Geology of Mercury

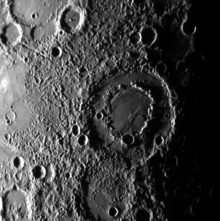
Of all the terrestrial planets in the Solar System, the geology of Mercury is the least understood. This stems largely from Mercury's proximity to the Sun which makes reaching it with spacecraft technically challenging and Earth-based observations difficult.
Mercury's surface is dominated by impact craters, basaltic rock and smooth plains, many of them a result of flood volcanism, similar in some respects to the lunar maria,[1][2] and locally by pyroclastic deposits,[3] Other notable features include vents which appear to be the source of magma-carved valleys, often-grouped irregular-shaped depressions termed "hollows" that are believed to be the result of collapsed magma chambers,[4] scarps indicative of thrust faulting and mineral deposits (possibly ice) inside craters at the poles. Long thought to be geologically inactive, new evidence suggests there may still be some level of activity.[5][6]
Mercury's density implies a solid iron-rich core that accounts for about 60% of its volume (75% of its radius).[7] Mercury's magnetic equator is shifted nearly 20% of the planet's radius towards the north, the largest ratio of all planets.[8] This shift lends to there being one or more iron-rich molten layers surrounding the core producing a dynamo effect similar to that of Earth. Additionally, the offset magnetic dipole may result in uneven surface weathering by the solar wind, knocking more surface particles up into the southern exosphere and transporting them for deposit in the north. Scientists are gathering telemetry to determine if such is the case.[8]
After having completed the first solar day of its mission in September 2011, more than 99% of Mercury's surface had been mapped by NASA's MESSENGER probe in both color and monochrome with such detail that scientists' understanding of Mercury's geology has eclipsed the level achieved following the Mariner 10 flybys of the 1970s.[4]
Difficulties in exploration

Reaching Mercury from Earth poses significant technical challenges, because the planet orbits so much closer to the Sun than does the Earth. A Mercury-bound spacecraft launched from Earth must travel 91 million kilometers into the Sun’s gravitational potential well. Starting from the Earth’s orbital speed of 30 km/s, the change in velocity (delta-v) the spacecraft must make to enter into a Hohmann transfer orbit that passes near Mercury is large compared to other planetary missions. The potential energy liberated by moving down the Sun’s potential well becomes kinetic energy; requiring another large delta-v to do anything other than rapidly pass by Mercury. In order to land safely or enter a stable orbit the spacecraft must rely entirely on rocket motors since aerobraking is ruled out because the planet has very little atmosphere. A direct trip to Mercury actually requires more rocket fuel than that required to escape the Solar System completely. As a result, only two space probes, Mariner 10 and MESSENGER, which are both NASA, have flown-by the planet so far.

2. Mantle - 600 km thick
3. Nucleus - 1,800 km radius
Furthermore, the space environment near Mercury is demanding, posing the double dangers to spacecraft of intense solar radiation and high temperatures.
Historically, a second obstacle has been that Mercury's period of rotation is a slow 58 Earth days, so that spacecraft flybys are restricted to viewing only a single illuminated hemisphere. In fact, unfortunately, even though Mariner 10 space probe flew past Mercury three times during 1974 and 1975, it observed the same area during each pass. This was because Mariner 10's orbital period was almost exactly 3 sidereal Mercury days, and the same face of the planet was lit at each of the close approaches. As a result, less than 45% of the planet’s surface was mapped.
Earth-based observations are made difficult by Mercury's constant proximity to the Sun. This has several consequences:
- Whenever the sky is dark enough for viewing through telescopes, Mercury is always already near the horizon, where viewing conditions are poor anyway due to atmospheric factors.
- The Hubble Space Telescope and other space observatories are usually prevented from pointing close to the Sun for safety reasons (Erroneously pointing such sensitive instruments at the Sun is likely to cause permanent damage).
Mercury's geological history

Like the Earth, Moon and Mars, Mercury's geologic history is divided up into eras. From oldest to youngest, these are: the pre-Tolstojan, Tolstojan, Calorian, Mansurian, and Kuiperian. These ages are based on relative dating only.[9]
After the formation of Mercury along with the rest of the Solar System 4.6 billion years ago, heavy bombardment by asteroids and comets ensued. The last intense bombardment phase, the Late Heavy Bombardment came to an end about 3.8 billion years ago. Some regions or massifs, a prominent one being the one that formed the Caloris Basin, were filled by magma eruptions from within the planet. These created smooth intercrater plains similar to the maria found on the Moon. Later, as the planet cooled and contracted, its surface began to crack and form ridges; these surface cracks and ridges can be seen on top of other features, such as the craters and smoother plains – a clear indication that they are more recent. Mercury's period of vulcanism ended when the planet's mantle had contracted enough to prevent further lava from breaking through to the surface. This probably occurred at some point during its first 700 or 800 million years of history.
Since then, the main surface processes have been intermittent impacts.
Timeline

Surface features
Mercury’s surface is overall similar in appearance to that of the Moon, with extensive mare-like plains and heavily cratered terrains similar to the Lunar highlands and locally by accumulations of pyroclastic deposits.[3]
Topography |
---|
![]() lowest (purple) to 10 km (6.2 mi) highest (red). |
Impact basins and craters

Craters on Mercury range in diameter from small bowl-shaped craters to multi-ringed impact basins hundreds of kilometers across. They appear in all states of degradation, from relatively fresh rayed-craters, to highly degraded crater remnants. Mercurian craters differ subtly from Lunar craters — the extent of their ejecta blankets is much smaller, which is a consequence of the 2.5 times stronger surface gravity on Mercury.[9]
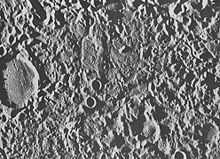
The largest known crater is the enormous Caloris Basin, with a diameter of 1550 km,[10] A basin of comparable size, tentatively named Skinakas Basin had been postulated from low resolution Earth-based observations of the non-Mariner-imaged hemisphere, but has not been observed in MESSENGER imagery of the corresponding terrain. The impact which created the Caloris Basin was so powerful that its effects are seen on a global scale. It caused lava eruptions and left a concentric ring over 2 km tall surrounding the impact crater. At the antipode of the Caloris Basin lies a large region of unusual, hilly and furrowed terrain, sometimes called “Weird Terrain”. The favoured hypothesis for the origin of this geomorphologic unit is that shock waves generated during the impact traveled around the planet, and when they converged at the basin’s antipode (180 degrees away) the high stresses were capable of fracturing the surface.[11] A much less favoured idea was that this terrain formed as a result of the convergence of ejecta at this basin’s antipode. Furthermore, the formation of the Caloris Basin appears to have produced a shallow depression concentric around the basin, which was later filled by the smooth plains (see below).
Overall about 15 impact basins have been identified on the imaged part of Mercury. Other notable basins include the 400 km wide, multi-ring, Tolstoj Basin which has an ejecta blanket extending up to 500 km from its rim, and its floor has been filled by smooth plains materials. Beethoven Basin also has a similar-sized ejecta blanket and a 625 km diameter rim.[9]
As on the Moon, fresh craters on Mercury show prominent bright ray systems. These are made by ejected debris, which while they remain relatively fresh tend to be brighter because of a lesser amount of space weathering than the surrounding older terrain.
Pit-floor craters
Some impact craters on Mercury have non-circular, irregularly shaped depressions or pits on their floors. Such craters have been termed pit-floor craters, and MESSENGER team members have suggested that such pits formed by the collapse of subsurface magma chambers. If this suggestion is correct, the pits are evidence of volcanic processes at work on Mercury.[6] Pit craters are rimless, often irregularly shaped, and steep-sided, and they display no associated ejecta or lava flows but are typically distinctive in color. For example, the pits of Praxiteles have an orange hue.[12] Thought to be evidence of shallow magmatic activity, pit craters may have formed when subsurface magma drained elsewhere and left a roof area unsupported, leading to collapse and the formation of the pit. Major craters exhibiting these features include Beckett, Gibran and Lermontov, among others.[13] It was suggested that these pits with associated brighter and redder deposits may be pyroclastic deposits caused by explosive volcanism.[3]

Plains
There are two geologically distinct plains units on Mercury:[9][14]
- Inter-crater plains are the oldest visible surface,[9] predating the heavily cratered terrain. They are gently rolling or hilly and occur in the regions between larger craters. The inter-crater plains appear to have obliterated many earlier craters, and show a general paucity of smaller craters below about 30 km in diameter.[14] It is not clear whether they are of volcanic or impact origin.[14] The inter-crater plains are distributed roughly uniformly over the entire surface of the planet.
- Smooth plains are widespread flat areas resembling the lunar maria, which fill depressions of various sizes. Notably, they fill a wide ring surrounding the Caloris Basin. An appreciable difference to the lunar maria is that the smooth plains of Mercury have the same albedo as the older intercrater plains. Despite a lack of unequivocally volcanic features, their localisation and lobate-shaped colour units strongly support a volcanic origin. All the Mercurian smooth plains formed significantly later than the Caloris basin, as evidenced by appreciably smaller crater densities than on the Caloris ejecta blanket.[9]
The floor of the Caloris Basin is also filled by a geologically distinct flat plain, broken up by ridges and fractures in a roughly polygonal pattern. It is not clear whether they are volcanic lavas induced by the impact, or a large sheet of impact melt.[9]
Tectonic features
One unusual feature of the planet’s surface is the numerous compression folds which crisscross the plains. It is thought that as the planet’s interior cooled, it contracted and its surface began to deform. The folds can be seen on top of other features, such as craters and smoother plains, indicating that they are more recent.[15] Mercury’s surface is also flexed by significant tidal bulges raised by the Sun—the Sun’s tides on Mercury are about 17% stronger than the Moon’s on Earth.[16]
Terminology
Non-crater surface features are given the following names:
- Albedo features — areas of markedly different reflectivity
- Dorsa — ridges (see List of ridges on Mercury)
- Montes — mountains (see List of mountains on Mercury)
- Planitiae — plains (see List of plains on Mercury)
- Rupes — scarps (see List of scarps on Mercury)
- Valles — valleys (see List of valleys on Mercury)
High-albedo polar patches and possible presence of ice
The first radar observations of Mercury were carried out by the radiotelescopes at Arecibo (Puerto Rico) and Goldstone (California, United States), with assistance from the U.S. National Radio Astronomy Observatory Very Large Array (VLA) facility in New Mexico. The transmissions sent from the NASA Deep Space Network site at Goldstone were at a power level of 460 kW at 8.51 GHz; the signals received by the VLA multi-dish array detected points of radar reflectivity (radar luminosity) with depolarized waves from Mercury's north pole.

Radar maps of the surface of the planet were made using the Arecibo radiotelescope. The survey was conducted with 420 kW UHF band (2.4 GHz) radio waves which allowed for a 15 km resolution. This study not only confirmed the existence of the zones of high reflectivity and depolarization, but also found a number of new areas (bringing the total to 20) and was even able to survey the poles. It has been postulated that surface ice may be responsible for these high luminosity levels, as the silicate rocks that compose most of the surface of Mercury have exactly the opposite effect on luminosity.
In spite of its proximity to the Sun, Mercury may have surface ice, since temperatures near the poles are constantly below freezing point: On the polar plains, the temperature does not rise above -106 °C. And craters at Mercury's higher latitudes (discovered by radar surveys from Earth as well) may be deep enough to shield the ice from direct sunlight. Inside the craters, where there is no solar light, temperatures fall to -171 °C.[17]
Despite sublimation into the vacuum of space, the temperature in the permanently shadowed region is so low that this sublimation is slow enough to potentially preserve deposited ice for billions of years.
At the South Pole, the location of a large zone of high reflectivity coincides with the location of the Chao Meng-Fu crater, and other small craters containing reflective areas have also been identified. At the North Pole, a number of craters smaller than Chao-Meng Fu have these reflective properties.
The strength of the radar reflections seen on Mercury are small compared to that which would occur with pure ice. This may be due to powder deposition that does not cover the surface of the crater completely or other causes, e.g. a thin overlying surface layer. However, the evidence for ice on Mercury is not definitive. The anomalous reflective properties could also be due to the existence of deposits of metallic sulfates or other materials with high reflectance.
Possible origin of ice
Mercury is not unique in having craters that stand in permanent shadow; at the south pole of Earth's Moon there is a large crater (Aitken) where some possible signs of the presence of ice have been seen (although their interpretation is disputed). It is thought by astronomers that ice on both Mercury and the Moon must have originated from external sources, mostly impacting comets. These are known to contain large amounts, or a majority, of ice. It is therefore conceivable for meteorite impacts to have deposited water in the permanently shadow craters, where it would remain unwarmed for possibly billions of years due to the lack of an atmosphere to efficiently conduct heat and stable orientation of Mercury's rotation axis.
Mercury |
---|
![]() |
See also
References
- ↑ "Solar System Exploration: Mercury". NASA. Retrieved 17 Feb 2012.
- ↑ "MESSENGER Team Presents New Mercury Findings". NASA. Retrieved 16 Feb 2012.
- ↑ 3.0 3.1 3.2 Thomas, Rebecca J.; Rothery, David A.; Conway, Susan J.; Anand, Mahesh (16 September 2014). "Long-lived explosive volcanism on Mercury". Geophysical Research Letters 41 (17): 6084–6092. doi:10.1002/2014GL061224.
- ↑ 4.0 4.1 "Orbital Observations of Mercury". Johns Hopkins University Applied Physics Lab. Retrieved 16 Feb 2012.
- ↑ "The MESSENGER Gamma-Ray Spectrometer: A window into the formation and early evolution of Mercury". Johns Hopkins University Applied Physics Lab. Retrieved 18 Feb 2012.
- ↑ 6.0 6.1 "Evidence of Volcanism on Mercury: It's the Pits". Johns Hopkins University Applied Physics Lab. Retrieved 16 Feb 2012.
- ↑ "Mercury: The Key to Terrestrial Planet Evolution". Johns Hopkins University Applied Physics Lab. Retrieved 18 Feb 2012.
- ↑ 8.0 8.1 "Mercury's Oddly Offset Magnetic Field". Johns Hopkins University Applied Physics Lab. Retrieved 18 Feb 2012.
- ↑ 9.0 9.1 9.2 9.3 9.4 9.5 9.6 P. D. Spudis (2001). "The Geological History of Mercury". Workshop on Mercury: Space Environment, Surface, and Interior, Chicago: 100. Bibcode:2001mses.conf..100S.
- ↑ Shiga, David (30 January 2008). "Bizarre spider scar found on Mercury's surface". NewScientist.com news service.
- ↑ Schultz P.H., Gault D.E. (1975), Seismic effects from major basin formations on the moon and Mercury, The Moon, vol. 12, Feb. 1975, p. 159-177
- ↑ "Overlaying Color onto Praxiteles Crater". Johns Hopkins University Applied Physics Lab. Retrieved 16 Feb 2012.
- ↑ "A Newly Pictured Pit-Floor Crater". Johns Hopkins University Applied Physics Lab. Retrieved 16 Feb 2012.
- ↑ 14.0 14.1 14.2 R.J. Wagner et al. (2001). "Application of an Updated Impact Cratering Chronology Model to Mercury's Time-Stratigraphic System". Workshop on Mercury: Space Environment, Surface, and Interior, Chicago: 106. Bibcode:2001mses.conf..106W.
- ↑ Dzurisin D. (1978), The tectonic and volcanic history of Mercury as inferred from studies of scarps, ridges, troughs, and other lineaments, Journal of Geophysical Research, v. 83, p. 4883-4906
- ↑ Van Hoolst, T., Jacobs, C. (2003), Mercury’s tides and interior structure, Journal of Geophysical Research, v. 108, p. 7.
- ↑ "Ice on Mercury". National Space Science Data Center. Retrieved 16 Feb 2012.
- Stardate, Guide to the Solar System. Publicación de la University of Texas at Austin McDonald Observatory
- Our Solar System, A Geologic Snapshot. NASA (NP-157). May 1992.
- Fotografía: Mercury. NASA (LG-1997-12478-HQ)
- This article draws heavily on the corresponding article in the Spanish-language Wikipedia, which was accessed in the version of 26 June 2005.
Original references for Spanish article
- Ciencias de la Tierra. Una Introducción a la Geología Física (Earth Sciences, an Introduction to Physical Geology), by Edward J. Tarbuck y Frederick K. Lutgens. Prentice Hall (1999).
- "Hielo en Mercurio" ("Ice on Mercury"). El Universo, Enciclopedia de la Astronomía y el Espacio ("The Universe, Encyclopedia of Astronomy and the Space"), Editorial Planeta-De Agostini, p. 141-145. Volume 5. (1997)
External links
- Mariner 10
- MESSENGER probe
- Mercury on Nineplanets.org
- USGS Geology of Mercury Retrieved 5 August 2007