MXenes
In materials science, MXenes are a class of two-dimensional inorganic compounds (i.e. graphene, which is the most famous member of two-dimensional materials family). These materials consist of few atoms thick layers of transition metal carbide or carbonitrides. First described in 2011, MXenes combine metallic conductivity of transition metal carbides and hydrophilic nature because of their hydroxyl or oxygen terminated surfaces.[1][2]
Structure
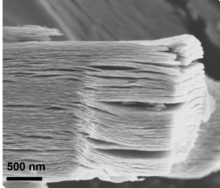
As-synthesized MXenes prepared via HF etching have an accordion-like morphology, which can be referred to as a multi-layer MXene (ML-MXene), or a few-layer MXene (FL-MXene) when there are fewer than five layers. Because the surfaces of MXenes can be terminated by functional groups, the naming convention Mn+1XnTx can be used, where T is a functional group (e.g. O, F, OH).[2]
MXenes adopt three structures, as inherited from the parent MAX phases: M2C, M3C2, and M4C3. They are produced by selectively etching out the A element from a MAX phase, which has the general formula Mn+1AXn, where M is an early transition metal, A is an element from group IIIA or IVA of the periodic table, X is C and/or N, and n = 1, 2, or 3. MAX phases have a layered hexagonal structure with P63/mmc symmetry, where M layers are nearly closed packed and X atoms fill octahedral sites.[2] Therefore, Mn+1Xn layers are interleaved with the A element, which is metallically bonded to the M element.[3]
Preparation
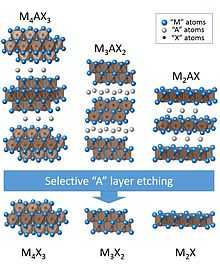
Producing a MXene by etching a MAX phase occurs mainly by using strong etching solutions that contain a fluoride ion (F−) such as hydrofluoric acid (HF),[2] ammonium bifluoride (NH4HF2),[4] and a mixture of hydrochloric acid (HCl) and lithium fluoride (LiF).[5] For example, etching of Ti3AlC2 in aqueous HF at room temperature causes the A (Al) atoms to be selectively removed, and the surface of the carbide layers becomes terminated by O, OH, and/or F atoms.[2][6][7]
The following MXenes have been synthesized to date:
2-1 MXenes: Ti2C,[8] (Ti0.5,Nb0.5)2C,[8] V2C,[9] Nb2C [9]
3-2 MXenes: Ti3C2 ,[1] Ti3CN [8]
4-3 MXenes: Nb4C3 ,[10] Ta4C3 [8]
Although theoretically possible, nitride-based MXenes have not yet been reported.[2]
Intercalation and delamination
Since MXenes are layered solids and the bonding between the layers is weak, intercalation of the guest molecules in MXenes is possible. Guest molecules include dimethyl sulfoxide (DMSO), hydrazine, and urea.[2] For example, N2H4 (hydrazine) can be intercalated into Ti3C2(OH)2 with the molecules parallel to the MXene basal planes to form a monolayer. Intercalaction increases the MXene c lattice parameter (crystal structure parameter that is directly proportional to the distance between individual MXene layers), which weakens the bonding between MX layers.[2] Ions, including Li+, Pb2+, and Al3+, can also be intercalated into MXenes, either spontaneously or when a negative potential is applied to a MXene electrode.[11]
Delamination
Ti3C2 MXene produced by HF etching has accordion-like morphology with residual forces that keep MXene layers together preventing separation into individual layers. Although those forces are quite weak, ultrasound treatment results only in very low yields of single-layer flakes. For large scale delamination, DMSO is intercalated into ML-MXene powders under constant stirring to further weaken the interlayer bonding and then delaminated with ultrasound treatment. This results in large scale layer separation and formation of the colloidal solutions of the FL-MXene. These solutions can later be filtered to prepare MXene "paper" (similar to Graphene oxide paper).[12]
MXene clay
For the case of Ti3C2Tx and Ti2CTx, etching with concentrated hydrofluoric acid leads to open, accordion-like morphology with a compact distance between layers (this is common for other MXene compositions as well). To be dispersed in suspension, the material must be pre-intercalated with something like dimethylsulfoxide. However, when etching is conducted with hydrochloric acid and LiF as a fluoride source, morphology is more compact with a larger inter-layer spacing, presumably due to amounts of intercalated water.[5] The material has been found to be ‘clay-like’: as seen in clay materials (a family including minerals such as smectite and kaolinite), this Ti3C2 demonstrates the ability to expand distance between the layers upon water vapor pressure increase due to water uptake between MXene layers. Further, when hydrated, the MXene clay becomes pliable and can be molded into desired shapes, becoming a hard solid upon drying. Unlike most clays, however, MXene clay shows high electrical conductivity upon drying and is hydrophilic, being easily dispersed into single layer two-dimensional sheets in water without surfactants. Further, due to these properties, it can be quickly rolled into freestanding, additive-free electrodes for energy storage applications.
Properties
With a high electron density near the Fermi level, MXene monolayers are predicted to be metallic.[13][14][15][16][17] In MAX phases, N(EF) is mostly M 3d orbitals, and the valence states below EF are composed of two sub-bands. One, sub-band A, made of hybridized Ti 3d-Al 3p orbitals, is near EF, and another, sub-band B, -10 to -3 eV below EF which is due to hybridized Ti 3d-C 2p and Ti 3d-Al 3s orbitals. Said differently, sub-band A is the source of Ti-Al bonds, while sub-band B is the source of Ti-C bond. Removing A layers causes the Ti 3d states to be redistributed from missing Ti-Al bonds to delocalized Ti-Ti metallic bond states near the Fermi energy in Ti2, therefore N(EF) is 2.5-4.5 times higher for MXenes than MAX phases.[1]
Only bare-surface MXenes are magnetic. Cr2C, Cr2N, and Ta3C2 are ferromagnetic, Ti3C2 and Ti3N2 are antiferromagnetic.[1]
Applications
MXenes, as conductive layered materials with tunable surface terminations, have been shown to be promising for energy storage applications (Li-ion batteries and supercapacitors), composites, photocatalysis[18] and gas sensors.[19]
Lithium-ion Batteries (LIBs)
Some MXenes have been investigated experimentally thus far in LIBs (e.g. V2CTx ,[20] Nb2CTx ,[20] Ti2CTx ,[21] and Ti3C2Tx [22]). V2CTx has demonstrated the highest reversible charge storage capacity among MXenes in multi-layer form (280 mAhg−1 at 1C rate and 125 mAhg−1 at 10C rate). Nb2CTx in multi-layer form showed a stable, reversible capacity of 170 mAhg−1 at 1C rate and 110 mAhg−1 at a 10C rate. Although Ti3C2Tx shows the lowest capacity among the four MXenes in multi-layer form, it can be easily delaminated via sonication of the multi-layer powder. By virtue of higher electrochemically active and accessible surface area, delaminated Ti3C2Tx paper demonstrates a reversible capacity of 410 mAhg−1 at 1C and 110 mAhg−1 at 36C rate. As a general trend, M2X MXenes can be expected to have greater capacity than their M3X2 or M4X3 counterparts at the same applied current, since M2X MXenes have the least number of atomic layers per sheet.
In addition to the high power capabilities of MXenes, each MXene has a different active voltage window, which could allow their use as cathodes or anodes in batteries. Moreover, the experimentally measured capacity for Ti3C2Tx paper is higher than predicted from computer simulations, indicating that further investigation is required to ascertain the charge storage mechanism on MXene surfaces.[23]
Supercapacitors
Supercapacitor electrodes based on Ti3C2 MXene paper in aqueous solutions demonstrate excellent cyclability and the ability to store 300-400 F/cm3, which translates to three times as much energy as for activated carbon and graphene-based capacitors.[24] Ti3C2 MXene clay shows a volumetric capacitance of 900 F/cm3, a higher capacitance per unit of volume than most other materials, and does not lose any of its capacitance through more than 10,000 charge/discharge cycles.[5]
Composites
FL-Ti3C2 (the most studied MXene) nanosheets can mix intimately with polymers such as polyvinyl alcohol (PVA), forming alternating MXene-PVA layered structures. The electrical conductivities of the composites can be controlled from 4*10−4 to 220 S/cm (MXene weight content from 40% to 90%). The composites have tensile strength up to 400% stronger than pure MXene films and show better capacitance up to 500 F/cm3.[25] A method of alternative filtration for forming MXene-carbon nanomaterials composite films is also devised. These composites show better rate performance at high scan rates in supercapacitors.[26] The intsertion of polymers or carbon nanomaterials between the MXene layers enables electrolyte ions to diffuse more easily through the MXenes, which is the key for their applications in flexible energy storage devices.
References
- ↑ 1.0 1.1 1.2 1.3 Naguib, M.; Kurtoglu, M.; Presser, V.; Lu, J.; Niu, J.; Gogotsi, Y.; Barsoum, M.W. "Two-Dimensional Nanocrystals Produced by Exfoliation of Ti3AlC2". Advanced Materials 23 (37): 4248–4253. doi:10.1002/adma.201102306.
- ↑ 2.0 2.1 2.2 2.3 2.4 2.5 2.6 2.7 Naguib, M.; Mochalin, V.N.; Barsoum, M.W.; Gogotsi, Y. "25th Anniversary Article: MXenes: A New Family of Two-Dimensional Materials". Advanced Materials 26 (7): 992–1005. doi:10.1002/adma.201304138.
- ↑ Sun, Z.; Music, D.; Ahuja, R.; Li, S.; Schneider, J.M. "Bonding and classification of nanolayered ternaray carbides". Physical Review B 70 (9): 092102. doi:10.1103/PhysRevB.70.092102.
- ↑ Halim, J.; Lukatskaya, M.; Cook, K.; Lu, J.; Smith, C. R.; Näslund, L.-Å.; May, S.J.; Hultman, L.; Gogotsi, Y.; Eklund, P.; Barsoum, M. W. "Transparent Conductive Two-Dimensional Titanium Carbide Epitaxial Thin Films". Chemistry of Materials 26 (7): 2374–2381. doi:10.1021/cm500641a.
- ↑ 5.0 5.1 5.2 Ghidiu, M.; Lukatskaya, M.R.; Zhao, M.-Q.; Gogotsi, Y.; Barsoum, M.W. "Conductive two-dimensional titanium carbide ‘clay’ with high volumetric capacitance". Nature 516 (7529): 78–81. doi:10.1038/nature13970.
- ↑ Naguib, M.; Mashtalir, O.; Carle, J.; Presser, V.; Lu, J.; Hultman, L.; Gogotsi, Y.; Barsoum, M.W. "Two-Dimensional Transition Metal Carbides". ACS Nano 6 (2): 1322–1331. doi:10.1021/nn204153h.
- ↑ Naguib, M.; Halim, J.; Lu, J.; Cook, K.M.; Hultman, L.; Gogotsi, Y.; Barsoum, M.W. "New two-dimensional niobium and vanadium carbides as promising materials for Li-ion batteries". J. Am. Chem. Soc. 135 (43): 15966–15969. doi:10.1021/ja405735d.
- ↑ 8.0 8.1 8.2 8.3 Naguib, M.; Mashtalir, O.; Carle, J.; Presser, V.; Lu, J.; Gogotsi, Y.; Barsoum, M.W. "Two-Dimensional Transition metal Carbides". ACS Nano 6 (2): 1322–1331. doi:10.1021/nn204153h.
- ↑ 9.0 9.1 Naguib, M.; Halim, J.; Lu, J.; Cook, K.M.; Hultman, L.; Gogotsi, Y.; Barsoum, M.W. "New Two-dimensional Niobium and Vanadium Carbides as Promising Materials for Li-ion Batteries". Journal of the American Chemical Society 135 (43): 15966–15969. doi:10.1021/ja405735d.
- ↑ Ghidiu, M.; Naguib, M.; Shi, C.; Mashtalir, O.; Pan, L.M.; Zhang, B.; Yang, J.; Gogotsi, Y.; Billinge, S.J.L.; Barsoum, M.W. "Synthesis and characterization of two-dimensional Nb4C3 (MXene)". Chemical Communications 50 (67): 9517–9520. doi:10.1039/C4CC03366C.
- ↑ Eames, C.; Islam, M.S. "Ion Intercalation into Two-Dimensional Transition-Metal Carbides: Global Screening for New High-Capacity Battery Materials". J. Am. Chem. Soc. 136 (46): 16270–16276. doi:10.1021/ja508154e.
- ↑ Mashtalir, O.; Naguib, M.; Mochalin, V.; Dall’Agnese, Y.; Heon, M.; Barsoum, M.; Gogotsi, Y. "Intercalation and delamination of layered carbides and carbonitrides". Nature Communications 4: 1716. doi:10.1038/ncomms2664.
- ↑ Naguib, M.; Kurtoglu, M.; Presser, V.; Lu, J; Niu, J.; Heon, M.; Hultman, L.; Gogotsi, Y.; Barsoum, M.W. "Two-Dimensional Nanocrystals Produced by Exfoliation of Ti3AlC2". Advanced Materials 23 (37): 4248–4253. doi:10.1002/adma.201102306.
- ↑ Enyashin, A.N.; Ivanovskii, A.L. "Structural and Electronic Properties and Stability of MXenes Ti2C and Ti3C2 Functionalized by Methoxy Groups". J Phys. Chem. C 117 (26): 13637–13643. doi:10.1021/jp401820b.
- ↑ Tang, Q.; Zhou, Z.; Shen, P. "Are MXenes promising anode materials for Li ion batteries? Computational studies on electronic properties and Li storage capability of Ti3C2 and Ti3C2X2 (X = F, OH) monolayer.". J. Am. Chem. Soc. 134 (40): 16909–16916. doi:10.1021/ja308463r.
- ↑ Khazaei, M.; Arai, M.; Sasaki, T.; Chung, C.-Y.; Venkataramanan, N.S.; Estili, M.; Sakka, Y. W.; Kawazoe, Y. "Novel Electronic and Magnetic Properties of Two-Dimensional Transition Metal Carbides and Nitrides". Adv. Funct. Mater. 23 (17): 2185–2192. doi:10.1002/adfm.201202502.
- ↑ Xie, Y.; Kent, P.R.C. "Hybrid density functional study of structural and electronic properties of functionalized Tin+1Xn (X=C, N) monolayers". Phys Rev. B 87 (23): 235441. doi:10.1103/PhysRevB.87.235441.
- ↑ Mashtalir, O.; Cook, K. M.; Mochalin, V. N.; Crowe, M.; Barsoum, M. W.; Gogotsi, Y. "Dye adsorption and decomposition on two-dimensional titanium carbide in aqueous media". J. Mater. Chem. A 2 (35): 14334–14338. doi:10.1039/C4TA02638A.
- ↑ Chen, J.; Chen, K.; Tong, D.; Huang, Y.; Zhang, J.; Xue, J.; Huang, Q.; Chen, T. "CO and temperature dual responsive "Smart" MXene phases". Chem. Commun. 51 (2): 314–317. doi:10.1039/C4CC07220K.
- ↑ 20.0 20.1 Naguib, M.; Halim, J.; Lu, J.; Cook, K.M.; Hultman, L.; Gogotsi, Y.; Barsoum, M.W. "New Two-Dimensional Niobium and Vanadium Carbides as Promising Materials for Li-Ion Batteries". J. Am. Chem. Soc. 135 (43): 15966–15969. doi:10.1021/ja405735d.
- ↑ Naguib, M.; Come, J.; Dyatkin, B.; Presser, V.; Taberna, P.-L.; Simon, P.; Barsoum, M.W.; Gogotsi, Y. "MXene: a promising transition metal carbide anode for lithium-ion batteries". Electrochemistry Communications 16 (1): 61–64. doi:10.1016/j.elecom.2012.01.002.
- ↑ Mashtalir, O.; Naguib, M.; Dall'Agnese, Y.; Heon, M.; Barsoum, M.W.; Gogotsi, Y. "Intercalation and delamination of layered carbides and carbonitrides". Nature Communications 4: 1716. doi:10.1038/ncomms2664.
- ↑ Xie, Yu; Naguib, M.; Mochalin, V.N.; Barsoum, M.W.; Gogotsi, Y.; Yu, X.; Nam, K.-W.; Yang, X.-Q.; Kolesnikov, A.I.; Kent, P.R.C. "Role of Surface Structure on Li-Ion Energy Storage Capacity of Two-Dimensional Transition-Metal Carbides". Journal of the American Chemical Society 136 (17): 6385–6394. doi:10.1021/ja501520b.
- ↑ Lukatskaya, M. R.; Mashtalir, O.; Ren, C. E.; Dall'Agnese, Y.; Rozier, P.; Taberna, P. L.; Naguib, M.; Simon, P.; Barsoum, M. W.; Gogotsi, Y. "Cation Intercalation and High Volumetric Capacitance of Two-Dimensional Titanium Carbide". Science 341 (6153): 1502–1505. doi:10.1126/science.1241488.
- ↑ Ling, Z.; Ren, C. E.; Zhao, M.-Q.; Yang, J.; Giammarco, J. M.; Qiu, J.; Barsoum, M. W.; Gogotsi, Y. "Flexible and conductive MXene films and nanocomposites with high capacitance". Proceedings of the National Academy of Sciences 111 (47): 16676–16681. doi:10.1073/pnas.1414215111.
- ↑ Zhao, M.-Q.; Ren, C. E.; Ling, Z.; Lukatskaya, M. R.; Zhang, C.; Van Aken, K. L.; Barsoum, M. W.; Gogotsi, Y. "Flexible MXene/Carbon Nanotube Composite Paper with High Volumetric Capacitance". Advanced Materials 27 (2): 339–345. doi:10.1002/adma.201404140.