Propelling nozzle
A propelling nozzle is the component of a jet engine that operates to constrict the flow, to form an exhaust jet and to maximise the velocity of propelling gases from the engine.[1]
Propelling nozzles can be subsonic, sonic, or supersonic.[2] Physically the nozzles can be convergent, or convergent-divergent. Convergent-divergent nozzles can give supersonic jet velocity within the divergent section, whereas in a convergent nozzle the exhaust fluid cannot exceed the speed of sound within the nozzle.
Propelling nozzles can be fixed geometry, or they can have variable geometry, to give different throat and exit diameters so as to deal with differences in ambient pressure, flow and engine pressure; thus permitting improvement of thrust and efficiency.
Principles of operation
- A nozzle operates by using its narrowest part, or 'throat', to increase pressure within the engine by constricting airflow, usually until the flow chokes, then expanding the exhaust stream to, or near to, atmospheric pressure, while forming it into a high speed jet to propel the vehicle.
- The energy to accelerate the stream comes from the temperature and pressure of the gas- the gas expands adiabatically, when done against a nozzle, this largely reversibly (and hence efficiently) cools, expands, and accelerates the gas, with the heat and pressure of exhaust gas being proportional to its speed.
- Airbreathing engines create forward thrust on the airframe by imparting a net rearward momentum onto the air via producing a jet of exhaust gas, which, when fully expanded, has a speed that exceeds the aircraft's airspeed.
- Engines that are required to generate thrust quickly from idle use propelling nozzles with variable area. While at idle, the nozzle is set to its open configuration for minimum thrust and high engine rpm, but when thrust is needed, (e.g., while initiating a go-around) constricting the nozzle will quickly generate thrust.
Convergent nozzles
Almost all nozzles have a convergent section because it increases the pressure in the rest of the engine- potentially yielding more thrust by acting on the forward sections. It is important to note that convergent nozzles end with this convergent section, and in general, narrower convergent nozzles give lower thrust and higher exhaust speed, but wider convergent nozzles give lower exhaust speed and higher thrust.
Simple convergent nozzles are used on many jet engines. If the nozzle pressure ratio is above the critical value (about 1.8:1) a convergent nozzle will choke, resulting in some of the expansion to atmospheric pressure taking place downstream of the throat (i.e. smallest flow area), in the jet wake. Although jet momentum still produces much of the gross thrust, the imbalance between the throat static pressure and atmospheric pressure still generates some (pressure) thrust.
Divergent nozzle
The supersonic speed the of air flowing into a scramjet allows the use of a simple divergent nozzle.
Convergent-divergent nozzles
Engines capable of supersonic flight have convergent-divergent duct features to generate supersonic flow. Rocket engines — the extreme case — owe their distinctive shape to the their very high area ratios of their nozzles.
The high nozzle pressure ratios of convergent nozzles often cause the pressure of exhaust exiting the engine to exceed the pressure of the surrounding air and thereby reduce efficiency by causing much of the expansion to take place downstream of the nozzle itself. Consequently, some engines (notably, rockets) incorporate a convergent-divergent nozzle, which, by causing more of the exhaust to expand against the inside of the nozzle. However, unlike the fixed convergent-divergent nozzle used on a conventional rocket motor, those on turbojet engines must have heavy and expensive variable geometry to cope with the great variation in the nozzle pressure ratio that engine throttling creates.
Afterburners
Non-afterburning engines have nozzles of a fixed size because variable geometry nozzles are expensive and the change in atmospheric pressure with changing altitude has little effect on engine aerodynamics. However, the afterburner present on many combat aircraft's exhaust systems increases exhaust flow volume to the point that upstream turbomachinery can aerodynamically rematch; although some engines achieve maximum net thrust by allowing a modest rematch, all others must have a nozzle that can accommodate this upsurge by increasing its throat area. To accomplish this task, the nozzle consists of a series of moving, overlapping petals with a nearly circular nozzle cross-section.
Types of nozzles
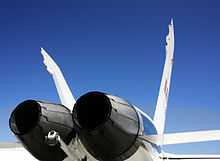
Ejector nozzles
The simpler of the two is the ejector nozzle, which creates an effective nozzle through a secondary airflow and spring-loaded petals. At subsonic speeds, the airflow constricts the exhaust to a convergent shape. As the aircraft speeds up, the two nozzles dilate, which allows the exhaust to form a convergent-divergent shape, speeding the exhaust gasses past Mach 1. More complex engines can actually use a tertiary airflow to reduce exit area at very low speeds. Advantages of the ejector nozzle are relative simplicity and reliability. Disadvantages are average performance (compared to the other nozzle type) and relatively high drag due to the secondary airflow. Notable aircraft to have utilized this type of nozzle include the SR-71, Concorde, F-111, and Saab Viggen

Iris nozzles
For higher performance, it is necessary to use an iris nozzle. This type uses overlapping, hydraulically adjustable "petals". Although more complex than the ejector nozzle, it has significantly higher performance and smoother airflow. As such, it is employed primarily on high-performance fighters such as the F-14, F-15, F-16, though it is also used in high-speed bombers such as the B-1B. Some modern iris nozzles additionally have the ability to change the angle of the thrust (see thrust vectoring).
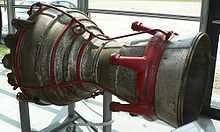
Rocket nozzles
Rocket motors also employ convergent-divergent nozzles, but these are usually of fixed geometry, to minimize weight. Because of the high pressure ratios used in rocketry, rocket motor con-di nozzles typically have a much greater area ratio (exit/throat) than those fitted to ducted jet engines.
The Convair F-106 Delta Dart has used such a nozzle design, as part of its overall design specification as an aerospace interceptor for high-altitude bomber interception, where conventional nozzle design would prove ineffective.
Low ratio nozzles
At the other extreme, some high bypass ratio civil turbofans control the fan working line by using a convergent-divergent nozzle with an extremely low (less than 1.01) area ratio on the bypass (or mixed exhaust) stream. At low airspeeds, such a setup causes the nozzle to act as if it had variable geometry by preventing it from choking and allowing it to accelerate and decelerate exhaust gas approaching the throat and divergent section, respectively. Consequently, the nozzle exit area controls the fan match, which, being larger than the throat, pulls the fan working line slightly away from surge. At higher flight speeds, the ram rise in the intake chokes the throat and causes the nozzle's area to dictate the fan match; the nozzle, being smaller than the exit, causes the throat to push the fan working line slightly toward surge. This is not a problem, however, for a fan's surge margin is much greater at high flight speeds.
Other Applications
Certain aircraft, like the German Bf-109 and the Macchi C.202/205 were fitted with "ejector-type exhausts". These exhausts converted some of the waste energy of the (internal combustion) engines exhaust-flow into a small amount of forward thrust by accelerating the hot gasses in a rearward direction to a speed greater than that of the aircraft. All exhaust setups do this to some extent, provided that the of exhaust-ejection vector is opposite/dissimilar to the direction of the aircraft movement.
See also
- De Laval nozzle
- Jet engine performance
- Rocket engine nozzles
References
|