Graphite intercalation compound
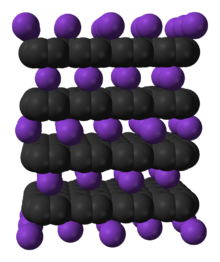
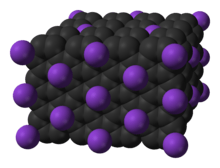
Graphite intercalation compounds (GICs) are complex materials having formula XCy where element or molecule X is inserted (intercalated) between the graphite layers.[1][2][3][4] In this type of compound, the graphite layers remain largely intact and the guest molecules or atoms are located in between. When the host (graphite) and the guest X interact by charge transfer the in-plane electrical conductivity generally increases. This process is largely relied upon in commercial lithium-ion batteries as a reversible charge storage mechanism. When the guest forms covalent bonds with the graphite layers as in fluorides or oxides the conductivity decreases as the conjugated sp2 system collapses.
General structure
In a graphite intercalation compound not every layer is necessarily occupied by guests. In so-called stage 1 compounds, graphite layers and intercalated layers alternate and in stage 2 compounds, two graphite layers with no guest material in between alternate with an intercalated layer. The actual composition may vary and therefore these compounds are an example of non-stoichiometric compounds. It is customary to specify the composition together with the stage.
At the first stage, binary graphite-metal intercalation have stoichiometry XC8 for the large metals (X = K, Rb and Cs) and XC6 for small ones (X = Li, Sr, Ba, Eu, Yb and Ca).[2]
Calcium graphite

Bulk samples of calcium graphite CaC6 are obtained by immersing highly oriented pyrolytic graphite in a well-chosen liquid Li–Ca alloy for long time (10 days at 350 °C). The crystal structure of CaC6 belongs to the R-3m space group. The graphite interlayer distance increases upon Ca intercalation from 3.35 to 4.524 Å, and the carbon-carbon distance increases from 1.42 to 1.444 Å. Among the superconducting graphite intercalation compounds, CaC6 exhibits the highest critical temperature Tc = 11.5 K, which further increases under applied pressure (15.1 K at 8 GPa).[2] Superconductivity in these compounds is thought to be intimately related to the role of an interlayer state, a free electron like band lying roughly 2 eV (0.32 aJ) above the Fermi level; superconductivity only occurs if the interlayer state is occupied.[5]
Potassium graphite
The KC8 form of potassium graphite is one of the strongest reducing agents known. It is prepared under inert atmosphere by melting potassium over graphite powder. The potassium is absorbed into the graphite and its color changes from black to bronze. The resulting solid is also quite pyrophoric, and explodes on contact with water.[6] Structurally, composition is explained by assuming that the potassium to potassium distance is twice the distance between hexagons in the carbon framework. The bond between graphite and potassium atoms is ionic and the compound is more electrically conductive than α-graphite.[6][7] KC8 is a superconductor with a very low critical temperature Tc = 0.14 K.[2] It has also been used as a catalyst in polymerizations and as a coupling reagent for aryl halides to biphenyls.[8] In one study, freshly prepared KC8 was treated with 1-iodododecane delivering a modification (micrometre scale carbon platelets with long alkyl chains sticking out providing solubility) that is soluble in chloroform.[8] Another potassium graphite compound, KC24, has been used as a neutron monochromator. A new essential application for potassium graphite was introduced by the invention of the potassium-ion battery. Like the lithium-ion battery, the potassium-ion battery should use a carbon-based anode instead of a metallic anode. In this circumstance, the stable structure of potassium graphite is an important advantage.
Heating KC8 leads to the formation of a series of decomposition products as the K atoms are eliminated, ultimately giving the blue compound KC60:
- KC8 → KC24 → KC36 → KC48 → KC60
Carbon fluorides
Carbon monofluoride is denoted as (CF)x and used as a cathode material in one type of primary (non-rechargeable) lithium batteries. It is prepared by reaction of gaseous fluorine with graphitic carbon at 215–230 °C. The color is greyish, white, or yellow. The bond between the carbon and fluorine atoms is covalent. The compound is not electrically conductive which makes the addition of conductive fillers necessary for battery use.
Tetracarbon monofluoride (C4F) is prepared by reacting gaseous fluorine mixed with hydrogen fluoride with graphite at room temperature. The compound has a blackish-blue color.
Graphite bisulfate and graphite perchlorate
The intercalation compounds graphite bisulfate and graphite perchlorate can be made by reacting graphite with strong acids in the presence of oxidizing agents, causing the graphite to lose electrons and become positively charged.
Graphite bisulfate, [C24]+[HSO4]-, is made by reacting graphite with concentrated sulfuric acid and a little nitric acid or chromic acid. The analogous graphite perchlorate can be made similarly by reaction with perchloric acid.
In graphite perchlorate, planar layers of carbon atoms are 794 picometers apart, separated by ClO4- ions. Cathodic reduction of graphite perchlorate is analogous to heating KC8, which leads to a sequential elimination of HClO4.
Both graphite bisulfate and graphite perchlorate are better conductors as compared to graphite, as predicted by using a positive-hole mechanism.[6]
Others
Other graphite intercalation compounds includes graphite oxide. Examples of complex graphite intercalation compounds are those with barium and ammonia guests (Ba(NH3)2.5C10.9(stage 1)) or those with caesium, hydrogen and potassium (CsC8·K2H4/3C8(stage 1)).
Intercalation compounds are formed when oxidized by chlorine, bromine, iodine monochloride, krypton difluoride, uranium hexafluoride and iron trichloride. Reaction of graphite with [O2]+[AsF6]- affords the salt [C8]+[AsF6]-.[6]
See also
- Covalent superconductors
- Graphite
- Magnesium diboride, which uses hexagonal planar boron sheets instead of carbon
- Pyrolytic graphite
References
- ↑ M.S. Dresselhaus and G. Dresselhaus Review: (1981). "Intercalation compounds of graphite". Advances in Physics 30 (2): 139. Bibcode:1981AdPhy..30..139D. doi:10.1080/00018738100101367. (187 pages), also reprinted as Dresselhaus, M. S.; Dresselhaus, G. (2002). "Intercalation compounds of graphite". Advances in Physics 51: 1. Bibcode:2002AdPhy..51....1D. doi:10.1080/00018730110113644.
- ↑ 2.0 2.1 2.2 2.3 N. Emery et al. (2008). "Review: Synthesis and superconducting properties of CaC6". Sci. Technol. Adv. Mater. (PDF) 9 (4): 044102. Bibcode:2008STAdM...9d4102E. doi:10.1088/1468-6996/9/4/044102.
- ↑ H-P Boehm et al. (1994). "Nomenclature and terminology of graphite intercalation compounds". Pure & Appl. Chem. (PDF) 66 (9): 1893. doi:10.1351/pac199466091893.
- ↑ D. Savoia et al. (1985). "Applications of potassium-graphite and metals dispersed on graphite in organic synthesis". Pure & Appl. Chem. (PDF) 57 (12): 1887. doi:10.1351/pac198557121887.
- ↑ Csányi et al. (2005). "The role of the interlayer state in the electronic structure of superconducting graphite intercalated compounds". Nature Physics 1: 42–45. Bibcode:2005NatPh...1...42C. doi:10.1038/nphys119.
- ↑ 6.0 6.1 6.2 6.3 Catherine E. Housecroft; Alan G. Sharpe (2008). "Chapter 14: The group 14 elements". Inorganic Chemistry, 3rd Edition. Pearson. p. 386. ISBN 978-0-13-175553-6.
- ↑ NIST Ionizing Radiation Division 2001 - Technical Highlights
- ↑ 8.0 8.1 S. Chakraborty et al. (2007). "Functionalization of Potassium Graphite". Angew. Chem. Int. Ed. 46 (24): 4486–8. doi:10.1002/anie.200605175. PMID 17477336.
Further reading
- T. Enoki, M. Suzuki and M. Endo (2003). Graphite intercalation compounds and applications. Oxford University Press. ISBN 0-19-512827-3.
External links
![]() |
Wikimedia Commons has media related to Graphite intercalation compounds. |