Hyperacuity (scientific term)
The sharpness of our senses is defined by the finest detail we can discriminate. Visual acuity is measured by the smallest letters that can be distinguished on a chart and is governed by the anatomical spacing of the mosaic of sensory elements on the retina. Yet spatial distinctions can be made on a finer scale still: misalignment of borders can be detected with a precision up to 10 times better than visual acuity. This hyperacuity, transcending by far the size limits set by the retinal 'pixels', depends on sophisticated information processing in the brain.
How does hyperacuity differ from traditional acuity?
The best example of the distinction between acuity and hyperacuity comes from vision. The first stage is the optical imaging of the outside world on the retina. Light impinges on the mosaic of receptor sense cells, rods and cones, which covers the retinal surface without gaps or overlap, just like the detecting pixels in the film plane of digital cameras. Each receptor accepts all the light reaching it but acts as a unit, representing a single location in visual space. This compartmentalization sets a limit to the decision whether an image came from a single or a double star (resolution). For a percept of separately articulated stars to emerge, the images of the two must be wide enough apart to leave at least one intervening pixel relatively unstimulated between them. This defines the resolution limit and the basis of visual acuity.
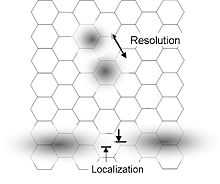
In the figure on the right, the retinal mosaic has superimposed on it, at top, the images of two stars at resolution limit when the intervening gap assures judgment that there are two stars and not a single elongated one. Shown below are the images of two separate short lines; the precision of the read-out of their location difference transcends the dimension of the mosaic elements.
Analysis of Hyperacuity Mechanism
Details of the neural apparatus for hyperacuity still await discovery. That the hyperacuity apparatus involves signals from a range of individual receptor cells, usually in more than one location of the stimulus space, has implications concerning performance in these tasks. Low contrast, close proximity of neighboring stimuli (crowding), temporal asynchrony of pattern components are examples of factors that cause performance deficits.[2] Of some conceptual interest are age changes[3] and susceptibility to perceptual learning[4] which can help in understanding underlying neural channeling.
Hyperacuity in Various Sense Modalities
The distinction between resolving power or acuity, literally sharpness, which depends on the spacing of the individual receptors through which the outside world is sampled, and the ability to identify individual locations in the sensory space is universal among modalities. There are many other examples where the organism’s performance substantially surpasses the spacing of the concerned receptor cell population. The normal human has only three kinds of color receptors in the retina, yet in color vision, by subtly weighing and comparing their relative output, one can detect thousand of hues. Braille reading involves hyperacuity among touch receptors in the fingertips.[5] We can hear many more different tones than there are hair cells in the cochlea; pitch discrimination, without which a violin could not be played in tune, is a hyperacuity.[6] It has been identified in many animal species, for example in the detection of prey by the electric fish,[7] echolocation in the bat,[8] and the mechanical deformation of whiskers in the rodent.[9]
Clinical Applications
In clinical vision tests,[10] hyperacuity has a special place because its processing is at the interfaces of the eye's optics, retinal functions, activation of the primary visual cortex and the perceptual apparatus. In particular, the determination of normal stereopsis is a hyperacuity task. Hyperacuity perimetry is used in clinical trials evaluating therapies for retinal degenerative changes.[11]
References
- ↑ Westheimer, Gerald (1975). "Editorial: Visual acuity and hyperacuity". Investigative Ophthalmology 14 (8): 570–2. PMID 1150397.
- ↑ Westheimer, G (2008). "Hyperacuity". In Squire, L. A. Encyclopedia of Neuroscience. Oxford: Academic Press.
- ↑ Wang, Yi-Zhong; Morale, Sarah E.; Cousins, Robert; Birch, Eileen E. (2009). "Course of Development of Global Hyperacuity over Lifespan". Optometry and Vision Science 86 (6): 695–700. doi:10.1097/OPX.0b013e3181a7b0ff. PMC 2733828. PMID 19430324.
- ↑ Crist, RE; Kapadia, MK; Westheimer, G; Gilbert, CD (1997). "Perceptual learning of spatial localization: Specificity for orientation, position, and context". Journal of neurophysiology 78 (6): 2889–94. PMID 9405509.
- ↑ Loomis, JM (1979). "An investigation of tactile hyperacuity". Sensory processes 3 (4): 289–302. PMID 262782.
- ↑ Altes, Richard A. (1989). "Ubiquity of hyperacuity". The Journal of the Acoustical Society of America 85 (2): 943–52. doi:10.1121/1.397566. PMID 2926010.
- ↑ Heiligenberg, W (1991). "Sensory control of behavior in electric fish". Current Opinion in Neurobiology 1 (4): 633–7. doi:10.1016/S0959-4388(05)80041-8. PMID 1822309.
- ↑ Simmons, J. A.; Ferragamo, M. J.; Sanderson, M. I. (2003). "Echo delay versus spectral cues for temporal hyperacuity in the big brown bat, Eptesicus fuscus". Journal of Comparative Physiology A: Sensory, Neural, and Behavioral Physiology 189 (9): 693–702. doi:10.1007/s00359-003-0444-9.
- ↑ Knutsen, P. M.; Pietr, M; Ahissar, E (2006). "Haptic Object Localization in the Vibrissal System: Behavior and Performance". Journal of Neuroscience 26 (33): 8451–64. doi:10.1523/JNEUROSCI.1516-06.2006. PMID 16914670.
- ↑ Whitaker, David; Buckingham, Terry (1987). "Theory and Evidence for a Clinical Hyperacuity Test". Ophthalmic and Physiological Optics 7 (4): 431–5. doi:10.1111/j.1475-1313.1987.tb00774.x.
- ↑ Goldstein, M; Loewenstein, A; Barak, A; Pollack, A; Bukelman, A; Katz, H; Springer, A; Schachat, AP et al. (2005). "Results of a Multicenter Clinical Trial to Evaluate the Preferential Hyperacuity Perimeter for Detection of Age-Related Macular Degeneration". Retina 25 (3): 296–303. doi:10.1097/00006982-200504000-00008. PMID 15805906.