Arthropod eye
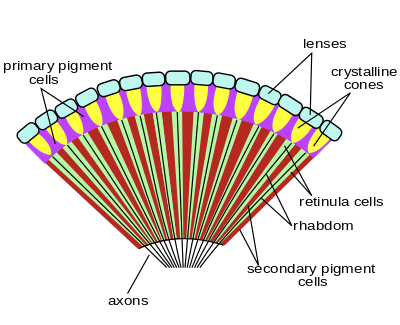
The arthropods ancestrally possessed compound eyes, but the type and origin of this eye varies between groups, and some taxa have secondarily developed simple eyes. The organ's development through the lineage can be estimated by comparing groups that branched early, such as the velvet worm and horseshoe crab to the advanced eye condition found in insects and other derived arthropods.
Apposition eyes are the most common form of eye, and are presumably the ancestral form of compound eye. They are found in all arthropod groups, although they may have evolved more than once within this phylum.[1] Some annelids and bivalves also have apposition eyes. They are also possessed by Limulus, the horseshoe crab, and there are suggestions that other chelicerates developed their simple eyes by reduction from a compound starting point.[1] Some caterpillars appear to have evolved compound eyes from simple eyes in the opposite fashion.
Eyes and functions

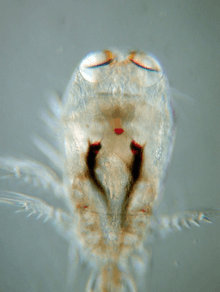
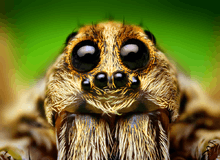

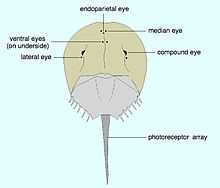
Most arthropods have at least one of two types of eye: lateral compound eyes, and smaller median ocelli, which are simple eyes.[5] When both are present, the two eye types are used in concert because each has its own advantage.[6] Flying insects can remain level with either type of eye surgically removed, but the two types combine to give better performance.[6] Ocelli can detect lower light levels,[note 1][7] and have a faster response time, while compound eyes are better at detecting edges and are capable of forming images.[6]
Genetic controls
The head patterning is controlled by orthodenticle, a homeobox gene which demarcates the segments from the top-middle of the head to the more lateral aspects. The ocelli are in an orthrodenticle-rich area, and the gene is not expressed by the time one gets as lateral as the compound eyes.[8]
The gene dachshund is involved in the development of the compound eye.[9]
Different opsins are used in the ocelli to the compound eyes.[10]
Evolution
Hexapods are currently thought to fall within the Crustacean crown group; while molecular work paved the way for this association, their eye morphology and development is also markedly similar.[11] The eyes are strikingly different from the myriapods, which were traditionally considered to be a sister group to the hexapoda.
Both ocelli and compound eyes were probably present in the last common arthropod ancestor,[12] and may be apomorphic with ocelli in other phyla,[13] such as the annelids.[14] Median ocelli are present in chelicerates and mandibulates; lateral ocelli are also present in chelicerates.[13]
Origin
No fossil organisms have been identified as similar to the last common ancestor of arthropods; hence the eyes possessed by the first arthropod remains a matter of conjecture. The largest clue into their appearance comes from the onychophorans: a stem group lineage that diverged soon before the first true arthropods. The eyes of these creatures are attached to the brain using nerves which enter into the centre of the brain, and there is only one area of the brain devoted to vision. This is similar to the wiring of the median ocelli (small simple eyes) possessed by many arthropods; the eyes also follow a similar pathway through the early development of organisms. This suggests that onychophoran eyes are derived from simple ocelli, and the absence of other eye structures implies that the ancestral arthropod lacked compound eyes, and only used median ocelli to sense light and dark.[5] However, a conflicting view notes that compound eyes appeared in many early arthropods, including the trilobites and eurypterids, suggesting that the compound eye may have developed after the onychophoran and arthropod lineages split, but before the radiation of arthropods.[13] This view is supported if a stem-arthropod position is supported for compound-eye bearing Cambrian organisms such as the Anomalocaridids. An alternative, however, is that compound eyes evolved multiple times among the arthropods.[14]
There were probably only a single pair of ocelli in the arthropod concestor; Cambrian lobopod fossils display a single pair, and while many arthropods today have three, four, or even six, the lack of common pathway suggests that a pair is the most probable ancestral state. The crustaceans and insects mainly have three ocelli, suggesting that such a formation was present in their concestor.[5]
It is deemed probable that the compound eye arose as a result of the 'duplication' of individual ocelli.[13] In turn, the dispersal of compound eyes seems to have created large networks of seemingly independent eyes in some arthropods, such as the larvae of certain insects.[13] In some other insects and myriapods, lateral ocelli appear to have arisen by the reduction of lateral compound eyes.[13]
Trilobite eyes
The eyes of trilobites came in three forms. The holochroal eye, the most common and most primitive, consisted of many small lenses, between 100-15000, covered by a single corneal membrane. This was the most ancient kind of eye. This eye morphology was found in the Cambrian trilobites (the earliest) and survived until the Permian extinction.[15]
The more complex schizochroal eye was found only in one sub-order of trilobite, the Phacopina (Ordovician-Silurian). It has no modern counterpart. The eye has up to 700 larger lenses with individual sclera separating each lens. Each lens has a cornea. Schizochroal eyes developed from holochroal ones and were more powerful with overlapping visual fields. They were particularly useful for nocturnal vision and possibly for colour and depth analysis. The lenses of the eye were constructed from single calcite crystals. Early schizochroal eye designs were rather haphazard and irregular, though constrained by the geometrical requirements of packing identical sized lenses on a curved surface. Later designs used graduating lens sizes.[15]
The third eye morphology of trilobites, called the abathochroal, was found only within the Eodiscina. This morphology consisted of up to 70 much smaller lenses. The cornea separated each lens, and the sclera on each lens terminated on top of each cornea. The eye morphology of trilobites is useful for determining their mode of life, and can function as palaeoenvironmental indicators.[15]
Horseshoe crab
The horseshoe crab has traditionally been used in investigations into the eye, because it has relatively large ommatidia with large nerve fibres (making them easy to experiment on). It also falls in the stem group of the chelicerates; its eyes are believed to represent the ancestral condition because they have changed so little over evolutionary time. Indeed the horseshoe crabs are often considered to be living fossils. Most other living chelicerates have lost their lateral compound eyes, evolving simple eyes in their place.[16]
Horseshoe crabs have two large compound eyes on the sides of its head. An additional simple eye is positioned at the rear of each of these structures.[16] In addition to these obvious structures, it also has two smaller ocelli situated in the middle-front of its carapace, which may superficially be mistaken for nostrils.[16] A further simple eye is located beneath these, on the underside of the carapace.[16] A further pair of simple eyes are positioned just in front of the mouth.[16] The simple eyes are probably important during the embryonic or larval stages of the organism, with the compound eyes and median ocelli becoming the dominant sight organisms during adulthood.[16] These ocelli are less complex, and probably less derived, than those of the mandibulata.[13] Unlike the trilobites', the compound eyes of horseshoe crabs are triangular in shape; they also have a generative region at their base, but this elongates with time. Hence the one ommatidium at the apex of the triangle was the original "eye" of the larval organism, with subsequent rows added as the organism grew.[11]
Insects and crustaceans
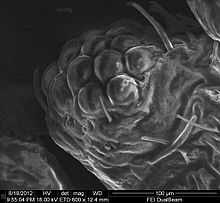
These two groups are probably monophyletic; their eyes certainly develop in a very similar fashion. While most crustacean and insect larvae possess only simple median eyes, including the Bolwig's organ pit-eye of Drosophila and the naupliar eye of most crustaceans, several groups have larvae with compound lateral eyes. The compound eyes of adults develop in a separate region of the head from the larval median eye.[11] New ommatidia are added in semicircular rows at the rear of the eye; during the first phase of growth, this leads to individual ommatidia being square, but later in development they become hexagonal. The hexagonal pattern will only become visible when the carapace of the stage with square eyes is molted.[11]
Both crustaceans and insects can have stalked eyes on peduncles.
Myriapods
Most myriapods bear stemmata - that is, single lensed eyes which evolved by the reduction of a compound eye.[13] However, the genus Scutigera has secondarily re-evolved a compound eye composed of repeated stemmata.[14] These appear to grow in rows which are inserted between existing rows of ocelli.[11]
See also
- Compound eye
- Mollusc eye
- Parietal eye
- Simple eye in invertebrates
- Vision in fish
Notes
- ↑ They are about 5000 times more sensitive than apposition compound eyes. They can, for instance, respond to the position of the full moon
References
- ↑ 1.0 1.1 M F Land; R D Fernald (1992), "The Evolution of Eyes", Annual Review of Neuroscience 15 (1): 1–29, doi:10.1146/annurev.ne.15.030192.000245, PMID 1575438.
- ↑ Schwab, Ivan R (2012) Evolution's Witness: How Eyes Evolved Page 231, Oxford University Press. ISBN 9780195369748.
- ↑ Miller, Charles B (2004) Biological Oceanography Page 122, John Wiley & Sons. ISBN 9780632055364.
- ↑ Barlow RB (2009) "Vision in horseshoe crabs" Pages 223–235 in JT Tanacredi, ML Botton and D Smith, Biology and Conservation of Horseshoe Crabs, Springer. ISBN 9780387899589.
- ↑ 5.0 5.1 5.2 Mayer, G. (2006), "Structure and development of onychophoran eyes: What is the ancestral visual organ in arthropods?", Arthropod Structure and Development 35 (4): 231–245, doi:10.1016/j.asd.2006.06.003, PMID 18089073
- ↑ 6.0 6.1 6.2 Taylor, Charles P. (1981), "Contribution of compound eyes and ocelli to steering of locusts in flight. I. Behavioural analysis", J Exp Biol: 1–18
- ↑ Wilson, M. (1978), "The functional organisation of locust ocelli", Journal of Comparative Physiology (4): 297–316
- ↑ Royet, J; Finkelstein, R (1995), "Pattern formation in Drosophila head development: the role of the orthodenticle homeobox gene", Development 121 (11): 3561–3572, PMID 8582270
- ↑ Mardon, G.; Solomon, N.M., N. M.; Rubin, G. M., G. M. (Dec 1994). "dachshund encodes a nuclear protein required for normal eye and leg development in Drosophila". Development 120 (12): 3473–3486. ISSN 0950-1991. PMID 7821215.
- ↑ S. Wehrhahn, William A. Zuker; Harris, WA; Kirschfeld, K; Wehrhahn, C; Zuker, CS (1988), "Targeted misexpression of a Drosophila opsin gene leads to altered visual function", Nature 333 (6175): 737, doi:10.1038/333737a0, PMID 2455230
- ↑ 11.0 11.1 11.2 11.3 11.4 Harzsch, S.; Hafner, G. (2006), "Evolution of eye development in arthropods: Phylogenetic aspects", Arthropod Structure and Development 35 (4): 319–340, doi:10.1016/j.asd.2006.08.009, PMID 18089079
- ↑ Friedrich, M (2006), "Ancient mechanisms of visual sense organ development based on comparison of the gene networks controlling larval eye, ocellus, and compound eye specification in Drosophila", Arthropod Structure and Development 35 (4): 357–378, doi:10.1016/j.asd.2006.08.010, PMID 18089081
- ↑ 13.0 13.1 13.2 13.3 13.4 13.5 13.6 13.7 Bitsch, C.; Bitsch, J. (2005), "Evolution of eye structure and arthropod phylogeny", Crustacean Issues 16: 185–214, doi:10.1201/9781420037548.ch8, ISBN 978-0-8493-3498-6
- ↑ 14.0 14.1 14.2 Paulus, H.F. (2000), "Phylogeny of the Myriapoda-Crustacea-Insecta: a new attempt using photoreceptor structure*", Journal of Zoological Systematics & Evolutionary Research 38 (3): 189–208, doi:10.1046/j.1439-0469.2000.383152.x
- ↑ 15.0 15.1 15.2 Clarkson, Euan (2009) Invertebrate Palaeontology and Evolution 4th Ed, John Wiley & Sons. ISBN 9781444313321.
- ↑ 16.0 16.1 16.2 16.3 16.4 16.5 Battelle, B.A. (December 2006), "The eyes of Limulus polyphemus (Xiphosura, Chelicerata) and their afferent and efferent projections.", Arthropod structure & development 35 (4): 261–74, doi:10.1016/j.asd.2006.07.002, PMID 18089075.
|