a suitable assay buffer. The exact concentrations of protein and dye are defined by experimental assay development studies.[5]
- Centrifugation and oil dispense: A brief centrifugation (~1000 × g-force, 1 min) of the assay plate to mix compounds into the protein solution, 1-2 μl silicone oil to prevent the evaporation during heating is overlaid onto the solution, followed by an additional centrifugation step (~1000 × g-force, 1 min).[5]
- Instrumental set up: A typical temperature ramp rates range from 0.1-10 °C/min but generally in the range of 1 °C/min. The fluorescence in each well is measured at regular intervals, 0.2-1 °C/image, over a temperature range spanning the typical protein unfolding temperatures of 25-95 °C.[5]
References
- ↑ Niesen FH, Berglund H, Vedadi M (September 2007). "The use of differential scanning fluorimetry to detect ligand interactions that promote protein stability". Nat Protoc. 2 (9): 2212–2221. doi:10.1038/nprot.2007.321. PMID 17853878.
- ↑ Koshland DE (Fedrurary 1985). "Application of a Theory of Enzyme Specificity to Protein Synthesis". Proc Natl Acad Sci U S A. 44 (2): 98–104. doi:10.1073/pnas.44.2.98. PMC 335371. PMID 16590179. Check date values in:
|date=
(help) - ↑ Linderstrøm-Lang, K., and Schellman, J. A. (1959). "Protein structure and enzyme activity". The Enzymes. 1(2) 443-510.
- ↑ Lavinder, Jason; Sanjay B. Hari; Brandon J. Sullivan; Thomas J. Magliery (Mar 4, 2009). "High-throughput thermal scanning: a general, rapid dye-binding thermal shift screen for protein engineering". J Am Chem Soc. 131 (11): 3794–3795. doi:10.1021/ja8049063. PMC 2701314. PMID 19292479.
- ↑ 5.0 5.1 5.2 5.3 5.4 5.5 5.6 James K. Kranz, Celine Schalk-Hihi (2011). "Protein thermal shifts to identify low molecular weight fragments". Methods Enzymol. 493: 277–298. doi:10.1016/B978-0-12-381274-2.00011-X. PMID 21371595.
- ↑ Ciulli A, Abell C. (December 2007). "Fragment-based approaches to enzyme inhibition.". Current Opinion in Biotechnology 18 (6): 489–96. doi:10.1016/j.copbio.2007.09.003. PMID 17959370.
- ↑ Reinhard L., Mayerhofer H., Geerlof A., Mueller-Dieckmann J., Weiss M.S. (January 2013). "Optimization of protein buffer cocktails using Thermofluor.". Acta Crystallogr Sect F Struct Biol Cryst Commun. 69 (2): 209–14. doi:10.1107/S1744309112051858. PMID 23385769.
- ↑ "NanoTemper Technologies GmbH: nanoDSF". nanotemper-technologies.com.
- ↑ "Genetic Engineering & Biotechnology News".