Organoiridium compound
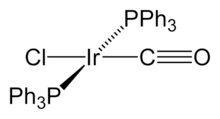

3.png)
Organoiridium compounds contain iridium-carbon chemical bonds. As a third row transition metal, iridium forms very stable bonds to carbon and the organometallic chemistry is extensive.
Examples
Iridium carbonyls
As for rhodium, the simplest iridium carbonyl, Ir2(CO)8 is not well characterized but tetrairidium dodecacarbonyl, Ir
4(CO)
12, is well known. which is the most common binary carbonyl of iridium.[1] In this compound each iridium centre is bonded to the three others, forming a tetrahedral cluster. Unlike Co4(CO)12, this Ir4 cluster exclusively features terminal carbonyl ligands. Some organometallic Ir(I) compounds are notable enough to be named after their discoverers. One is Vaska's complex, IrCl(CO)[P(C
6H
5)
3]
2, which has the unusual property of reversibly binding dioxygen molecule, O2.[2] The anionic carbonyl [IrI2(CO)2]2- is an intermediate in the Cativa Process discussed below.
Olefin complexes
Diene complexes of iridium are extensively developed, mainly starting from Ir2Cl2(cyclooctadiene)2. One noteworthy derivative is Crabtree's catalyst, a homogeneous catalyst for hydrogenation reactions.[3] Iridium(I) complexes are often square planar with a total of 16 valence electrons, which accounts for their reactivity.[4]
Cp and Cp* complexes
Many organoiridium compounds are generated from pentamethylcyclopentadienyl iridium dichloride dimer. The half-sandwich complexes were central in the development of C-H activation.[5][6]
- Organoiridium chemistry has been central to the development of C-H activation, two examples of which are shown here.
Iridocene, IrCp2, remains lightly studied because of lack of applications.[7] As with rhodocene, iridocene dimerises at room temperature.[8]
Uses
The dominant application of organoiridium complexes is as catalysts in the Cativa process for carbonylation of methanol to produce acetic acid[9] Iridium is competitive with rhodium for this large-scale application because of lower operating costs.
In the academic laboratories, iridium complexes are widely studied because its complexes promote C-H activation, but such reactions are not employed in any commercial process. Iridium complexes are highly active for hydrogenation both directly and via transfer hydrogenation. The asymmetric versions of these reactions are widely studied.
Iridium complexes such as Ir(mppy)3 have been examined in the context of phosphorescent organic light-emitting diode technology to increase the internal quantum efficiency from 25% to almost 100% by harvesting emission from triplet states.[10]
See also
- Category:Iridium compounds
- Other chemistries of carbon with other elements in the periodic table.
CH | He | ||||||||||||||||
CLi | CBe | CB | CC | CN | CO | CF | Ne | ||||||||||
CNa | CMg | CAl | CSi | CP | CS | CCl | CAr | ||||||||||
CK | CCa | CSc | CTi | CV | CCr | CMn | CFe | CCo | CNi | CCu | CZn | CGa | CGe | CAs | CSe | CBr | CKr |
CRb | CSr | CY | CZr | CNb | CMo | CTc | CRu | CRh | CPd | CAg | CCd | CIn | CSn | CSb | CTe | CI | CXe |
CCs | CBa | CHf | CTa | CW | CRe | COs | CIr | CPt | CAu | CHg | CTl | CPb | CBi | CPo | CAt | Rn | |
Fr | CRa | Rf | Db | Sg | Bh | Hs | Mt | Ds | Rg | Cn | Uut | Fl | Uup | Lv | Uus | Uuo | |
↓ | |||||||||||||||||
CLa | CCe | CPr | CNd | CPm | CSm | CEu | CGd | CTb | CDy | CHo | CEr | CTm | CYb | CLu | |||
Ac | CTh | CPa | CU | CNp | CPu | CAm | CCm | CBk | CCf | CEs | Fm | Md | No | Lr |
Core organic chemistry | Many uses in chemistry |
Academic research, but no widespread use | Bond unknown |
References
- ↑ Greenwood, N. N.; Earnshaw, A. (1997). Chemistry of the Elements (2nd ed.). Oxford:Butterworth-Heinemann. pp. 1113–1143, 1294. ISBN 0-7506-3365-4.
- ↑ Vaska, Lauri; DiLuzio, J.W. (1961). "Carbonyl and Hydrido-Carbonyl Complexes of Iridium by Reaction with Alcohols. Hydrido Complexes by Reaction with Acid". Journal of the American Chemical Society 83 (12): 2784–2785. doi:10.1021/ja01473a054.
- ↑ Crabtree, Robert H. (1979). "Iridium compounds in catalysis". Acc. Chem. Res 12 (9): 331–337. doi:10.1021/ar50141a005.
- ↑ Robert H. Crabtree (2005). The Organometallic Chemistry of the Transition Metals. Wiley. ISBN 978-0-471-66256-3.
- ↑ Carbon–hydrogen activation in saturated hydrocarbons: direct observation of M + R−H → M(R)(H) Andrew H. Janowicz, Robert G. Bergman J. Am. Chem. Soc.; 1982; 104(1); 352–354.doi:10.1021/ja00365a091
- ↑ Oxidative addition of the carbon–hydrogen bonds of neopentane and cyclohexane to a photochemically generated iridium(I) complex James K. Hoyano, William A. G. Graham J. Am. Chem. Soc. 1982; 104(13); 3723–3725. doi:10.1021/ja00377a032
- ↑ Keller, H. J.; Wawersik, H. (1967). "Spektroskopische Untersuchungen an Komplexverbindungen. VI. EPR-spektren von (C5H5)2Rh und (C5H5)2Ir". J. Organomet. Chem. (in German) 8 (1): 185–188. doi:10.1016/S0022-328X(00)84718-X.
- ↑ Fischer, E. O.; Wawersik, H. (1966). "Über Aromatenkomplexe von Metallen. LXXXVIII. Über Monomeres und Dimeres Dicyclopentadienylrhodium und Dicyclopentadienyliridium und Über Ein Neues Verfahren Zur Darstellung Ungeladener Metall-Aromaten-Komplexe". J. Organomet. Chem. (in German) 5 (6): 559–567. doi:10.1016/S0022-328X(00)85160-8.
- ↑ Cheung, Hosea; Tanke, Robin S.; Torrence, G. Paul (2000). "Acetic acid". Ullmann's Encyclopedia of Industrial Chemistry. Wiley. doi:10.1002/14356007.a01_045.
- ↑ Wang, Xiangjun; Andersson, Mats R.; Thompson, Mark E. Inganäsa, Olle (2004). "Electrophosphorescence from substituted poly(thiophene) doped with iridium or platinum complex". Thin Solid Films 468 (1-2): 226–233. Bibcode:2004TSF...468..226W. doi:10.1016/j.tsf.2004.05.095.