Microbiota
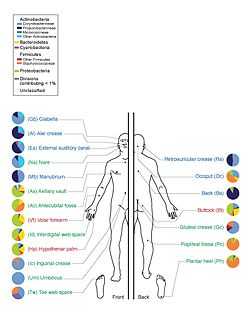
A microbiota is "the ecological community of commensal, symbiotic and pathogenic microorganisms that literally share our body space".[1][2] Joshua Lederberg coined the term, emphasising the importance of microorganisms inhabiting the human body in health and disease. Many scientific articles distinguish microbiome and microbiota to describe either the collective genomes of the microorganisms that reside in an environmental niche or the microorganisms themselves, respectively.[3][4][5] However, by the original definitions these terms are largely synonymous.
The human body contains over 10 times more microbial cells than human cells, although the entire microbiome only weighs about 200 grams (7.1 oz),[6][7] with some weight-estimates ranging as high as 3 pounds (approximately 48 ounces or 1,400 grams). Some regard the microbiome as a "newly discovered organ" since its existence was not generally recognized until the late 1990s and it is understood to have potentially overwhelming impact on human health.[8] Modern techniques for sequencing DNA have enabled researchers to find the majority of these microbes - the majority of them cannot be cultured in a lab using current techniques. The human microbiome may have a role in auto-immune diseases like diabetes, rheumatoid arthritis, muscular dystrophy, multiple sclerosis, fibromyalgia, and perhaps some cancers. A poor mix of microbes in the gut may also aggravate common obesity.[9][10][11] Since some of the microbes in the human body can modify the production of neurotransmitters known to occur in the brain, it may also relieve schizophrenia, depression, bipolar disorder and other neuro-chemical imbalances.[12]
The microbes being discussed are generally non-pathogenic (they do not cause disease unless they grow abnormally); they exist in harmony and symbiotically with their hosts.[13] Moreover, it has been stated that microbiome and host emerged as a unity along evolution by a process of integration.[14]
Introduction
All plants and animals, from protists to humans, live in close association with microbial organisms (see for example the human microbiome). Up until relatively recently, however, biologists have defined the interactions of plants and animals with the microbial world mostly in the context of disease states and of a relatively small number of symbiotic case studies. Organisms do not live in isolation, but have evolved in the context of complex communities. A number of advances have driven a change in the perception of microbiomes, including:
- the ability to perform genomic and gene expression analyses of single cells and even of entire microbial communities in the new disciplines of metagenomics and metatranscriptonomics
- massive databases making this information accessible to researchers across multiple disciplines
- methods of mathematical analysis that help researchers to make sense of complex data sets
Increasingly, biologists have come to appreciate that microbes make up an important part of an organism's phenotype, far beyond the occasional symbiotic case study.[15]
Pierre-Joseph van Beneden(1809-1894), a Belgian professor at the University of Louvain, developed the concept of commensalism during the nineteenth century. In his 1875 publication Animal Parasites and Messmates, Van Beneden presented 264 examples of commensalism. His conception was widely accepted by his contemporaries and commensalism has continued to be used as a concept right up to the present day: microbiome is clearly linked to commensalism.[16]
Case studies
There is a strengthening consensus among evolutionary biologists that one should not separate an organism's genes from the context of its resident microbes.
Studies in humans
- Community sequencing of total gut microbiota taken from obese and lean twins show substantial differences in their compositions. Total population sequences were analyzed to determine the levels of enzymes involved in carbohydrate, lipid, and amino acid metabolism. Obesity is associated with phylum-level differences in the microbiota, a significantly reduced bacterial diversity, and an increase in the population expression of enzymes which result in an increased efficiency of calorie harvest in the diets of the obese twins.[17]
- Type I diabetes is an autoimmune disease that is correlated with a multiplicity of predisposing factors, including an aberrant intestinal microbiota, a leaky intestinal mucosal barrier, and intrinsic differences in immune responsiveness. Various animal models for diabetes have shown a role for bacteria in the onset of the disease. Community DNA sequencing of intestinal flora comparing healthy and autoimmune children showed that autoimmune children had relatively unstable gut biomes with significantly decreased levels of species diversity, and the populations showed large scale replacement of Firmicutes species with Bacteroidetes species.[18]
- Human skin represents the most extensive organ of the human body, whose functions include protecting the body from pathogens, preventing loss of moisture, and participating in the regulation of body temperature. Considered as an ecosystem, the skin supports a range of microbial communities that live in distinct niches. Hair-covered scalp lies but a few inches from exposed neck, which in turn lies inches away from moist hairy underarms, but these niches are, at a microbial level, as distinct as a temperate forest would be compared with savanna and tropical rain forest. Studies characterizing the microbiota that inhabit these different niches are providing insights into the balance between skin health and disease.[19]
- Prevention of urogenital diseases in women depends on healthy vaginal microbiomes, but what is meant by "healthy" has not been understood. Community population studies using advanced sequencing methodologies (including pyrosequencing) are yielding insights into the range of microbial diversity in the human vagina. An unexpected finding was the prevalence of Prevotella species, which are known to positively affect the growth of Gardnerella vaginalis and Peptostreptococcus anaerobius, two species linked to bacterial vaginosis, by providing these disease-associated bacteria with key nutrients.[20]
- A proposal has been made to classify people by enterotype, based on the composition of the gut microbiome. By combining 22 newly sequenced fecal metagenomes of individuals from four countries with previously published data sets, three robust clusters were identified that are not nation or continent specific.[21][22]
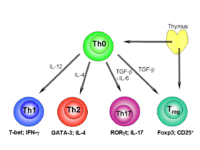
- The traditional view of the immune system is that it is a complex assembly of organs, tissues, cells and molecules that work together to eliminate pathogens. Modifications to this traditional view, that the immune system has evolved to control microbes, have come from the discovery that microbes coevolve with and exert control upon the immune system. It is known that germ-free animals possess an underdeveloped immune system. The biology of the T helper 17 cells (Th17) has generated interest due to their key role in inflammatory processes. Excessive amounts of the cell are thought to play a key role in autoimmune diseases such as multiple sclerosis, psoriasis, juvenile diabetes, rheumatoid arthritis, Crohn's disease, and autoimmune uveitis. It has been discovered that specific microbiota direct the differentiation of Th17 cells in the mucosa of the small intestine.[23]
Animal studies
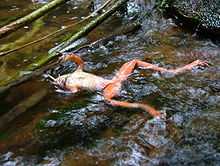
- A massive, worldwide decline in amphibian populations has been well-publicised. Habitat loss and over-exploitation account for part of the problem, but many other processes seem to be at work. The spread of the virulent fungal disease chytridiomycosis represents an enigma.[24] The ability of some species to coexist with the causative agent Batrachochytrium dendrobatidis appears to be due to the expression of antimicrobial skin peptides along with the presence of symbiotic microbes that benefit the host by resisting pathogen colonization or inhibiting their growth while being themselves resistant to high concentrations of antimicrobial skin peptides.[25]
- The bovine rumen harbors a complex microbiome that converts plant cell wall biomass into proteins, short chain fatty acids, and gases. Multiple species are involved in this conversion. Traditional methods of characterizing the microbial population, based on culture analysis, missed many of the participants in this process. Comparative metagenomic studies yielded the surprising result that individual steer had markedly different community structures, predicted phenotype, and metabolic potentials,[26] even though they were fed identical diets, were housed together, and were apparently functionally identical in their utilization of plant cell wall resources.
- Leaf-cutter ants form huge underground colonies with millions of workers, each colony harvesting hundreds of kilograms of leaves each year. Unable to digest the cellulose in the leaves directly, they maintain fungus gardens that are the colony's primary food source. The fungus itself does not digest cellulose. Instead, a microbial community containing a diversity of bacteria is responsible for cellulose digestion. Analysis of the microbial population's genomic content by community metagenome sequencing methods revealed the presence of many genes with a role in cellulose digestion. This microbiome's predicted carbohydrate-degrading enzyme profile is similar to that of the bovine rumen, but the species composition is almost entirely different.[27]
- Mice are the most used models for human disease. As more and more diseases are linked to dysfunctional microbiomes, mice have become the most studied organism in this regard. Mostly it is the gut microbiota that have been studied in relation to allergic airway disease, obesity, gastointesinal diseses and diabetes. Intriguingly, recent work has shown that perinatal shifting of microbiota through administration of low dose antibiotics can have long-lasting effects on future susceptibility to allergic airway disease.[28][29] These studies showed a remarkable link between the frequency of certain subsets of microbes and disease severity. In aggregate these studies suggest that the presence of specific microbes, early in postnatal life, play an instructive role in the development of future immune responses. Mechanistically, a recent study done on gnotobiotic mice described a method in which certain strains of gut bacteria were found to transmit a particular phenotype to recipient germ-free mice, identifying an unanticipated range of bacterial strains that promoted accumulation of colonic regulatory T cells, as well as strains that modulated mouse adiposity and cecal metabolite concentrations. This combinatorial approach enables a systems-level understanding of microbial contributions to human biology.[30] But also other mucoide tissues as lung and vagina have been studies in relation to diseses such as asthma, allergy and vaginosis [31]
Plant studies
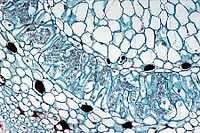
- Plants exhibit a broad range of relationships with symbiotic microorganisms, ranging from parasitism, in which the association is disadvantageous to the host organism, to mutualism, in which the association is beneficial to both, to commensalism, in which the symbiont benefits while the host is not affected. Exchange of nutrients between symbiotic partners is an important part of the relationship: it may be bidirectional or unidirectional, and it may be context dependent. The strategies for nutrient exchange are highly diverse. Oomycetes and fungi have, through convergent evolution, developed similar morphology and occupy similar ecological niches. They develop hyphae, filamentous structures that penetrate the host cell. In those cases where the association is mutualistic, the plant often exchanges hexose sugars for inorganic phosphate from the fungal symbiont. It is speculated that such associations, which are very ancient, may have aided plants when they first colonized land.[32][33]
- A huge range of bacterial symbionts colonize plants. Many of these are pathogenic, but others known as plant-growth promoting bacteria (PGPB) provide the host with essential services such as nitrogen fixation, solubilization of minerals such as phosphorus, synthesis of plant hormones, direct enhancement of mineral uptake, and protection from pathogens.[34][35] PGPBs may protect plants from pathogens by competing with the pathogen for an ecological niche or a substrate, producing inhibitory allelochemicals, or inducing systemic resistance in host plants to the pathogen[36]
Effects on cognition
Depression
Microbes are also implicated in depression. The pathogenic bacteria Borrelia burgdorferi causes Lyme disease which causes depression in up to 2/3 of all cases.[37] Non-pathogenic bacteria are also implicated in depression in which bacterial populations are suppressed. One model of depression is periodic separation of infant mice from their mothers. These mice show reductions in Lactobacillus and Bifidobacterium species, functional gut abnormalities, increased corticosterone (stress hormone) levels, weight loss, and causes them to not swim as much in a forced swim test as control mice, indicating behavioural despair. Treating the mice with Lactobacillus lowered corticosterone levels and gut abnormalities.[38] Another experiment has replicated the effect that germ free mice have an exaggerated stress response and also found reduced expression of brain-derived neurotrophic factor in the cortex and hippocampus.[39] Another experiment showed that treating the maternally separated mice with a probiotic culture of Bifodobacterium infantis minimizes weight loss, causes mice to swim longer and causes an increase in the amount of the serotonin precursor tryptophan produced.[40] Increasing serotonin levels through selective serotonin reuptake inhibitors is the primary treatment of depression in humans. Human patients with depression are less able to properly digest fructose,[41] which is also associated with a reduction in tryptophan production.[42] Eliminating fructose from their diet improved their depression.[43]
Anxiety
Gut microbes are also implicated in anxiety disorders. In humans anxiety disorders are common in patients with disturbed gut flora.[44] The bacteria Campylobacter jejuni has been shown to cause anxious behaviour in mice.[45] Germ free mice show less anxious behaviour and also less NR2B mRNA expression selectively in the central amygdala which might be responsible for the anxiolytic behaviour since NR2B antagonists have an anxiolytic effect on behaviour.[46] The behavioural change might also be caused by increased brain derived neurotrophic factor (BDNF) mRNA expression possibly inducing plasticity in the dentate granular layer of the hippocampus.[47] BDNF and the hippocampus are implicated in memory. Increased gut bacterial diversity has been shown to improve both working and reference memory as well as reducing anxiety-like behaviour.[48]
Autism
Autistic populations have a unique microbiome consisting of more clostridial species.[49] Half of all autistic children with gastrointestinal dysfunction were found to have the bacteria Sutterella which was completely absent in non-autistic children with gastrointestinal dysfunction.[50] There is evidence that for some children with late-onset autism antibiotics can alleviate symptoms temporarily.[51]
Immune system
The symbiotic relationship between animal host and microbiota has a significant impact on shaping the immune system. The immune system is able to recognize the types of bacteria that are harmful to the host and combats them, while allowing the helpful bacteria to carry out their functions. After an infant is born completely sterile, their gut is quickly populated by commensal bacteria that affect the immune response, resulting in future tolerance to that bacteria. This early colonization helps to establish the symbiotic microbiome inside the host early in its life. The bacteria are also able to stimulate lymphoid tissue associated with the gut mucosa. This enables the tissue to produce antibodies for pathogens that may enter the gut. It has been found that bacteria may also play a role in the activation of TLRs (toll-like receptors) in the intestines. TLRs are a type of PRR (pattern recognition receptor) used by host cells to help repair damage and recognize dangers to the host. This could be important in immune tolerance and autoimmune diseases. Pathogens could influence this symbiotic coexistence leading to immune dysregulation and susceptibility to diseases. This could provide new direction for managing immunological and metabolic diseases.[52]
Human microbiome
The human microbiome consists of about 100 trillion microbial cells, outnumbering human cells 10 to 1.[53] It can significantly affect human physiology. For example, in healthy individuals the microbiota provide a wide range of metabolic functions that humans lack.[54] In diseased individuals altered microbiota are associated with diseases such as neonatal necrotizing enterocolitis,[55] inflammatory bowel disease[56] and vaginosis.[20] Thus studying the human microbiome is an important task that has been undertaken by initiatives such as the Human Microbiome Project[57] and MetaHIT.[58]
Studying the human microbiome
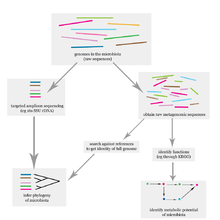
The problem of elucidating the human microbiome is essentially identifying the members of a microbial community which includes bacteria, eukaryotes, and viruses. This is done primarily using DNA-based studies, though RNA, protein and metabolite based studies are also performed.[59] DNA-based microbiome studies typically can be categorized as either targeted amplicon studies or more recently shotgun metagenomic studies. The former focuses on specific known marker genes and is primarily informative taxonomically, while the latter is an entire metagenomic approach which can also be used to study the functional potential of the community. One of the challenges that is present in human microbiome studies but not in other metagenomic studies is to avoid including the host DNA in the study.[60]
Presence of a core microbiome
Aside from simply elucidating the composition of the human microbiome, one of the major questions involving the human microbiome is whether there is a "core", that is, whether there is a subset of the community that is shared between most humans.[61][62] If there is a core, then it would be possible to associate certain community compositions with disease states, which is one of the goals of the Human Microbiome Project. It is known that the human microbiome is highly variable both within a single subject and between different individuals. For example, the gut microbiota of humans is markedly dissimilar between individuals, a phenomenon which is also observed in mice.[63] Hamady and Knight show that one can rule out the possibility that any species is shared among all humans at more than 0.9% abundance in the gut or at more than 2% abundance on hands.[62] Although there is very little species level conservation between individuals, it has been shown that this may be a result of functional redundancy as different communities tend to converge on the same functional state.[17]
On 13 June 2012, a major milestone of the Human Microbiome Project (HMP) was announced by the NIH director Francis Collins.[64] The announcement was accompanied with a series of coordinated articles published in Nature[65][66] and several journals in the Public Library of Science (PLoS) on the same day. By mapping the normal microbial make-up of healthy humans using genome sequencing techniques, the researchers of the HMP have created a reference database and the boundaries of normal microbial variation in humans. From 242 healthy U.S. volunteers, more than 5,000 samples were collected from tissues from 15 (men) to 18 (women) body sites such as mouth, nose, skin, lower intestine (stool), and vagina. All the DNA, human and microbial, were analyzed with DNA sequencing machines. The microbial genome data were extracted by identifying the bacterial specific ribosomal RNA, 16S rRNA. The researchers calculated that more than 10,000 microbial species occupy the human ecosystem and they have identified 81 – 99% of the genera.
Hologenome theory of evolution
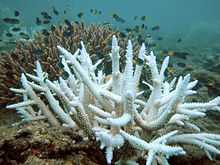
The hologenome theory proposes that the object of natural selection is not the individual organism, but the organism together with its associated microbial communities.
The hologenome theory originated in studies on coral reefs. Coral reefs are the largest structures created by living organisms, and contain abundant and highly complex microbial communities. Over the past several decades, major declines in coral populations have occurred. Climate change, water pollution and over-fishing are three stress factors that have been described as leading to disease susceptibility. Over twenty different coral diseases have been described, but of these, only a handful have had their causative agents isolated and characterized. Coral bleaching is the most serious of these diseases. In the Mediterranean Sea, the bleaching of Oculina patagonica was first described in 1994 and shortly determined to be due to infection by Vibrio shiloi. From 1994 to 2002, bacterial bleaching of O. patagonica occurred every summer in the eastern Mediterranean. Surprisingly, however, after 2003, O. patagonica in the eastern Mediterranean has been resistant to V. shiloi infection, although other diseases still cause bleaching. The surprise stems from the knowledge that corals are long lived, with lifespans on the order of decades,[67] and do not have adaptive immune systems. Their innate immune systems do not produce antibodies, and they should seemingly not be able to respond to new challenges except over evolutionary time scales. The puzzle of how corals managed to acquire resistance to a specific pathogen led Eugene Rosenberg and Ilana Zilber-Rosenberg to propose the Coral Probiotic Hypothesis. This hypothesis proposes that a dynamic relationship exists between corals and their symbiotic microbial communities. By altering its composition, this "holobiont" can adapt to changing environmental conditions far more rapidly than by genetic mutation and selection alone. Extrapolating this hypothesis of adaptation and evolution to other organisms, including higher plants and animals, led to the proposal of the Hologenome Theory of Evolution.[68]
The hologenome theory is still being debated.[69] A major criticism has been the claim that V. shiloi was misidentified as the causative agent of coral bleaching, and that its presence in bleached O. patagonica was simply that of opportunistic colonization.[70] If this is true, the basic observation leading to the theory would be invalid. Nevertheless, the theory has gained significant popularity as a way of explaining rapid changes in adaptation that cannot otherwise be explained by traditional mechanisms of natural selection. For those who accept the hologenome theory, the holobiont has become the principal unit of natural selection. On the other hand, it has been stated that the holobiont is the result of other step of integration that it is also observed at the cell (symbiogenesis, endosymbiosis) and genomic levels.[14]
Research methods
Targeted amplicon sequencing
Targeted amplicon sequencing relies on having some expectations about the composition of the community that is being studied. In target amplicon sequencing a phylogenetically informative marker is targeted for sequencing. Such a marker should be present in ideally all the expected organisms. It should also evolve in such a way that it is conserved enough that primers can target genes from a wide range of organisms while evolving quickly enough to allow for finer resolution at the taxonomic level. A common marker for human microbiome studies is the gene for bacterial 16S rRNA (i.e. "16S rDNA", the sequence of DNA which encodes the ribosomal RNA molecule).[59] Since ribosomes are present in all living organisms, using 16S rDNA allows for DNA to be amplified from many more organisms than if another marker were used. The 16S rDNA gene contains both slowly evolving regions and fast evolving regions; the former can be used to design broad primers while the latter allow for finer taxonomic distinction. However, species-level resolution is not typically possible using the 16S rDNA. Primer selection is an important step, as anything that cannot be targeted by the primer will not be amplified and thus will not be detected. Different sets of primers have been shown to amplify different taxonomic groups due to sequence variation.
Targeted studies of eukaryotic and viral communities are limited[71] and subject to the challenge of excluding host DNA from amplification and the reduced eukaryotic and viral biomass in the human microbiome.[60]
After the amplicons are sequenced, molecular phylogenetic methods are used to infer the composition of the microbial community. This is done by clustering the amplicons into operational taxonomic units (OTUs) and inferring phylogenetic relationships between the sequences. Due to the complexity of the data, distance measures such as UniFrac distances are usually defined between microbiome samples, and downstream multivariate methods are carried out on the distance matrices. An important point is that the scale of data is extensive, and further approaches must be taken to identify patterns from the available information. Tools used to analyze the data include VAMPS,[72] QIIME[73] and mothur.[74]
Metagenomic sequencing
Metagenomics is also used extensively for studying microbial communities.[17][58][75] In metagenomic sequencing, DNA is recovered directly from environmental samples in an untargeted manner with the goal of obtaining an unbiased sample from all genes of all members of the community. Recent studies use shotgun Sanger sequencing or pyrosequencing to recover the sequences of the reads.[76] The reads can then be assembled into contigs. To determine the phylogenetic identity of a sequence, it is compared to available full genome sequences using methods such as BLAST. One drawback of this approach is that many members of microbial communities do not have a representative sequenced genome.[59]
Despite the fact that metagenomics is limited by the availability of reference sequences, one significant advantage of metagenomics over targeted amplicon sequencing is that metagenomics data can elucidate the functional potential of the community DNA.[77][78] Targeted gene surveys cannot do this as they only reveal the phylogenetic relationship between the same gene from different organisms. Functional analysis is done by comparing the recovered sequences to databases of metagenomic annotations such as KEGG. The metabolic pathways that these genes are involved in can then be predicted with tools such as MG-RAST,[79] CAMERA[80] and IMG/M.[81]
RNA and protein-based approaches
Metatranscriptomics studies have been performed to study the gene expression of microbial communities through methods such as the pyrosequencing of extracted RNA.[82] Structure based studies have also identified non-coding RNAs (ncRNAs) such as ribozymes from microbiota.[83] Metaproteomics is a new approach that studies the proteins expressed by microbiota, giving insight into its functional potential.[84]
Projects
The Human Microbiome Project (HMP) is a United States National Institutes of Health initiative with the goal of identifying and characterizing the microorganisms which are found in association with both healthy and diseased humans (their microbial flora). Launched in 2008, it is a five-year project, best characterized as a feasibility study, and has a total budget of $115 million. The ultimate goal of this and similar NIH-sponsored microbiome projects is to test how changes in the human microbiome are associated with human health or disease.
The Earth Microbiome Project (EMP) is an initiative to collect natural samples and analyze the microbial community around the globe. Microbes are highly abundant, diverse and have an important role in the ecological system. Yet as of 2010, it was estimated that the total global environmental DNA sequencing effort had produced less than 1 percent of the total DNA found in a liter of seawater or a gram of soil,[85] and the specific interactions between microbes are largely unknown. The EMP aims to process as many as 200,000 samples in different biomes, generating a complete database of microbes on earth to characterize environments and ecosystems by microbial composition and interaction. Using these data, new ecological and evolutionary theories can be proposed and tested.[86]
The Brazilian Microbiome Project (BMP) aims to assemble a Brazilian Microbiome Consortium/Database. At present, many metagenomic projects underway in Brazil are widely known. Our goal is to co-ordinate and standardize these, together with future projects. This is the first attempt to collect and collate information about Brazilian microbial genetic and functional diversity in a systematic and holistic manner. New sequence data have been generated from samples collected in all Brazilian regions, however the success of the BMP depends on a massive collaborative effort of both the Brazilian and international scientific communities. Therefore, we invite all colleagues to participate in this project. There is no prioritization of specific taxonomic groups, studies could include any ecosystem, and all proposals and any help will be very welcome.
A philosophical approach to the impacts of microbiomes in human behavior plays a part in Mookie Tenembaum's system of Disillusionism.
Conclusion
Many more case studies exist than the few presented in this article, which illustrate the diverse interactions that have been shown to exist between macro organisms and their microbial inhabitants. Elucidation of these interactions has required new technologies and an interdisciplinary approach. Genomics and ecology, once separate disciplines, are showing rapid convergence, and may together allow us to understand the molecular basis underlying the adaptations and interactions of the communities of life.[15]
See also
- Anagenesis
- Biome
- Gut flora
- Human microbiome
- Human microbiome project
- Metagenomics
- Multigenomic organism
- Skin flora
- Microbiota of the lower reproductive tract of women
References
- ↑ Lederberg, J; McCray, AT (2001). "'Ome Sweet 'Omics—a genealogical treasury of words". Scientist 15: 8.
- ↑ "The NIH Human Microbiome Project". Genome Res 19 (12): 2317–2323. 2009. doi:10.1101/gr.096651.109.
- ↑ Backhed, F; Ley, R.E.; Sonnenburg, J.L.; Peterson, D.A.; Gordon, J.I. (2005). "Host-Bacterial Mutualism in the Human Intestine". Science 307: 1915–1920. doi:10.1126/science.1104816.
- ↑ Turnbaugh, P.J.; Ley, R.E.; Hamady, M.; Fraser-Liggett, C.M.; Knight, R.; Gordon, J.I. (2007). "The Human Microbiome Project". Nature 449: 804–810. doi:10.1038/nature06244. PMID 17943116.
- ↑ Ley, R.E.; Peterson, D.A.; Gordon, J.I. (2006). "Ecological and Evolutionary Forces Shaping Microbial Diversity in the Human Intestine". Cell 124: 837–848. doi:10.1016/j.cell.2006.02.017.
- ↑ Zimmer, Carl (13 July 2010). "How Microbes Defend and Define Us". New York Times. Retrieved 17 July 2010.
- ↑ Because bacteria are 10-100 times smaller than human cells, the entire microbiome weighs about 200 grams (7.1 oz). See also Coyle, MD, Walter J. "The Human Microbiome: The Undiscovered Country". p. 16. Retrieved 2 March 2012
- ↑ Willyard C (24 November 2011). "Microbiome: Gut reaction". Nature 479 (7374): S5–S7. doi:10.1038/479S5a.
- ↑ Turnbaugh, Peter J., et al. "A core gut microbiome in obese and lean twins." nature 457.7228 (2009): 480-484.
- ↑ Ridaura, Vanessa K., et al. "Gut microbiota from twins discordant for obesity modulate metabolism in mice." Science 341.6150 (2013): 1241214.
- ↑ Turnbaugh, Peter J., et al. "An obesity-associated gut microbiome with increased capacity for energy harvest." Nature 444.7122 (2006): 1027-131.
- ↑ Bravo, Javier A., et al. "Ingestion of Lactobacillus strain regulates emotional behavior and central GABA receptor expression in a mouse via the vagus nerve." Proceedings of the National Academy of Sciences 108.38 (2011): 16050-16055.
- ↑ Madigan, Michael T. (2012). Brock biology of microorganisms (13th ed.). San Francisco: Benjamin Cummings. ISBN 9780321649638.
- ↑ 14.0 14.1 Salvucci, E. (2014). "Microbiome, holobiont and the net of life". Critical Reviews in Microbiology: 1. doi:10.3109/1040841X.2014.962478.
- ↑ 15.0 15.1 Bosch, T. C. G.; McFall-Ngai, M. J. (2011). "Metaorganisms as the new frontier". Zoology 114 (4): 185–190. doi:10.1016/j.zool.2011.04.001. PMID 21737250.
- ↑ Poreau B., Biologie et complexité : histoire et modèles du commensalisme. PhD Dissertation, University of Lyon, France, 2014.
- ↑ 17.0 17.1 17.2 Turnbaugh, P. J.; Hamady, M.; Yatsunenko, T.; Cantarel, B. L.; Duncan, A.; Ley, R. E.; Sogin, M. L.; Jones, W. J.; Roe, B. A.; Affourtit, J. P.; Egholm, M.; Henrissat, B.; Heath, A. C.; Knight, R.; Gordon, J. I. (2008). "A core gut microbiome in obese and lean twins". Nature 457 (7228): 480–484. doi:10.1038/nature07540. PMC 2677729. PMID 19043404.
- ↑ Giongo A, Gano KA, Crabb DB, Mukherjee N, Novelo LL, Casella G, Drew JC, Ilonen J, Knip M, Hyöty H, Veijola R, Simell T, Simell O, Neu J, Wasserfall CH, Schatz D, Atkinson MA, Triplett EW (2011). "Toward defining the autoimmune microbiome for type 1 diabetes". The ISME Journal 5 (1): 82–91. doi:10.1038/ismej.2010.92. PMC 3105672. PMID 20613793.
- ↑ Grice EA, Kong HH, Conlan S, Deming CB, Davis J, Young AC, NISC Comparative Sequencing Program, Bouffard GG, Blakesley RW, Murray PR, Green ED, Turner ML, Segre JA; Kong; Conlan; Deming; Davis; Young; Bouffard; Blakesley; Murray; Green; Turner; Segre (2009). "Topographical and Temporal Diversity of the Human Skin Microbiome". Science 324 (5931): 1190–2. Bibcode:2009Sci...324.1190G. doi:10.1126/science.1171700. PMC 2805064. PMID 19478181.
- ↑ 20.0 20.1 Ravel, J.; Gajer, P.; Abdo, Z.; Schneider, G. M.; Koenig, S. S. K.; McCulle, S. L.; Karlebach, S.; Gorle, R.; Russell, J.; Tacket, C. O.; Brotman, R. M.; Davis, C. C.; Ault, K.; Peralta, L.; Forney, L. J. (2010). "Colloquium Paper: Vaginal microbiome of reproductive-age women". Proceedings of the National Academy of Sciences 108 (Supplement_1): 4680–4687. doi:10.1073/pnas.1002611107. PMC 3063603. PMID 20534435.
- ↑ Zimmer, Carl (20 April 2011). "Bacteria Divide People Into 3 Types, Scientists Say". The New York Times. Retrieved 21 April 2011.
a group of scientists now report just three distinct ecosystems in the guts of people they have studied.
- ↑ Arumugam, Manimozhiyan et al. (March 2010). "Enterotypes of the human gut microbiome". Nature 473 (7346): 174–80. Bibcode:2011Natur.473..174.. doi:10.1038/nature09944. PMC 3728647. PMID 21508958.
- ↑ Ivanov II, de Llanos Frutos R, Manel N, Yoshinaga K, Rifkin DB, Sartor RB, Finlay BB, Littman DR (2008). "Specific microbiota direct the differentiation of Th17 cells in the mucosa of the small intestine". Cell Host Microbe 4 (4): 337–349. doi:10.1016/j.chom.2008.09.009. PMC 2597589. PMID 18854238.
- ↑ Stuart SN, Chanson JS, Cox NA, Young BE, Rodrigues ASL, Fischman DL, Waller RW; Chanson; Cox; Young; Rodrigues; Fischman; Waller (2004). "Status and Trends of Amphibian Declines and Extinctions Worldwide" (PDF). Science 306 (5702): 1783–6. Bibcode:2004Sci...306.1783S. doi:10.1126/science.1103538. PMID 15486254.
- ↑ Woodhams DC, Rollins-Smith LA, Alford RA, Simon MA, Harris RN (2007). "Innate immune defenses of amphibian skin: antimicrobial peptides and more". Animal Conservation 10 (4): 425–8. doi:10.1111/j.1469-1795.2007.00150.x.
- ↑ Brulc JM, Antonopoulos DA, Miller MEB, Wilson MK, Yannarell AC, Dinsdale EA, Edwards RE, Frank ED, Emerson JB, Wacklin P, Coutinho PM, Henrissat B, Nelson KE, White BA; Antonopoulos; Berg Miller; Wilson; Yannarell; Dinsdale; Edwards; Frank; Emerson; Wacklin; Coutinho; Henrissat; Nelson; White (2009). "Gene-centric metagenomics of the fiber-adherent bovine rumen microbiome reveals forage specific glycoside hydrolases". Proc. Natl. Acad. Sci. USA 106 (6): 1948–53. Bibcode:2009PNAS..106.1948B. doi:10.1073/pnas.0806191105. PMC 2633212. PMID 19181843.
- ↑ Suen G, Scott JJ, Aylward FO, Adams SM, Tringe SG, Pinto-Tomás AA, Foster CE, Pauly M, Weimer PJ, Barry KW, Goodwin LA, Bouffard P, Li L, Osterberger J, Harkins TT, Slater SC, Donohue TJ, Currie CR (2010). Sonnenburg, Justin, ed. "An Insect Herbivore Microbiome with High Plant Biomass-Degrading Capacity". PLoS Genet 6 (9): e1001129. doi:10.1371/journal.pgen.1001129. PMC 2944797. PMID 20885794.
- ↑ Russell SL, , Gold MJ et al. (May 2012). "Early life antibiotic-driven changes in microbiota enhance susceptibility to allergic asthma.". EMBO Rep. 13 (5): 440–7. doi:10.1038/embor.2012.32. PMID 22422004.
- ↑ Russell SL, Gold MJ et al. (Aug 2014). "Perinatal antibiotic-induced shifts in gut microbiota have differential effects on inflammatory lung diseases.". J Allergy Clin Immunol. 135: 100–9. doi:10.1016/j.jaci.2014.06.027. PMID 25145536.
- ↑ Faith JJ, Ahern PP, Ridaura VK et al. (Jan 2014). "Identifying gut microbe-host phenotype relationships using combinatorial communities in gnotobiotic mice.". Sci Transl Med. 6 (220): 220. doi:10.1126/scitranslmed.3008051. PMC 3973144. PMID 24452263.
- ↑ Barfod, KK; Roggenbuck, M; Hansen, LH; Schjørring, S; Larsen, ST; Sørensen, SJ; Krogfelt, KA (2013). "The murine lung microbiome in relation to the intestinal and vaginal bacterial communities". BMC Microbiol 13: 303. doi:10.1186/1471-2180-13-303.
- ↑ Remy W, Taylor TN, Hass H, Kerp H; Taylor; Hass; Kerp (1994). "Four hundred-million-year-old vesicular arbuscular mycorrhizae" (PDF). Proc. Natl. Acad. Sci. USA 91 (25): 11841–3. Bibcode:1994PNAS...9111841R. doi:10.1073/pnas.91.25.11841. PMC 45331. PMID 11607500.
- ↑ Chibucos MC, Tyler BM (2009). "Common themes in nutrient acquisition by plant symbiotic microbes, described by the Gene Ontology". BMC Microbiology. 9(Suppl 1): S6. doi:10.1186/1471-2180-9-S1-S6.
- ↑ Kloepper, J. W (1993). "Plant growth-promoting rhizobacteria as biological control agents". In Metting, F. B., Jr. Soil microbial ecology: applications in agricultural and environmental management. New York: Marcel Dekker Inc. pp. 255–274. ISBN 0-8247-8737-4.
- ↑ Bloemberg, G. V.; Lugtenberg, B. J. J. (2001). "Molecular basis of plant growth promotion and biocontrol by rhizobacteria". Current Opinion in Plant Biology 4 (4): 343–350. doi:10.1016/S1369-5266(00)00183-7. PMID 11418345.
- ↑ Compant S, Duffy B, Nowak J, Clément C, Barka EA (2005). "Use of Plant Growth-Promoting Bacteria for Biocontrol of Plant Diseases: Principles, Mechanisms of Action, and Future Prospects". Appl Environ Microbiol. 71 (9): 4951–9. doi:10.1128/AEM.71.9.4951-4959.2005. PMC 1214602. PMID 16151072.
- ↑ Fallon, B. A.; Nields, J. A. (1994). "Lyme disease: A neuropsychiatric illness". The American journal of psychiatry 151 (11): 1571–1583. PMID 7943444.
- ↑ Gareau, M. G.; Jury, J.; MacQueen, G.; Sherman, P. M.; Perdue, M. H. (2007). "Probiotic treatment of rat pups normalises corticosterone release and ameliorates colonic dysfunction induced by maternal separation". Gut 56 (11): 1522–1528. doi:10.1136/gut.2006.117176. PMC 2095679. PMID 17339238.
- ↑ Sudo, N.; Chida, Y.; Aiba, Y.; Sonoda, J.; Oyama, N.; Yu, X. N.; Kubo, C.; Koga, Y. (2004). "Postnatal microbial colonization programs the hypothalamic-pituitary-adrenal system for stress response in mice". The Journal of Physiology 558 (Pt 1): 263–275. doi:10.1113/jphysiol.2004.063388. PMC 1664925. PMID 15133062.
- ↑ Desbonnet, L.; Garrett, L.; Clarke, G.; Kiely, B.; Cryan, J. F.; Dinan, T. G. (2010). "Effects of the probiotic Bifidobacterium infantis in the maternal separation model of depression". Neuroscience 170 (4): 1179–1188. doi:10.1016/j.neuroscience.2010.08.005. PMID 20696216.
- ↑ Ledochowski, M.; Widner, B.; Sperner-Unterweger, B.; Propst, T.; Vogel, W.; Fuchs, D. (2000). "Carbohydrate malabsorption syndromes and early signs of mental depression in females". Digestive diseases and sciences 45 (7): 1255–1259. doi:10.1023/A:1005527230346. PMID 10961700.
- ↑ Ledochowski, M.; Widner, B.; Murr, C.; Sperner-Unterweger, B.; Fuchs, D. (2001). "Fructose malabsorption is associated with decreased plasma tryptophan". Scandinavian journal of gastroenterology 36 (4): 367–371. doi:10.1080/003655201300051135. PMID 11336160.
- ↑ Ledochowski, M.; Widner, B.; Bair, H.; Probst, T.; Fuchs, D. (2000). "Fructose- and sorbitol-reduced diet improves mood and gastrointestinal disturbances in fructose malabsorbers". Scandinavian journal of gastroenterology 35 (10): 1048–1052. doi:10.1080/003655200451162. PMID 11099057.
- ↑ Mikocka-Walus, A. A.; Turnbull, D. A.; Moulding, N. T.; Wilson, I. G.; Andrews, J. M.; Holtmann, G. J. (2007). "Controversies surrounding the comorbidity of depression and anxiety in inflammatory bowel disease patients". Inflammatory Bowel Diseases 13 (2): 225–234. doi:10.1002/ibd.20062. PMID 17206706.
- ↑ Goehler, L. E.; Park, S. M.; Opitz, N.; Lyte, M.; Gaykema, R. P. A. (2008). "Campylobacter jejuni infection increases anxiety-like behavior in the holeboard: Possible anatomical substrates for viscerosensory modulation of exploratory behavior". Brain, Behavior, and Immunity 22 (3): 354–366. doi:10.1016/j.bbi.2007.08.009. PMC 2259293. PMID 17920243.
- ↑ Rodrigues, S. M.; Schafe, G. E.; Ledoux, J. E. (2001). "Intra-amygdala blockade of the NR2B subunit of the NMDA receptor disrupts the acquisition but not the expression of fear conditioning". The Journal of neuroscience : the official journal of the Society for Neuroscience 21 (17): 6889–6896. PMID 11517276.
- ↑ Neufeld, K. M.; Kang, N.; Bienenstock, J.; Foster, J. A. (2011). "Reduced anxiety-like behavior and central neurochemical change in germ-free mice". Neurogastroenterology & Motility 23 (3): 255–264, e119. doi:10.1111/j.1365-2982.2010.01620.x. PMID 21054680.
- ↑ Li, W.; Dowd, S. E.; Scurlock, B.; Acosta-Martinez, V.; Lyte, M. (2009). "Memory and learning behavior in mice is temporally associated with diet-induced alterations in gut bacteria". Physiology & Behavior 96 (4–5): 557–567. doi:10.1016/j.physbeh.2008.12.004. PMID 19135464.
- ↑ Finegold, S. M.; Molitoris, D.; Song, Y.; Liu, C.; Vaisanen, M. L.; Bolte, E.; McTeague, M.; Sandler, R.; Wexler, H.; Marlowe, E. M.; Collins, M. D.; Lawson, P. A.; Summanen, P.; Baysallar, M.; Tomzynski, T. J.; Read, E.; Johnson, E.; Rolfe, R.; Nasir, P.; Shah, H.; Haake, D. A.; Manning, P.; Kaul, A. (2002). "Gastrointestinal Microflora Studies in Late‐Onset Autism". Clinical Infectious Diseases 35 (Suppl 1): S6–S16. doi:10.1086/341914. PMID 12173102.
- ↑ Williams, B. L.; Hornig, M.; Parekh, T.; Lipkin, W. I. (2012). "Application of Novel PCR-Based Methods for Detection, Quantitation, and Phylogenetic Characterization of Sutterella Species in Intestinal Biopsy Samples from Children with Autism and Gastrointestinal Disturbances". MBio 3 (1): e00261–e00211. doi:10.1128/mBio.00261-11. PMC 3252763. PMID 22233678.
- ↑ Sandler, R. H.; Finegold, S. M.; Bolte, E. R.; Buchanan, C. P.; Maxwell, A. P.; Väisänen, M. L.; Nelson, M. N.; Wexler, H. M. (2000). "Short-term benefit from oral vancomycin treatment of regressive-onset autism". Journal of child neurology 15 (7): 429–435. doi:10.1177/088307380001500701. PMID 10921511.
- ↑ Nikoopour, E; Singh, B (2014). "Reciprocity in microbiome and immune system interactions and its implications in disease and health". Inflamm Allergy Drug Targets 13 (2): 94–104. doi:10.2174/1871528113666140330201056. PMID 24678760.
- ↑ Savage, D. C. (1977). "Microbial Ecology of the Gastrointestinal Tract". Annual Review of Microbiology 31: 107–133. doi:10.1146/annurev.mi.31.100177.000543. PMID 334036.
- ↑ Gill, S. R.; Pop, M.; Deboy, R. T.; Eckburg, P. B.; Turnbaugh, P. J.; Samuel, B. S.; Gordon, J. I.; Relman, D. A.; Fraser-Liggett, C. M.; Nelson, K. E. (2006). "Metagenomic Analysis of the Human Distal Gut Microbiome". Science 312 (5778): 1355–1359. doi:10.1126/science.1124234. PMC 3027896. PMID 16741115.
- ↑ Mitchum, Rob (2012-03-05). "Bacterial frontier could yield future cures: UChicago scientists find untapped potential in the "microbiome" of bacteria inside the human body." (WEB FEATURE). The University of Chicago. Retrieved 2013-02-26.
- ↑ Aas, J.; Gessert, C. E.; Bakken, J. S. (2003). "RecurrentClostridium difficileColitis: Case Series Involving 18 Patients Treated with Donor Stool Administered via a Nasogastric Tube". Clinical Infectious Diseases 36 (5): 580–585. doi:10.1086/367657. PMID 12594638.
- ↑ Peterson, J.; Peterson, S.; Garges, M.; Giovanni, P.; McInnes, L.; Wang, J. A.; Schloss, V.; Bonazzi, J. E.; McEwen, K. A.; Wetterstrand, C.; Deal, C. C.; Baker, V.; Di Francesco, T. K.; Howcroft, R. W.; Karp, R. D.; Lunsford, C. R.; Wellington, T.; Belachew, M.; Wright, C.; Giblin, H.; David, M.; Mills, R.; Salomon, C.; Mullins, B.; Akolkar, L.; Begg, C.; Davis, L.; Grandison, M.; Humble, J.; Khalsa, A. R. (2009). "The NIH Human Microbiome Project". Genome Research 19 (12): 2317–2323. doi:10.1101/gr.096651.109. PMC 2792171. PMID 19819907.
- ↑ 58.0 58.1 Qin, J.; Li, R.; Raes, J.; Arumugam, M.; Burgdorf, K. S.; Manichanh, C.; Nielsen, T.; Pons, N.; Levenez, F.; Yamada, T.; Mende, D. R.; Li, J.; Xu, J.; Li, S.; Li, D.; Cao, J.; Wang, B.; Liang, H.; Zheng, H.; Xie, Y.; Tap, J.; Lepage, P.; Bertalan, M.; Batto, J. M.; Hansen, T.; Le Paslier, D.; Linneberg, A.; Nielsen, H. B. R.; Pelletier, E.; Renault, P. (2010). "A human gut microbial gene catalogue established by metagenomic sequencing". Nature 464 (7285): 59–65. doi:10.1038/nature08821. PMC 3779803. PMID 20203603.
- ↑ 59.0 59.1 59.2 Kuczynski, J.; Lauber, C. L.; Walters, W. A.; Parfrey, L. W.; Clemente, J. C.; Gevers, D.; Knight, R. (2011). "Experimental and analytical tools for studying the human microbiome". Nature Reviews Genetics 13 (1): 47–58. doi:10.1038/nrg3129. PMID 22179717.
- ↑ 60.0 60.1 Vestheim, H.; Jarman, S. N. (2008). "Blocking primers to enhance PCR amplification of rare sequences in mixed samples – a case study on prey DNA in Antarctic krill stomachs". Frontiers in Zoology 5: 12. doi:10.1186/1742-9994-5-12. PMC 2517594. PMID 18638418.
- ↑ Tap, Julien; Mondot, Stanislas.; Levenez, Florence; Pelletier, Eric; Caron, Christophe; Furet, Jean-Pierre; Ugarte, Edgardo; Munoz-Tamayo, Rafael et al. (2009). "Towards the human intestinal microbiota phylogenetic core". Environmental Microbiology 11 (102): 2574–2584. doi:10.1111/j.1462-2920.2009.01982.x. PMID 19601958.
- ↑ 62.0 62.1 Hamady, M.; Knight, R. (2009). "Microbial community profiling for human microbiome projects: Tools, techniques, and challenges". Genome Research 19 (7): 1141–1152. doi:10.1101/gr.085464.108. PMID 19383763.
- ↑ Ley, R. E.; Bäckhed, F.; Turnbaugh, P.; Lozupone, C. A.; Knight, R. D.; Gordon, J. I. (2005). "Obesity alters gut microbial ecology". Proceedings of the National Academy of Sciences 102 (31): 11070–11075. doi:10.1073/pnas.0504978102. PMC 1176910. PMID 16033867.
- ↑ "NIH Human Microbiome Project defines normal bacterial makeup of the body". NIH News. 13 June 2012.
- ↑ The Human Microbiome Project Consortium, Barbara A.; Nelson, Karen E.; Pop, Mihai; Creasy, Heather H.; Giglio, Michelle G.; Huttenhower, Curtis; Gevers, Dirk; Petrosino, Joseph F. et al. (2012). "A framework for human microbiome research". Nature 486 (7402): 215–221. Bibcode:2012Natur.486..215T. doi:10.1038/nature11209. PMC 3377744. PMID 22699610.
- ↑ The Human Microbiome Project Consortium, Curtis; Gevers, Dirk; Knight, Rob; Abubucker, Sahar; Badger, Jonathan H.; Chinwalla, Asif T.; Creasy, Heather H.; Earl, Ashlee M. et al. (2012). "Structure, function and diversity of the healthy human microbiome". Nature 486 (7402): 207–214. Bibcode:2012Natur.486..207T. doi:10.1038/nature11234. PMC 3564958. PMID 22699609.
- ↑ Baird AH, Bhagooli R, Ralph PJ, Takahashi S (2009). "Coral bleaching: the role of the host" (PDF). Trends in Ecology and Evolution 24 (1): 16–20. doi:10.1016/j.tree.2008.09.005. PMID 19022522.
- ↑ Rosenberg E, Koren O, Reshef L, Efrony R, Zilber-Rosenberg I (2007). "The role of microorganisms in coral health, disease and evolution" (PDF). Nature Reviews Microbiology 5 (5): 355–362. doi:10.1038/nrmicro1635. PMID 17384666.
- ↑ Leggat W, Ainsworth T, Bythell J, Dove S, Gates R, Hoegh-Guldberg O, Iglesias-Prieto R, Yellowlees D (2007). "The hologenome theory disregards the coral holobiont". Nature Reviews Microbiology 5 (10): Online Correspondence. doi:10.1038/nrmicro1635-c1.
- ↑ Ainsworth TD, Fine M, Roff G, Hoegh-Guldberg O (2008). "Bacteria are not the primary cause of bleaching in the Mediterranean coral Oculina patagonica". The ISME Journal 2 (1): 67–73. doi:10.1038/ismej.2007.88. PMID 18059488.
- ↑ Marchesi, J. R. (2010). "Prokaryotic and Eukaryotic Diversity of the Human Gut". Advances in Applied Microbiology Volume 72. Advances in Applied Microbiology 72. pp. 43–62. doi:10.1016/S0065-2164(10)72002-5. ISBN 9780123809896. PMID 20602987.
- ↑ "VAMPS: The Visualization and Analysis of Microbial Population Structures". Bay Paul Center, MBL, Woods Hole. Retrieved 11 March 2012.
- ↑ Caporaso, J. G.; Kuczynski, J.; Stombaugh, J.; Bittinger, K.; Bushman, F. D.; Costello, E. K.; Fierer, N.; Peña, A. G.; Goodrich, J. K.; Gordon, J. I.; Huttley, G. A.; Kelley, S. T.; Knights, D.; Koenig, J. E.; Ley, R. E.; Lozupone, C. A.; McDonald, D.; Muegge, B. D.; Pirrung, M.; Reeder, J.; Sevinsky, J. R.; Turnbaugh, P. J.; Walters, W. A.; Widmann, J.; Yatsunenko, T.; Zaneveld, J.; Knight, R. (2010). "QIIME allows analysis of high-throughput community sequencing data". Nature Methods 7 (5): 335–336. doi:10.1038/nmeth.f.303. PMC 3156573. PMID 20383131.
- ↑ Schloss, P. D.; Westcott, S. L.; Ryabin, T.; Hall, J. R.; Hartmann, M.; Hollister, E. B.; Lesniewski, R. A.; Oakley, B. B.; Parks, D. H.; Robinson, C. J.; Sahl, J. W.; Stres, B.; Thallinger, G. G.; Van Horn, D. J.; Weber, C. F. (2009). "Introducing mothur: Open-Source, Platform-Independent, Community-Supported Software for Describing and Comparing Microbial Communities". Applied and Environmental Microbiology 75 (23): 7537–7541. doi:10.1128/AEM.01541-09. PMC 2786419. PMID 19801464.
- ↑ Tringe, S. G.; Von Mering, C.; Kobayashi, A.; Salamov, A. A.; Chen, K.; Chang, H. W.; Podar, M.; Short, J. M.; Mathur, E. J.; Detter, J. C.; Bork, P.; Hugenholtz, P.; Rubin, E. M. (2005). "Comparative Metagenomics of Microbial Communities". Science 308 (5721): 554–557. doi:10.1126/science.1107851. PMID 15845853.
- ↑ Wooley, J. C.; Godzik, A.; Friedberg, I. (2010). Bourne, Philip E., ed. "A Primer on Metagenomics". PLoS Computational Biology 6 (2): e1000667. doi:10.1371/journal.pcbi.1000667. PMC 2829047. PMID 20195499.
- ↑ Muller, J.; Szklarczyk, D.; Julien, P.; Letunic, I.; Roth, A.; Kuhn, M.; Powell, S.; Von Mering, C.; Doerks, T.; Jensen, L. J.; Bork, P. (2009). "EggNOG v2.0: Extending the evolutionary genealogy of genes with enhanced non-supervised orthologous groups, species and functional annotations". Nucleic Acids Research 38 (Database issue): D190–D195. doi:10.1093/nar/gkp951. PMC 2808932. PMID 19900971.
- ↑ Kanehisa, M.; Goto, S.; Furumichi, M.; Tanabe, M.; Hirakawa, M. (2009). "KEGG for representation and analysis of molecular networks involving diseases and drugs". Nucleic Acids Research 38 (Database issue): D355–D360. doi:10.1093/nar/gkp896. PMC 2808910. PMID 19880382.
- ↑ Meyer, F.; Paarmann, D.; d'Souza, M.; Olson, R.; Glass, E. M.; Kubal, M.; Paczian, T.; Rodriguez, A.; Stevens, R.; Wilke, A.; Wilkening, J.; Edwards, R. A. (2008). "The metagenomics RAST server – a public resource for the automatic phylogenetic and functional analysis of metagenomes". BMC Bioinformatics 9: 386. doi:10.1186/1471-2105-9-386. PMC 2563014. PMID 18803844.
- ↑ Sun, S.; Chen, J.; Li, W.; Altintas, I.; Lin, A.; Peltier, S.; Stocks, K.; Allen, E. E.; Ellisman, M.; Grethe, J.; Wooley, J. (2010). "Community cyberinfrastructure for Advanced Microbial Ecology Research and Analysis: The CAMERA resource". Nucleic Acids Research 39 (Database issue): D546–D551. doi:10.1093/nar/gkq1102. PMC 3013694. PMID 21045053.
- ↑ Markowitz, V. M.; Ivanova, N. N.; Szeto, E.; Palaniappan, K.; Chu, K.; Dalevi, D.; Chen, I. M. A.; Grechkin, Y.; Dubchak, I.; Anderson, I.; Lykidis, A.; Mavromatis, K.; Hugenholtz, P.; Kyrpides, N. C. (2007). "IMG/M: A data management and analysis system for metagenomes". Nucleic Acids Research 36 (Database issue): D534–D538. doi:10.1093/nar/gkm869. PMC 2238950. PMID 17932063.
- ↑ Shi, Y.; Tyson, G. W.; Delong, E. F. (2009). "Metatranscriptomics reveals unique microbial small RNAs in the ocean's water column". Nature 459 (7244): 266–269. doi:10.1038/nature08055. PMID 19444216.
- ↑ Jimenez, R. M.; Delwart, E.; Luptak, A. (2011). "Structure-based Search Reveals Hammerhead Ribozymes in the Human Microbiome". Journal of Biological Chemistry 286 (10): 7737–7743. doi:10.1074/jbc.C110.209288. PMC 3048661. PMID 21257745.
- ↑ Maron, P. A.; Ranjard, L.; Mougel, C.; Lemanceau, P. (2007). "Metaproteomics: A New Approach for Studying Functional Microbial Ecology". Microbial Ecology 53 (3): 486–493. doi:10.1007/s00248-006-9196-8. PMID 17431707.
- ↑ Gilbert, J. A.; Meyer, F.; Antonopoulos, D.; Balaji, P.; Brown, C. T.; Brown, C. T.; Desai, N.; Eisen, J. A.; Evers, D.; Field, D.; Feng, W.; Huson, D.; Jansson, J.; Knight, R.; Knight, J.; Kolker, E.; Konstantindis, K.; Kostka, J.; Kyrpides, N.; MacKelprang, R.; McHardy, A.; Quince, C.; Raes, J.; Sczyrba, A.; Shade, A.; Stevens, R. (2010). "Meeting Report: The Terabase Metagenomics Workshop and the Vision of an Earth Microbiome Project". Standards in Genomic Sciences 3 (3): 243–248. doi:10.4056/sigs.1433550. PMC 3035311. PMID 21304727.
- ↑ Gilbert, J. A.; O'Dor, R.; King, N.; Vogel, T. M. (2011). "The importance of metagenomic surveys to microbial ecology: Or why Darwin would have been a metagenomic scientist". Microbial Informatics and Experimentation 1: 5. doi:10.1186/2042-5783-1-5.
External links
![]() |
Look up microbiota in Wiktionary, the free dictionary. |
- "The microbiome in autoinflammatory diseases"
- "Exploring The Human Microbiome: Diet, Microbes and Western Diseases"