Cephalopod
Cephalopods Temporal range: Late Cambrian – Present[1] | |||||||
---|---|---|---|---|---|---|---|
![]() | |||||||
Bigfin reef squid (Sepioteuthis lessoniana) | |||||||
Scientific classification ![]() | |||||||
Kingdom: | Animalia | ||||||
Phylum: | Mollusca | ||||||
Class: | Cephalopoda Cuvier, 1797 | ||||||
Subclasses and orders | |||||||
| |||||||
A cephalopod is any member of the molluscan class Cephalopoda (Greek plural κεφαλόποδα (kephalópoda); "head-feet"). These exclusively marine animals are characterized by bilateral body symmetry, a prominent head, and a set of arms or tentacles (muscular hydrostats) modified from the primitive molluscan foot. Fishermen sometimes call them inkfish, referring to their common ability to squirt ink. The study of cephalopods is a branch of malacology known as teuthology.[2]
Cephalopods became dominant during the Ordovician period, represented by primitive nautiloids. The class now contains two, only distantly related, extant subclasses: Coleoidea, which includes octopuses, squid, and cuttlefish; and Nautiloidea, represented by Nautilus and Allonautilus. In the Coleoidea, the molluscan shell has been internalized or is absent, whereas in the Nautiloidea, the external shell remains. About 800 living species of cephalopods have been identified. Two important extinct taxa are the Ammonoidea (ammonites) and Belemnoidea (belemnites).
Distribution


Right: Benthoctopus sp. on the Davidson Seamount at 2,422 m depth
There are around 800 extant species of cephalopod,[3] although new species continue to be described. An estimated 11,000 extinct taxa have been described, although the soft-bodied nature of cephalopods means they are not easily fossilised.[4]
Cephalopods are found in all the oceans of Earth. None of them can tolerate freshwater, but the brief squid, Lolliguncula brevis, found in Chesapeake Bay, may be a notable exception in that it tolerates brackish water.[5]
Cephalopods occupy most of the depth of the ocean, from the abyssal plain to the sea surface. Their diversity is greatest near the equator (~40 species retrieved in nets at 11°N by a diversity study) and decreases towards the poles (~5 species captured at 60°N).[6]
Nervous system and behavior

_behavior_.jpg)
Right: Hawaiian bobtail squid, Euprymna scolopes, burying itself in the sand, leaving only the eyes exposed
Cephalopods are widely regarded as the most intelligent of the invertebrates, and have well developed senses and large brains (larger than those of gastropods). The nervous system of cephalopods is the most complex of the invertebrates[7] and their brain-to-body-mass ratio falls between that of endothermic and ectothermic vertebrates.[6]:14 The brain is protected in a cartilaginous cranium. The giant nerve fibers of the cephalopod mantle have been widely used for many years as experimental material in neurophysiology; their large diameter (due to lack of myelination) makes them relatively easy to study compared with other animals.[8]
Cephalopods are social creatures; when isolated from their own kind, they will sometimes shoal with fish.[9]
Some cephalopods are able to "fly" through the air for distances of up to 50 m. While cephalopods are not particularly aerodynamic, they achieve these impressive ranges by jet-propulsion; water continues to be expelled from the funnel while the organism is in the air.[10]
Senses
Cephalopods have advanced vision, can detect gravity with statocysts, and have a variety of chemical sense organs.[6]:34 Octopuses use their tentacles to explore their environment and can use them for depth perception.[6]
Vision
.jpg)
Most cephalopods rely on vision to detect predators and prey, and to communicate with one another.[11] Consequently, cephalopod vision is acute: training experiments have shown that the common octopus can distinguish the brightness, size, shape, and horizontal or vertical orientation of objects. The morphological construction gives cephalopod eyes the same performance as sharks'; however, their construction differs, as cephalopods lack a cornea, and have an everted retina.[11] Cephalopods' eyes are also sensitive to the plane of polarization of light.[12] Surprisingly—given their ability to change color—all octopuses[13] and most cephalopods[14] are color blind. When camouflaging themselves, they use their chromatophores to change brightness and pattern according to the background they see, but their ability to match the specific color of a background may come from cells such as iridophores and leucophores that reflect light from the environment.[15] They also produce visual pigments throughout their body, and may sense light levels directly from their body.[16] Evidence of color vision has been found in the sparkling enope squid (Watasenia scintillans),[14][17] which achieves color vision by the use of three distinct retinal molecules (A1, sensitive to red; A2, to purple, and A4, to yellow?) which bind to its opsin.[18]
Unlike many other cephalopods, nautiluses do not have good vision; their eye structure is highly developed, but lacks a solid lens. They have a simple "pinhole" eye through which water can pass. Instead of vision, the animal is thought to use olfaction as the primary sense for foraging, as well as locating or identifying potential mates.
Hearing
Some squids have been shown to detect sound using their statocysts.[19]
Use of light

Most cephalopods possess chromatophores - that is, coloured pigments - which they can use in a startling array of fashions.[6] As well as providing camouflage with their background, some cephalopods bioluminesce, shining light downwards to disguise their shadows from any predators that may lurk below.[6] The bioluminescence is produced by bacterial symbionts; the host cephalopod is able to detect the light produced by these organisms.[20] Bioluminescence may also be used to entice prey, and some species use colourful displays to impress mates, startle predators, or even communicate with one another.[6] It is not certain whether bioluminescence is actually of epithelial origin or if it is a bacterial production.[21]
Coloration
Cephalopods can change their colours and patterns in milliseconds, whether for signalling (both within the species and for warning) or active camouflage,[6] as their chromatophores are expanded or contracted.[21] Coloration is typically stronger in near-shore species than those living in the open ocean, whose functions tend to be restricted to disruptive camouflage.[6]:2
Evidence of original coloration has been detected in cephalopod fossils dating as far back as the Silurian; these orthoconic individuals bore concentric stripes, which are thought to have served as camouflage.[22] Devonian cephalopods bear more complex colour patterns, of unknown function.[23]
Ink
With the exception of the Nautilidae and the species of octopus belonging to the suborder Cirrina,[24] all known cephalopods have an ink sac, which can be used to expel a cloud of dark ink to confuse predators.[13] This sac is a muscular bag which originated as an extension of the hind gut. It lies beneath the gut and opens into the anus, into which its contents – almost pure melanin – can be squirted; its proximity to the base of the funnel means the ink can be distributed by ejected water as the cephalopod uses its jet propulsion.[13] The ejected cloud of melanin is usually mixed, upon expulsion, with mucus, produced elsewhere in the mantle, and therefore forms a thick cloud, resulting in visual (and possibly chemosensory) impairment of the predator, like a smokescreen. However, a more sophisticated behaviour has been observed, in which the cephalopod releases a cloud, with a greater mucus content, that approximately resembles the cephalopod that released it (this decoy is referred to as a Pseudomorph). This strategy often results in the predator attacking the pseudomorph, rather than its rapidly departing prey.[13] For more information, see Inking behaviors.
The inking behaviour of cephalopods has led to a common name of "inkfish", primarily used in fisheries science and the fishing industry, paralleling the terms white fish, oily fish, and shellfish.[citation needed]


Circulatory system
Cephalopods are the only mollusks with a closed circulatory system. Coleoids have two gill hearts (also known as branchial hearts) that move blood through the capillaries of the gills. A single systemic heart then pumps the oxygenated blood through the rest of the body.[25]
Like most molluscs, cephalopods use hemocyanin, a copper-containing protein, rather than hemoglobin, to transport oxygen. As a result, their blood is colorless when deoxygenated and turns blue when exposed to air.[26]
Respiration
Cephalopods exchange gases with the seawater by forcing water through their gills, which are attached to the roof of the organism.[27]:488[28] Water enters the mantle cavity on the outside of the gills, and the entrance of the mantle cavity closes. When the mantle contracts, water is forced through the gills, which lie between the mantle cavity and the funnel. The water's expulsion through the funnel can be used to power jet propulsion. The gills, which are much more efficient than those of other molluscs, are attached to the ventral surface of the mantle cavity.[28] There is a trade-off with gill size regarding lifestyle. To achieve fast speeds, gills need to be small—water will be passed through them quickly when energy is needed, compensating for their small size. However, organisms which spend most of their time moving slowly along the bottom do not naturally pass much water through their cavity for locomotion; thus they have larger gills, along with complex systems to ensure that water is constantly washing through their gills, even when the organism is stationary.[27] The water flow is controlled by contractions of the radial and circular mantle cavity muscles.[29]
The gills of cephalopods are supported by a skeleton of robust fibrous proteins; the lack of mucopolysaccharides distinguishes this matrix from cartilage.[30][31] The gills are also thought to be involved in excretion, with NH4+ being swapped with K+ from the seawater.[28]
Locomotion and buoyancy

While most cephalopods can move by jet propulsion, this is a very energy-consuming way to travel compared to the tail propulsion used by fish.[32] The efficiency of a propellor-driven waterjet (i.e. Froude efficiency) is a more efficient model than rocket efficiency.[33] The relative efficiency of jet propulsion decreases further as animal size increases; paralarvae are far more efficient than juvenile and adult individuals.[34] Since the Paleozoic era, as competition with fish produced an environment where efficient motion was crucial to survival, jet propulsion has taken a back role, with fins and tentacles used to maintain a steady velocity.[4] Whilst jet propulsion is never the sole mode of locomotion,[4]:208 the stop-start motion provided by the jets continues to be useful for providing bursts of high speed - not least when capturing prey or avoiding predators.[4] Indeed, it makes cephalopods the fastest marine invertebrates,[6]:Preface and they can out-accelerate most fish.[27] The jet is supplemented with fin motion; in the squid, the fins flap each time that a jet is released, amplifying the thrust; they are then extended between jets (presumably to avoid sinking).[34] Oxygenated water is taken into the mantle cavity to the gills and through muscular contraction of this cavity, the spent water is expelled through the hyponome, created by a fold in the mantle. The size difference between the posterior and anterior ends of this organ control the speed of the jet the organism can produce.[35] The velocity of the organism can be accurately predicted for a given mass and morphology of animal.[36] Motion of the cephalopods is usually backward as water is forced out anteriorly through the hyponome, but direction can be controlled somewhat by pointing it in different directions.[37] Some cephalopods accompany this expulsion of water with a gunshot-like popping noise, thought to function to frighten away potential predators.[38]
Cephalopods employ a similar method of propulsion despite their increasing size (as they grow) changing the dynamics of the water in which they find themselves. Thus their paralarvae do not extensively use their fins (which are less efficient at low Reynolds numbers) and primarily use their jets to propel themselves upwards, whereas large adult cephalopods tend to swim less efficiently and with more reliance on their fins.[34]

Early cephalopods are thought to have produced jets by drawing their body into their shells, as Nautilus does today.[39] Nautilus is also capable of creating a jet by undulations of its funnel; this slower flow of water is more suited to the extraction of oxygen from the water.[39] The jet velocity in Nautilus is much slower than in coleoids, but less musculature and energy is involved in its production.[40] Jet thrust in cephalopods is controlled primarily by the maximum diameter of the funnel orifice (or, perhaps, the average diameter of the funnel)[41]:440 and the diameter of the mantle cavity.[42] Changes in the size of the orifice are used most at intermediate velocities.[41] The absolute velocity achieved is limited by the cephalopod's requirement to inhale water for expulsion; this intake limits the maximum velocity to eight body-lengths per second, a speed which most cephalopods can attain after two funnel-blows.[41] Water refills the cavity by entering not only through the orifices, but also though the funnel.[41] Squid can expel up to 94% of the fluid within their cavity in a single jet thrust.[33] To accommodate the rapid changes in water intake and expulsion, the orifices are highly flexible and can change their size by a factor of twenty; the funnel radius, conversely, changes only by a factor of around 1.5.[41]
Some octopus species are also able to walk along the sea bed. Squids and cuttlefish can move short distances in any direction by rippling of a flap of muscle around the mantle.
While most cephalopods float (i.e. are neutrally buoyant or nearly so; in fact most cephalopods are about 2-3% denser than seawater[9]), they achieve this in different ways.[32] Some, such as Nautilus, allow gas to diffuse into the gap between the mantle and the shell; others allow purer water to ooze from their kidneys, forcing out denser salt water from the body cavity;[32] others, like some fish, accumulate oils in the liver;[32] and some octopuses have a gelatinous body with lighter chlorine ions replacing sulfate in the body chemistry.[32]
Shell


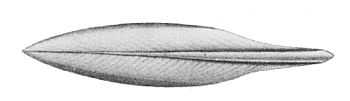
Nautiluses are the only extant cephalopods with a true external shell. However, all molluscan shells are formed from the ectoderm (outer layer of the embryo); in cuttlefish (Sepia spp.), for example, an invagination of the ectoderm forms during the embryonic period, resulting in a shell (cuttlebone) that is internal in the adult.[43] The same is true of the chitinous gladius of squid[43] and octopuses.[44] Cirrate octopods have arch-shaped cartilaginous fin supports,[45] which are sometimes referred to as a "shell vestige" or "gladius".[46] The Incirrina have either a pair of rod-shaped stylets or no vestige of an internal shell,[47] and some squid also lack a gladius.[48] Interestingly, the shelled coleoids do not form a clade or even a paraphyletic group.[49] The Spirula shell begins as an organic structure, and is then very rapidly mineralized.[50] Shells that are "lost" may be lost by resorption of the calcium carbonate component.[51]
Females of the octopus genus Argonauta secrete a specialised paper-thin eggcase in which they reside, and this is popularly regarded as a "shell", although it is not attached to the body of the animal.
The largest group of shelled cephalopods, the ammonites, are extinct, but their shells are very common as fossils.
The deposition of carbonate, leading to a mineralized shell, appears to be related to the acidity of the organic shell matrix (see Mollusc shell); shell-forming cephalopods have an acidic matrix, whereas the gladius of squid has a basic matrix.[52]


Right: Detail of the tentacular club of Abraliopsis morisi
Head appendages
Cephalopods, as the name implies, have muscular appendages extending from their heads and surrounding their mouths. These are used in feeding, mobility, and even reproduction. In coleoids they number eight or ten. Decapods such as cuttlefish and squid have five pairs. The longer two, termed tentacles, are actively involved in capturing prey;[1]:225 they can lengthen rapidly (in as little as 15 milliseconds[1]:225). In giant squid they may reach a length of 8 metres. They may terminate in a broadened, sucker-coated club.[1]:225 The shorter four pairs are termed arms, and are involved in holding and manipulating the captured organism.[1]:225 They too have suckers, on the side closest to the mouth; these help to hold onto the prey.[1]:226 Octopods only have four pairs of sucker-coated arms, as the name suggests, though developmental abnormalities can modify the number of arms expressed.[53]
The tentacle consists of a thick central nerve cord (which must be thick to allow each sucker to be controlled independently)[54] surrounded by circular and radial muscles. Because the volume of the tentacle remains constant, contracting the circular muscles decreases the radius and permits the rapid increase in length. Typically a 70% lengthening is achieved by decreasing the width by 23%.[1]:227 The shorter arms lack this capability.
The size of the tentacle is related to the size of the buccal cavity; larger, stronger tentacles can hold prey as small bites are taken from it; with more numerous, smaller tentacles, prey is swallowed whole, so the mouth cavity must be larger.[55]
Externally shelled nautilids (Nautilus and Allonautilus) have on the order of 90 finger-like appendages, termed tentacles, which lack suckers but are sticky instead, and are partly retractable.
Feeding

All living cephalopods have a two-part beak;[6]:7 most have a radula, although it is reduced in most octopus and absent altogether in Spirula.[6]:7[56]:110 They feed by capturing prey with their tentacles, drawing it into their mouth and taking bites from it.[13] They have a mixture of toxic digestive juices, some of which are manufactured by symbiotic algae, which they eject from their salivary glands onto their captured prey held in their mouth. These juices separate the flesh of their prey from the bone or shell.[13] The salivary gland has a small tooth at its end which can be poked into an organism to digest it from within.[13]
The digestive gland itself is rather short.[13] It has four elements, with food passing through the crop, stomach and caecum before entering the intestine. Most digestion, as well as the absorption of nutrients, occurs in the digestive gland, sometimes called the liver. Nutrients and waste materials are exchanged between the gut and the digestive gland through a pair of connections linking the gland to the junction of the stomach and caecum.[13] Cells in the digestive gland directly release pigmented excretory chemicals into the lumen of the gut, which are then bound with mucus passed through the anus as long dark strings, ejected with the aid of exhaled water from the funnel.[13] Cephalopods tend to concentrate ingested heavy metals in their body tissue.[57]
Radula

The cephalopod radula consists of multiple symmetrical rows of up to nine teeth[58] – thirteen in fossil classes.[59] The organ is reduced or even vestigial in certain octopus species and is absent in Spirula.[59] The teeth may be homodont (i.e. similar in form across a row), heterodont (otherwise), or ctenodont (comb-like).[59] Their height, width and number of cusps is variable between species.[59] The pattern of teeth repeats, but each row may not be identical to the last; in the octopus, for instance, the sequence repeats every five rows.[59]:79
Cephalopod radulae are known from fossil deposits dating back to the Ordovician.[60] They are usually preserved within the cephalopod's body chamber, commonly in conjunction with the mandibles; but this need not always be the case;[61] many radulae are preserved in a range of settings in the Mason Creek.[62] Radulae are usually difficult to detect, even when they are preserved in fossils, as the rock must weather and crack in exactly the right fashion to expose them; for instance, radulae have only been found in nine of the 43 ammonite genera,[63] and they are rarer still in non-ammonoid forms: only three pre-Mesozoic species possess one.[60]
Excretory system
Most cephalopods possess a single pair of large nephridia. Filtered nitrogenous waste is produced in the pericardial cavity of the branchial hearts, each of which is connected to a nephridium by a narrow canal. The canal delivers the excreta to a bladder-like renal sac, and also resorbs excess water from the filtrate. Several outgrowths of the lateral vena cava project into the renal sac, continuously inflating and deflating as the branchial hearts beat. This action helps to pump the secreted waste into the sacs, to be released into the mantle cavity through a pore.[64]
Nautilus, unusually, possesses four nephridia, none of which are connected to the pericardial cavities.
The incorporation of ammonia is important for shell formation in terrestrial molluscs and other non-molluscan lineages.[65] Because protein (i.e. flesh) is a major constituent of the cephalopod diet, large amounts of ammonium are produced as waste. The main organs involved with the release of this excess ammonium are the gills.[66] The rate of release is lowest in the shelled cephalopods Nautilus and Sepia as a result of their using nitrogen to fill their shells with gas to increase buoyancy.[66] Other cephalopods use ammonium in a similar way, storing the ions (as ammonium chloride) to reduce their overall density and increase buoyancy.[66]
Reproduction and life cycle
![]() Female Argonauta argo with eggcase and eggs ![]() Detail of the hectocotylus of Ocythoe tuberculata |
![]() A dissected male specimen of Onykia ingens, showing a non-erect penis (the white tubular structure located below most of the other organs) ![]() A specimen of the same species exhibiting elongation of the penis to 67 cm in length |
With a few exceptions, Coleoidea live short lives with rapid growth. Most of the energy extracted from their food is used for growing. The penis in most male Coleoidea is a long and muscular end of the gonoduct used to transfer spermatophores to a modified arm called a hectocotylus. That, in turn, is used to transfer the spermatophores to the female. In species where the hectocotylus is missing, the penis is long and able to extend beyond the mantle cavity and transfers the spermatophores directly to the female. Deep water squid have the greatest known penis length relative to body size of all mobile animals, second in the entire animal kingdom only to certain sessile barnacles.[67] Penis elongation in Onykia ingens may result in a penis that is as long as the mantle, head and arms combined.[67][68]
Most cephalopods tend towards a semelparous reproduction strategy; they lay many small eggs in one batch and die afterwards. The Nautiloidea, on the other hand, stick to iteroparity; they produce a few large eggs in each batch and live for a long time.[citation needed]
External sexual characteristics are lacking in cephalopods, so cephalopods use colour communication. A courting male will approach a likely looking opposite number flashing his brightest colours, often in rippling displays. If the other cephalopod is female and receptive, her skin will change colour to become pale, and mating will occur. If the other cephalopod remains brightly coloured, it is taken as a warning.[69]
The male has a sperm-carrying arm, known as the hectocotylous arm, with which to impregnate the female. In many cephalopods, mating occurs head to head and the male may simply transfer sperm to the female. Others may detach the sperm-carrying arm and leave it attached to the female. In the paper nautilus, this arm remains active and wriggling for some time, prompting the zoologists who discovered it to conclude it was some sort of worm-like parasite. It was duly given a genus name Hectocotylus, which held for some time until the mistake was discovered.[69]:227
Nidamental glands are involved in the secretion of egg cases or the gelatinous substance comprising egg masses.[70] The eggs may be brooded: female paper nautilus construct a shelter for the young, while Gonatiid squid carry a larva-laden membrane from the hooks on their arms.[71] Other cephalopods deposit their young under rocks and aerate them with their tentacles hatching. Often, though, the eggs are left to their own devices; many squid lay sausage-like bunches of eggs in crevices or occasionally on the sea floor. Cuttlefish lay their eggs separately in cases and attach them to coral or algal fronds.[72] Fossilised egg clutches show that ammonites also laid clutches of eggs.[73]
Cephalopods are occasionally long-lived, especially in the deep water or polar forms, but most of the group live fast and die young, maturing rapidly to their adult size. Some may gain as much as 12% of their body mass each day.[13] Most live for one to two years,[13] reproducing and then dying shortly thereafter.[74]
To free up resources for reproduction, many squid are known to resorb the muscle tissue of their mantle and tentacles, breaking down the tissue and using the energy contained therein to produce more gametes.[75]

Embryology
Cephalopod eggs span a large range of sizes, from 1 to 30 mm in diameter.[78] The fertilised ovum initially divides to produce a disc of germinal cells at one pole, with the yolk remaining at the opposite pole. The germinal disc grows to envelop and eventually absorb the yolk, forming the embryo. The tentacles and arms first appear at the hind part of the body, where the foot would be in other molluscs, and only later migrate towards the head.[64][76]
The funnel of cephalopods develops on the top of their head, whereas the mouth develops on the opposite surface.[77]:86 The early embryological stages are reminiscent of ancestral gastropods and extant Monoplacophora.[76]
The shells develop from the ectoderm as an organic framework which is subsequently mineralised.[43] In Sepia, which has an internal shell, the ectoderm forms an invagination whose pore is sealed off before this organic framework is deposited.[43]
Development

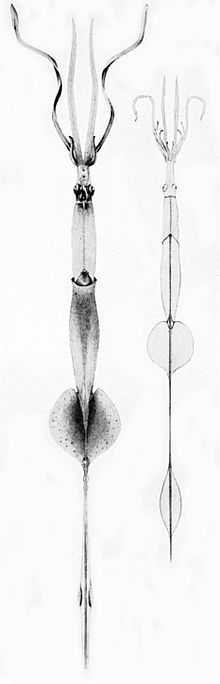
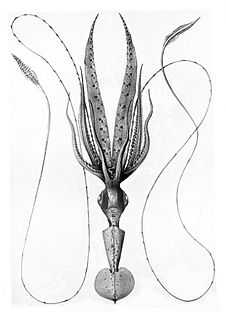
Right: A mature Chiroteuthis veranyi. This species has some of the longest tentacles in proportion to its size of any known cephalopod.
The length of time before hatching is highly variable; smaller eggs in warmer waters are the fastest to hatch, and newborns can emerge after as little as a few days. Larger eggs in colder waters can develop for over a year before hatching.[78]
The process from spawning to hatching follows a similar trajectory in all species, the main variable being the amount of yolk available to the young and when it is absorbed by the embryo.[78]
Unlike most other molluscs, cephalopods do not have a morphologically distinct larval stage. Instead the juveniles are known as paralarvae. They quickly learn how to hunt, using encounters with prey to refine their strategies
Growth in juveniles is usually allometric, whilst adult growth is isometric.[79]
Evolution
The traditional view of cephalopod evolution holds that they evolved in the Late Cambrian from a monoplacophoran-like ancestor[80] with a curved, tapering shell,[81] which was closely related to the gastropods (snails).[82] The similarity of the early shelled cephalopod Plectronoceras to some gastropods was used in support of this view. The development of a siphuncle would have allowed the shells of these early forms to become gas-filled (thus buoyant) in order to support them and keep the shells upright while the animal crawled along the floor, and separated the true cephalopods from putative ancestors such as Knightoconus, which lacked a siphuncle.[82] Neutral or positive buoyancy (i.e. the ability to float) would have come later, followed by swimming in the Plectronocerida and eventually jet propulsion in more derived cephalopods.[83]
However, some morphological evidence is difficult to reconcile with this view, and the redescription of Nectocaris pteryx, which did not have a shell and appeared to possess jet propulsion in the manner of "derived" cephalopods, complicated the question of the order in which cephalopod features developed – provided Nectocaris is a cephalopod at all.[84] Their position within the Mollusca is currently wide open to interpretation - see Mollusca#Phylogeny.
Early cephalopods were likely predators near the top of the food chain.[13] They underwent pulses of diversification during the Ordovician period[85] to become diverse and dominant in the Paleozoic and Mesozoic seas.[86] In the Early Palaeozoic, their range was far more restricted than today; they were mainly constrained to sublittoral regions of shallow shelves of the low latitudes, and usually occur in association with thrombolites.[87] A more pelagic habit was gradually adopted as the Ordovician progressed.[87] Deep-water cephalopods, whilst rare, have been found in the Lower Ordovician - but only in high-latitude waters.[87] The mid Ordovician saw the first cephalopods with septa strong enough to cope with the pressures associated with deeper water, and could inhabit depths greater than 100–200 m.[85] The direction of shell coiling would prove to be crucial to the future success of the lineages; endogastric coiling would only permit large size to be attained with a straight shell, whereas exogastric coiling - initially rather rare - permitted the spirals familiar from the fossil record to develop, with their corresponding large size and diversity.[88] (Endogastric mean the shell is curved so as the ventral or lower side is longitudinally concave (belly in); exogastric means the shell is curved so as the ventral side is longitudinally convex (belly out) allowing the funnel to be pointed backwards beneath the shell.)[88]

The ancestors of coleoids (including most modern cephalopods) and the ancestors of the modern nautilus, had diverged by the Floian Age of the Early Ordovician Period, over 470 million years ago.[87][89] The Bactritida, a Silurian–Triassic group of orthocones, are widely held to be paraphyletic to the coleoids and ammonoids – that is, the latter groups arose from within the Bactritida.[90]:393 An increase in the diversity of the coleoids and ammonoids is observed around the start of the Devonian period, and corresponds with a profound increase in fish diversity. This could represent the origin of the two derived groups.[90]
Unlike most modern cephalopods, most ancient varieties had protective shells. These shells at first were conical but later developed into curved nautiloid shapes seen in modern nautilus species. Competitive pressure from fish is thought to have forced the shelled forms into deeper water, which provided an evolutionary pressure towards shell loss and gave rise to the modern coleoids, a change which led to greater metabolic costs associated with the loss of buoyancy, but which allowed them to recolonise shallow waters.[82]:36 However, some of the straight-shelled nautiloids evolved into belemnites, out of which some evolved into squid and cuttlefish. The loss of the shell may also have resulted from evolutionary pressure to increase manoeuvrability, resulting in a more fish-like habit.[1]:289
Phylogeny
Cephalopod phylogeny | |||||||||||||||||||||||||||||||||||||||||||||||||||
| |||||||||||||||||||||||||||||||||||||||||||||||||||
Approximate consensus of extant cephalopod phylogeny, after Strugnell et al. 2007[49] Mineralized taxa are in bold. The attachment of the clade including Sepia and Spirula is unclear; either of the points marked with an asterisk may represent the root of this clade. |
The internal phylogeny of the cephalopods is difficult to constrain; many molecular techniques have been adopted, but the results produced are conflicting.[49][91] Nautilus tends to be considered an outgroup, with Vampyroteuthis forming an outgroup to other squid; however in one analysis the nautiloids, octopus and teuthids plot as a polytomy.[49] Some molecular phylogenies do not recover the mineralized coleoids (Spirula, Sepia, and Metasepia) as a clade; however, others do recover this more parsimonious-seeming clade, with Spirula as a sister group to Sepia and Metasepia in a clade that had probably diverged before the end of the Triassic.[92][93]
Molecular estimates for clade divergence vary. One 'statistically robust' estimate has Nautilus diverging from Octopus at 415 ± 24 million years ago.[94]
Taxonomy

.jpg)



The classification presented here, for recent cephalopods, follows largely from Current Classification of Recent Cephalopoda (May 2001), for fossil cephalopods takes from Arkell et al. 1957, Teichert and Moore 1964, Teichert 1988, and others. The three subclasses are traditional, corresponding to the three orders of cephalopods recognized by Bather.[95]
Class Cephalopoda († indicates extinct groups)
- Subclass Nautiloidea: Fundamental ectocochliate cephalopods that provided the source for the Ammonoidea and Coleoidea.
- Order † Plectronocerida: the ancestral cephalopods from the Cambrian Period
- Order † Ellesmerocerida (500 to 470 Ma)
- Order † Endocerida (485 to 430 Ma)
- Order † Actinocerida (480 to 312 Ma)
- Order † Discosorida (482 to 392 Ma)
- Order † Pseudorthocerida (432 to 272 Ma)
- Order † Tarphycerida (485 to 386 Ma)
- Order † Oncocerida (478.5 to 324 Ma)
- Order Nautilida (extant; 410.5 to 0 Ma)
- Order † Orthocerida (482.5 to 211.5 Ma)
- Order † Ascocerida (478 to 412 Ma)
- Order † Bactritida (418.1 to 260.5 Ma)
- Subclass † Ammonoidea: Ammonites (479 to 66 Ma)
- Order † Goniatitida (388.5 to 252 Ma)
- Order † Ceratitida (254 to 200 Ma)
- Order † Ammonitida (215 to 66 Ma)
- Subclass Coleoidea (410.0 Ma-Rec)
- Cohort † Belemnoidea: Belemnites and kin
- Genus † Jeletzkya
- Order † Aulacocerida (265 to 183 Ma)
- Order † Phragmoteuthida (189.6 to 183 Ma)
- Order † Hematitida (339.4 to 318.1 Ma)
- Order † Belemnitida (339.4 to 66 Ma)
- Genus † Belemnoteuthis (189.6 to 183 Ma)
- Cohort Neocoleoidea
- Superorder Decapodiformes (also known as Decabrachia or Decembranchiata)
- ?Order † Boletzkyida
- Order Spirulida: Ram's horn squid
- Order Sepiida: cuttlefish
- Order Sepiolida: pygmy, bobtail and bottletail squid
- Order Teuthida: squid
- Superorder Octopodiformes (also known as Vampyropoda)
- Family † Trachyteuthididae
- Order Vampyromorphida: Vampire squid
- Order Octopoda: octopus
- Superorder Decapodiformes (also known as Decabrachia or Decembranchiata)
- Cohort † Belemnoidea: Belemnites and kin
Other classifications differ, primarily in how the various decapod orders are related, and whether they should be orders or families.
Suprafamilial classification of the Treatise
This is the older classification that combines those found in parts K and L of the Treatise on Invertebrate Paleontology, which forms the basis for and is retained in large part by classifications that have come later.
Nautiloids in general, (Teichert and Moore 1964) Sequence as given.
- Subclass † Endoceratoidea. Not used by Flower, e.g. Flower and Kummel 1950, interjocerids included in the Endocerida.
- Order † Endocerida
- Order † Intejocerida
- Subclass † Actinoceratoidea Not used by Flower, ibid
- Order † Actinocerida
- Subclass † Nautiloidea Nautiloidea in the restricted sense.
- Order † Ellesmerocerida Plectronocerida subsequently split off as separate order.
- Order † Orthocerida Includes orthocerids and pseudorthocerids
- Order † Ascocerida
- Order † Oncocerida
- Order † Discosorida
- Order † Tarphycerida
- Order † Barrandeocerida A polyphyletic group now included in the Tarphycerida
- Order Nautilida
- Subclass † Bactritoidea
- Order † Bactritida
Paleozoic Ammonoidea ( Miller, Furnish, and Schindewolf, 1957)
- Suborder † Anarcestina
- Suborder † Clymeniina
- Suborder † Goniatitina
- Suborder † Prolecanitina
Mesozoic Ammonoidea (Arkel et al., 1957)
- Suborder † Ceratitina
- Suborder † Phylloceratina
- Suborder † Lytoceratina
- Suborder † Ammonitina
Subsequent revisions include the establishment of three Upper Cambrian orders, the Plectronocerida, Protactinocerida and Yanhecerida; separation of the pseudorthocerids as the Pseudorthocerida, and elevating orthoceritoids as the Subclass Orthoceratoidea.
Shevyrev classification
Shevyrev (2005) suggested a division into eight subclasses, mostly comprising the more diverse and numerous fossil forms,[96][97] although this classification has been criticized as arbitrary.[98]


Class Cephalopoda
- Subclass † Ellesmeroceratoidea
- Order † Plectronocerida (501 to 490 Ma)
- Order † Protactinocerida
- Order † Yanhecerida
- Order † Ellesmerocerida (500 to 470 Ma)
- Subclass † Endoceratoidea (485 to 430 Ma)
- Order † Endocerida (485 to 430 Ma)
- Order † Intejocerida (485 to 480 Ma)
- Subclass † Actinoceratoidea
- Order † Actinocerida (480 to 312 Ma)
- Subclass Nautiloidea (490.0 Ma- Rec)
- Order † Basslerocerida (490 to 480 Ma)
- Order † Tarphycerida (485 to 386 Ma)
- Order † Lituitida (485 to 480 Ma)
- Order † Discosorida (482 to 392 Ma)
- Order † Oncocerida (478.5 to 324 Ma)
- Order Nautilida (410.5 Ma-Rec)
- Subclass † Orthoceratoidea (482.5 to 211.5 Ma)
- Order † Orthocerida (482.5 to 211.5 Ma)
- Order † Ascocerida (478 to 412 Ma)
- Order † Dissidocerida (479 to 457.5 Ma)
- Order † Bajkalocerida
- Subclass † Bactritoidea (422 to 252 Ma)
- Subclass † Ammonoidea (410 to 66 Ma)
- Subclass Coleoidea (410.0 Ma-rec)[99]
Cladistic classification
Another recent system divides all cephalopods into two clades. One includes nautilus and most fossil nautiloids. The other clade (Neocephalopoda or Angusteradulata) is closer to modern coleoids, and includes belemnoids, ammonoids, and many orthocerid families. There are also stem group cephalopods of the traditional Ellesmerocerida that belong to neither clade.[100][101]
Monophyly of coeloids
The coeloids have been thought to possibly represent a polyphyletic group,[1]:289 although this has not been supported by the rising body of molecular data.[102]
Post-mortem decay
After death, if undisturbed, cephalopods[note 1] decay relatively quickly. Their muscle softens within a couple of days, and may swell; egg sacs can swell so much that they rip through the mantle. Subsequently, the organs shrink again; at this point the organism may start to break up into fragments. The eyes retain their size while the head shrinks around them. The gills may remain swollen at this point. After around a week, the carcass collapses in on itself and begins to disintegrate. The ink sac solidifies around this point. After a fortnight little is left but a blob with eyes, arms and ink sac visible. After a couple of months, these are only recognisable as flattened dark stains - although in some cases the eye lenses can remain intact for up to a year.[75]
In popular culture
Cephalopods, typically octopuses and squids, have been depicted commonly in Western pop culture as creatures that enjoy hugging or latching onto things with their limbs and refusing to release. Some of the most notable uses of cephalopods in popular culture include Squidward Tentacles, Cthulhu, and the character Doctor Octopus, who has arms that are characteristic of a cephalopod, although he is not actually a cephalopod himself.
See also
![]() |
|
- Cephalopod size
- Kraken
- List of nautiloids
- List of ammonites
Notes
- ↑ Experiments were performed on a variety of squid
References
- ↑ 1.0 1.1 1.2 1.3 1.4 1.5 1.6 1.7 1.8 Wilbur, Karl M.; Trueman, E.R.; Clarke, M.R., eds. (1985), The Mollusca, 11. Form and Function, New York: Academic Press, ISBN 0-12-728702-7
- ↑ From Greek τεῦθος (gen. τεύθου) "cuttlefish, squid" — J.M. Arnold, "Natural History of the Squid", p. 1.
- ↑ [updated 13-Jun-2003] [cit. 27-Feb-2005] http://www.cephbase.utmb.edu/spdb/allsp.cfm
- ↑ 4.0 4.1 4.2 4.3 Wilbur, Karl M.; Clarke, M.R.; Trueman, E.R., eds. (1985), The Mollusca, 12. Paleontology and neontology of Cephalopods, New York: Academic Press, ISBN 0-12-728702-7
- ↑ Bartol I.K., Mann R., Vecchione M. (2002). "Distribution of the euryhaline squid Lolliguncula brevis in Chesapeake Bay: effects of selected abiotic factors". Mar. Ecol. Prog. Ser. 226: 235–247. doi:10.3354/meps226235.
- ↑ 6.0 6.1 6.2 6.3 6.4 6.5 6.6 6.7 6.8 6.9 6.10 6.11 Marion Nixon; J.Z. Young (2003). The brains and lives of cephalopods. New York: Oxford University Press. ISBN 0-19-852761-6.
- ↑ Kutsch, Wolfram (1995). The nervous systems of invertebrates: An evolutionary and comparative approach. ISBN 978-3-7643-5076-5.
- ↑ http://www.pnas.org/content/50/4/619.full.pdf
- ↑ 9.0 9.1 Packard, A. (1972). "Cephalopods and Fish: the Limits of Convergence". Biological Reviews 47 (2): 241–307. doi:10.1111/j.1469-185X.1972.tb00975.x.
- ↑ Macia, Silvia; Robinson, Michael P.; Craze, Paul; Dalton, Robert; Thomas, James D. (2004). "New observations on airborne jet propulsion (flight) in squid, with a review of previous reports". Journal Molluscan Studies 70 (3): 297–299. doi:10.1093/mollus/70.3.297.
- ↑ 11.0 11.1 Serb, J. M.; Eernisse, D. J. (2008). "Charting Evolution's Trajectory: Using Molluscan Eye Diversity to Understand Parallel and Convergent Evolution". Evolution Education and Outreach 1 (4): 439–447. doi:10.1007/s12052-008-0084-1.
- ↑ Martin J. Wells (2011). Part M, Chapter 4: Physiology of Coleoids. Lawrence, Kansas, USA. ISSN 2153-4012.(subscription required)
- ↑ 13.0 13.1 13.2 13.3 13.4 13.5 13.6 13.7 13.8 13.9 13.10 13.11 13.12 Boyle, Peter; Rodhouse, Paul (2004). Cephalopods : ecology and fisheries. Ames, Iowa: Blackwell. doi:10.1002/9780470995310.ch2. ISBN 0-632-06048-4.
- ↑ 14.0 14.1 Messenger, John B.; Roger T. Hanlon (1998). Cephalopod Behaviour. Cambridge: Cambridge University Press. pp. 17–21. ISBN 0-521-64583-2.
- ↑ Hanlon and Messenger, 68.
- ↑ Mäthger, L.; Roberts, S.; Hanlon, R. (2010). "Evidence for distributed light sensing in the skin of cuttlefish, Sepia officinalis". Biology Letters 6 (5): 600–603. doi:10.1098/rsbl.2010.0223. PMC 2936158. PMID 20392722.
- ↑ Michinomae, M.; Masuda, H.; Seidou, M.; Kito, Y. (1994). "Structural basis for wavelength discrimination in the banked retina of the firefly squid Watasenia Scintillans". The Journal of experimental biology 193 (1): 1–12. PMID 9317205.
- ↑ Seidou, M.; Sugahara, M.; Uchiyama, H.; Hiraki, K.; Hamanaka, T.; Michinomae, M.; Yoshihara, K.; Kito, Y. (1990). "On the three visual pigments in the retina of the firefly squid, Watasenia scintillans". Journal of Comparative Physiology A 166 (6). doi:10.1007/BF00187321.
- ↑ "The cephalopods can hear you". BBC News. 2009-06-15. Retrieved 2010-04-28.
- ↑ Tong, D.; Rozas, S.; Oakley, H.; Mitchell, J.; Colley, J.; Mcfall-Ngai, J. (Jun 2009). "Evidence for light perception in a bioluminescent organ". Proceedings of the National Academy of Sciences of the United States of America 106 (24): 9836–9841. Bibcode:2009PNAS..106.9836T. doi:10.1073/pnas.0904571106. ISSN 0027-8424. PMC 2700988. PMID 19509343.
- ↑ 21.0 21.1 "integument (mollusks)."Encyclopædia Britannica. 2009. Encyclopædia Britannica 2006 Ultimate Reference Suite DVD
- ↑ Manda, Štěpán; Turek, Vojtěch (2009). "Minute Silurian oncocerid nautiloids with unusual colour patterns". Acta Palaeontologica Polonica 54 (3): 503–512.
- ↑ Colour patterns in Early Devonian cephalopods from the Barrandian Area: Taphonomy and taxonomy Vojtěch Turek Acta Palaeontologica Polonica 54 (3). 2009. pp. 491–502.
- ↑ Roger T. Hanlon, John B. Messenger: Cephalopod Behaviour, page 2. Cambridge University Press, 1999, ISBN 0-521-64583-2
- ↑ Wells, M.J. (1 April 1980). "Nervous control of the heartbeat in octopus". Journal of Experimental Biology 85 (1): 111–28. PMID 7373208.
- ↑ Ghiretti-Magaldi, A. (October 1992). "The Pre-history of Hemocyanin. The Discovery of Copper in the Blood of Molluscs". Cellular and Molecular Life Sciences (Birkhäuser Basel) 48 (10). doi:10.1007/BF01919143.
- ↑ 27.0 27.1 27.2 Daniel L. Gilbert; Adelman, William J.; John M. Arnold (1990). Squid as Experimental Animals (illustrated ed.). Springer. ISBN 978-0-306-43513-3.
- ↑ 28.0 28.1 28.2 "Electron Microscopical and Histochemical Studies of Differentiation and Function of the Cephalopod Gill (Sepia officinalis L.)". Zoomorphologie 93 (3): 193–207. 1979. doi:10.1007/BF00993999.
- ↑ Q. Bone; E. R. Brown (1994). "On the respiratory flow in the cuttlefish Sepia officinalis". Journal of Experimental Biology 194: 153–165.
- ↑ Cole, A. .; Hall, B. . (2009). "Cartilage differentiation in cephalopod molluscs". Zoology (Jena, Germany) 112 (1): 2–15. doi:10.1016/j.zool.2008.01.003. PMID 18722759.
- ↑ See also http://tolweb.org/articles/?article_id=4200
- ↑ 32.0 32.1 32.2 32.3 32.4 Wilbur, Karl M.; Clarke, M.R.; Trueman, E.R., eds. (1985), "11: Evolution of Buoyancy and Locomotion in recent cephalopods", The Mollusca, 12. Paleontology and neontology of Cephalopods, New York: Academic Press, ISBN 0-12-728702-7
- ↑ 33.0 33.1 Anderson, E.; Demont, M. (2000). "The mechanics of locomotion in the squid Loligo pealei: Locomotory function and unsteady hydrodynamics of the jet and intramantle pressure". The Journal of experimental biology 203 (Pt 18): 2851–2863. PMID 10952883.
- ↑ 34.0 34.1 34.2 Bartol, I. K.; Krueger, P. S.; Thompson, J. T.; Stewart, W. J. (2008). "Swimming dynamics and propulsive efficiency of squids throughout ontogeny". Integrative and Comparative Biology 48 (6): 720–733. doi:10.1093/icb/icn043. PMID 21669828.
- ↑ E., S.; M., V. (2002). "Quantification of ontogenetic discontinuities in three species of oegopsid squids using model II piecewise linear regression". Marine Biology 140 (5): 971. doi:10.1007/s00227-001-0772-7.
- ↑ W. Johnson; P. D. Soden; E. R. Trueman. "A Study in Jet Propulsion: An Analysis of the Motion of the Squid, Loligo Vulgaris". Journal of Experimental Biology 56 (1972): 155–165.
- ↑ Campbell, Reece, & Mitchell, p.612
- ↑ Guerra, A.; Martinell, X.; González, A. F.; Vecchione, M.; Gracia, J.; Martinell, J. (2007). "A new noise detected in the ocean". Journal of the Marine Biological Association of the UK 87. doi:10.1017/S0025315407058225.
- ↑ 39.0 39.1 Wells, M.J.; O'Dor, R.K. (July 1991). "Jet Propulsion and the Evolution of the Cephalopods". Bulletin of Marine Science 49 (1): 419–432(14).
- ↑ Chamberlain, Jr, J. (1993). "Locomotion in ancient seas: Constraint and opportunity in Cephalopod adaptive design". Geobios 26 (Suppl. 1): 49–61. doi:10.1016/S0016-6995(06)80360-8.
- ↑ 41.0 41.1 41.2 41.3 41.4 O'Dor, R.K. (1988). "The forces acting on swimming squid". Journal of Experimental Biology 137: 421–442.
- ↑ O'Dor, R. K., and Hoar, J. A. (2000). "Does geometry limit squid growth?". ICES Journal of Marine Science 57: 8–14. doi:10.1006/jmsc.1999.0502.
- ↑ 43.0 43.1 43.2 43.3 Baratte; Andouche, A.; Bonnaud, L. (2007). "Engrailed in cephalopods: a key gene related to the emergence of morphological novelties". Development genes and evolution 217 (5): 353–362. doi:10.1007/s00427-007-0147-2. PMID 17394016.
- ↑ von Boletzky, S. (2004). "“Nude ammonoids”: a challenge to cephalopod phylogeny? [« Ammonoïdes nus » : un défi pour la phylogénie des céphalopodes ?]". Geobios 37: 117–118. doi:10.1016/j.geobios.2003.01.009.
- ↑ R. N. Gibson, R. J. A. Atkinson, J. D. M. Gordon, ed. (2006). Oceanography and Marine Biology: An Annual Review. CRC Press. p. 288. ISBN 1420006398.
- ↑ Aldred, R. G.; Nixon, M.; Young, J. Z. (1983). "Cirrothauma Murrayi Chun, A Finned Octopod". Philosophical Transactions of the Royal Society B: Biological Sciences 301 (1103): 1. Bibcode:1983RSPTB.301....1A. doi:10.1098/rstb.1983.0021.
- ↑ Fuchs, D., C. Ifrim & W. Stinnesbeck (2008). A new Palaeoctopus (Cephalopoda: Coleoidea) from the Late Cretaceous of Vallecillo, north-eastern Mexico, and implications for the evolution of Octopoda. Palaeontology 51(5): 1129–1139. doi:10.1111/j.1475-4983.2008.00797.x
- ↑ Boletzky, Sigurd v. (July 1991). "The Terminal Spine of Sepiolid Hatchlings: Its Development and Functional Morphology (Mollusca, Cephalopoda)". Bulletin of Marine Science 49: 107–112.
- ↑ 49.0 49.1 49.2 49.3 Strugnell, J.; Nishiguchi, M. K. (2007). "Molecular phylogeny of coleoid cephalopods (Mollusca: Cephalopoda) inferred from three mitochondrial and six nuclear loci: a comparison of alignment, implied alignment and analysis methods". Journal of Molluscan Studies 73 (4): 399. doi:10.1093/mollus/eym038.
- ↑ Warnke, K.; Keupp, H. (2005). "Spirula—a window to the embryonic development of ammonoids? Morphological and molecular indications for a palaeontological hypothesis". Facies 51: 60. doi:10.1007/s10347-005-0054-9.
- ↑ Furuhashi, T.; Schwarzinger, C.; Miksik, I.; Smrz, M.; Beran, A. (2009). "Molluscan shell evolution with review of shell calcification hypothesis". Comparative biochemistry and physiology. Part B, Biochemistry & molecular biology 154 (3): 351–371. doi:10.1016/j.cbpb.2009.07.011. PMID 19665573.
- ↑ Dauphin, Y. (1996). "The organic matrix of coleoid cephalopod shells: molecular weights and isoelectric properties of the soluble matrix in relation to biomineralization processes". Marine Biology 125 (3): 525–529. doi:10.1007/BF00353265.
- ↑ Toll, R. B.; Binger, L. C. (1991). "Arm anomalies: Cases of supernumerary development and bilateral agenesis of arm pairs in Octopoda (Mollusca, Cephalopoda)". Zoomorphology 110 (6): 313. doi:10.1007/BF01668021.
- ↑ Anatomy of the Common Squid. 1912.
- ↑ Nixon 1988 in Wippich, M. G. E.; Lehmann, J. (2004). "Allocrioceras from the Cenomanian (mid-Cretaceous) of the Lebanon and its bearing on the palaeobiological interpretation of heteromorphic ammonites". Palaeontology 47 (5): 1093–1107. doi:10.1111/j.0031-0239.2004.00408.x.
- ↑ Wilbur, Karl M.; Clarke, M.R.; Trueman, E.R., eds. (1985), "5", The Mollusca, 12. Paleontology and neontology of Cephalopods, New York: Academic Press, ISBN 0-12-728702-7
- ↑ C.Michael Hogan. 2011. Celtic Sea. eds. P.Saundry & C.Cleveland. Encyclopedia of Earth. National Council for Science and the Environment. Washington DC.
- ↑ Richard E. Young, Michael Vecchione, and Katharina M. Mangold. "Cephalopod radula". Tree of Life web project.
- ↑ 59.0 59.1 59.2 59.3 59.4 Nixon, M. (1995). "A nomenclature for the radula of the Cephalopoda (Mollusca)-living and fossil". Journal of Zoology 236: 73–81. doi:10.1111/j.1469-7998.1995.tb01785.x.
- ↑ 60.0 60.1 Gabbott, S. E. (1999). "Orthoconic cephalopods and associated fauna from the late Ordovician Soom Shale Lagerstatte, South Africa". Palaeontology 42: 123–148. doi:10.1111/1475-4983.00065.
- ↑ Landman, Neil H; Davis, Richard Arnold; Mapes, Royal H (2007). Cephalopods present and past: new insights and fresh perspectives. Springer. ISBN 978-1-4020-6461-6.
- ↑ Richardson & ... (1977). Fossils of the Mason Creek.
- ↑ Kruta, I.; Landman, N.; Rouget, I.; Cecca, F.; Tafforeau, P. (2011). "The Role of Ammonites in the Mesozoic Marine Food Web Revealed by Jaw Preservation". Science 331 (6013): 70–72. Bibcode:2011Sci...331...70K. doi:10.1126/science.1198793. PMID 21212354.
- ↑ 64.0 64.1 Barnes, Robert D. (1982). Invertebrate Zoology. Philadelphia, PA: Holt-Saunders International. pp. 450–451. ISBN 0-03-056747-5.
- ↑ Loest, R. A. (1979). "Ammonia Volatilization and Absorption by Terrestrial Gastropods_ a Comparison between Shelled and Shell-Less Species". Physiological Zoology (The University of Chicago Press) 52 (4): 461–469. doi:10.2307/30155937. JSTOR 30155937.
- ↑ 66.0 66.1 66.2 Boucher-Rodoni, R.; Mangold, K. (1994). "Ammonia production in cephalopods, physiological and evolutionary aspects". Marine and Freshwater Behaviour and Physiology 25: 53. doi:10.1080/10236249409378907.
- ↑ 67.0 67.1 Arkhipkin, A.I. & V.V. Laptikhovsky 2010. Observation of penis elongation in Onykia ingens: implications for spermatophore transfer in deep-water squid. Journal Molluscan Studies, published online on June 30, 2010. doi:10.1093/mollus/eyq019
- ↑ Walker, M. 2010. Super squid sex organ discovered. BBC Earth News, July 7, 2010.
- ↑ 69.0 69.1 Branch George, Branch, Margo and Bannister, Anthony (1981). The Living Shores of Southern Africa. Cape Town: C. Struik. ISBN 0-86977-115-9.
- ↑ Young, R.E., M. Vecchione & K.M. Mangold 1999. Cephalopoda Glossary. Tree of Life web project.
- ↑ Seibel, B. A.; Robison, B. H.; Haddock, S. H. (Dec 2005). "Post-spawning egg care by a squid". Nature 438 (7070): 929. Bibcode:2005Natur.438..929S. doi:10.1038/438929a. ISSN 0028-0836. PMID 16355206.
- ↑ Mapes, R. H.; Nützel, Alexander (2009). "Late Palaeozoic mollusc reproduction: cephalopod egg-laying behavior and gastropod larval palaeobiology". Lethaia 42 (3): 341. doi:10.1111/j.1502-3931.2008.00141.x.
- ↑ Etches, S.; Clarke, J.; Callomon, J. (2009). "Ammonite eggs and ammonitellae from the Kimmeridge Clay Formation (Upper Jurassic) of Dorset, England". Lethaia 42 (2): 204–217. doi:10.1111/j.1502-3931.2008.00133.x. - this reference also contains a good summary of the process of egg development.
- ↑ Norman, M.D. (2000). Cephalopods: A World Guide. ConchBooks.
- ↑ 75.0 75.1 Kear, A.J.; Briggs, D.E.G.; Donovan, D.T. (1995). "Decay and fossilization of non-mineralized tissue in coleoid cephalopods". Palaeontology 38 (1): 105–132. Retrieved 2009-04-21.
- ↑ 76.0 76.1 Shigeno, S.; Sasaki, T.; Moritaki, T.; Kasugai, T.; Vecchione, M.; Agata, K. (Jan 2008). "Evolution of the cephalopod head complex by assembly of multiple molluscan body parts: Evidence from Nautilus embryonic development". Journal of Morphology 269 (1): 1–17. doi:10.1002/jmor.10564. PMID 17654542.
- ↑ Daniel L. Gilbert; William J. Adelman; John M. Arnold (1990). Squid as experimental animals. New York: Plenum Press. ISBN 0-306-43513-6.
- ↑ 78.0 78.1 Von Boletzky, S. (2003). "Biology of early life stages in cephalopod molluscs". Advances in marine biology. Advances in Marine Biology 44: 143–203. doi:10.1016/S0065-2881(03)44003-0. ISBN 978-0-12-026144-4. PMID 12846042.
- ↑ Natalie A. Moltschaniwskyj (2004). "Understanding the process of growth in cephalopods". Marine and Freshwater Research 55 (4): 379–386. doi:10.1071/MF03147.
- ↑ Lemche, H; Wingstrand, K.G. (1959). "The anatomy of Neopilina galatheae Lemche, 1957 (Mollusca, Tryblidiacea)" (Link to free full text + plates). Galathea Rep. 3: 9–73.
- ↑ Wingstrand, KG (1985). "On the anatomy and relationships of Recent Monoplacophora" (Link to free full text + plates). Galathea Rep. 16: 7–94.
- ↑ 82.0 82.1 82.2 Boyle, P.; Rodhouse, P. (2005). "Origin and Evolution". Cephalopods. p. 36. doi:10.1002/9780470995310.ch3. ISBN 9780470995310.
- ↑ Kröger, B. R. (2007). "Some Lesser Known Features of the Ancient Cephalopod Order Ellesmerocerida (Nautiloidea, Cephalopoda)". Palaeontology 50 (3): 565–572. doi:10.1111/j.1475-4983.2007.00644.x.
- ↑ Smith, Martin R and Caron, Jean-Bernard (2010). "Primitive soft-bodied cephalopods from the Cambrian". Nature 465 (7297): 427–428. Bibcode:2010Natur.465..427B. doi:10.1038/465427a. PMID 20505713
- ↑ 85.0 85.1 Kröger, B.; Yun-bai, Y. B. (2009). "Pulsed cephalopod diversification during the Ordovician". Palaeogeography Palaeoclimatology Palaeoecology 273: 174–201. doi:10.1016/j.palaeo.2008.12.015.
- ↑ Dzik, J. (1981). "Origin of the cephalopoda" (PDF). Acta Palaeontologica Polonica 26 (2): 161–191.
- ↑ 87.0 87.1 87.2 87.3 Kröger, B. R.; Servais, T.; Zhang, Y.; Kosnik, M. (2009). "The Origin and Initial Rise of Pelagic Cephalopods in the Ordovician". In Kosnik, Matthew. PLoS ONE 4 (9): e7262. doi:10.1371/journal.pone.0007262. PMC 2749442. PMID 19789709.
- ↑ 88.0 88.1 Holland, C. H. (1987). "The nautiloid cephalopods: a strange success: President's anniversary address 1986". Journal of the Geological Society 144: 1–0. doi:10.1144/gsjgs.144.1.0001.
- ↑ Kröger, Björn (2006). "Early growth-stages and classification of orthoceridan Cephalopods of the Darriwillian (Middle Ordovician) of Baltoscandia". Lethaia 39 (2): 129–139. doi:10.1080/00241160600623749.
- ↑ 90.0 90.1 Young, R.E.; Vecchione, M.; Donovan, D.T. "The evolution of coleoid cephalopods and their present biodivesity and ecology". In Payne, AIL; Lipin'ski, M.R.; Clarke, M.R.; Roeleveld, M.A.C. Cephalopod biodiversity, ecology & evolution. South Afriocan journal of Marine Sciences 20. pp. 393–420.
- ↑ Strugnell, J.; Norman, M.; Jackson, J.; Drummond, A.; Cooper, A. (2005). "Molecular phylogeny of coleoid cephalopods (Mollusca: Cephalopoda) using a multigene approach; the effect of data partitioning on resolving phylogenies in a Bayesian framework". Molecular Phylogenetics & Evolution 37 (2): 426–441. doi:10.1016/j.ympev.2005.03.020. PMID 15935706.
- ↑ Strugnell, J.; Jackson, J.; Drummond, A. J.; Cooper, A. (2006). "Divergence time estimates for major cephalopod groups: evidence from multiple genes". Cladistics 22: 89–96. doi:10.1111/j.1096-0031.2006.00086.x.
- ↑ Carlini, D. B.; Reece, K. S.; Graves, J. E. (2000). "Actin gene family evolution and the phylogeny of coleoid cephalopods (Mollusca: Cephalopoda)". Molecular Biology and Evolution 17 (9): 1353–1370. doi:10.1093/oxfordjournals.molbev.a026419. PMID 10958852.
- ↑ Bergmann, S.; Lieb, B.; Ruth, P.; Markl, J. (2006). "The hemocyanin from a living fossil, the cephalopod Nautilus pompilius: protein structure, gene organization, and evolution". Journal of molecular evolution 62 (3): 362–374. doi:10.1007/s00239-005-0160-x. PMID 16501879.
- ↑ Bather, F.A. (1888b). "Professor Blake and Shell-Growth in Cephalopoda". Annals and Magazine of Natural History. 6 1: 421–426.
- ↑ Shevyrev, A.A. (2005). "The Cephalopod Macrosystem: A Historical Review, the Present State of Knowledge, and Unsolved Problems: 1. Major Features and Overall Classification of Cephalopod Mollusks". Paleontological Journal 39 (6): 606–614. "Translated from Paleontologicheskii Zhurnal No. 6, 2005, 33-42."
- ↑ Shevyrev, A. A. (2006). "The cephalopod macrosystem; a historical review, the present state of knowledge, and unsolved problems; 2, Classification of nautiloid cephalopods". Paleontological Journal 40 (1): 46–54. doi:10.1134/S0031030106010059.
- ↑ Kroger, B. "Peer review in the Russian "Paleontological Journal"".
- ↑ Bather, F.A. (1888a). "Shell-growth in Cephalopoda (Siphonopoda)". Annals and Magazine of Natural History. 6 1: 298–310.
- ↑ Berthold, Thomas, & Engeser, Theo (1987). "Phylogenetic analysis and systematization of the Cephalopoda (Mollusca)". Verhandlungen Naturwissenschaftlichen Vereins in Hamburg. (NF) 29: 187–220.
- ↑ Engeser (1997). "Fossil Nautiloidea Page". Archived from the original on 2006-09-25.
- ↑ Lindgren, A. R.; Giribet, G.; Nishiguchi, M. K. (2004). "A combined approach to the phylogeny of Cephalopoda (Mollusca)". Cladistics 20 (5): 454. doi:10.1111/j.1096-0031.2004.00032.x.
Further reading
- Barskov, I.S., M.S. Boiko, V.A. Konovalova, T.B. Leonova & S.V. Nikolaeva (2008). "Cephalopods in the marine ecosystems of the Paleozoic". Paleontological Journal 42 (11): 1167–1284. doi:10.1134/S0031030108110014. A comprehensive overview of Paleozoic cephalopods.
- Campbell, Neil A., Reece, Jane B., and Mitchell, Lawrence G.: Biology, fifth edition. Addison Wesley Longman, Inc. Menlo Park, California. 1999. ISBN 0-8053-6566-4.
- Felley, J., Vecchione, M., Roper, C. F. E., Sweeney, M. & Christensen, T., 2001-2003: Current Classification of Recent Cephalopoda. National Museum of Natural History: Department of Systematic Biology: Invertebrate Zoology: Cephalopods
- N. Joan Abbott, Roddy Williamson, Linda Maddock. Cephalopod Neurobiology. Oxford University Press, 1995. ISBN 0-19-854790-0
- Marion Nixon & John Z. Young. The brains and lives of Cephalopods. Oxford University Press, 2003. ISBN 0-19-852761-6
- Hanlon, Roger T. & John B. Messenger. Cephalopod Behaviour. Cambridge University Press, 1996. ISBN 0-521-42083-0
- Martin Stevens & Sami Merilaita. Animal camouflage: mechanisms and function. Cambridge University Press, 2011. ISBN 0-521-19911-5
- Rodhouse, P. G.; Nigmatullin, Ch. M. (1996). "Role as Consumers". Philosophical Transactions of the Royal Society B: Biological Sciences 351 (1343): 1003–1022. doi:10.1098/rstb.1996.0090.
- Classification key to modern cephalopods: ftp://ftp.fao.org/docrep/fao/009/a0150e/a0150e03.pdf
External links
![]() |
Wikispecies has information related to: Cephalopoda |
![]() |
The Wikibook Dichotomous Key has a page on the topic of: Cephalopoda |
- CephBase – cephalopod database
- TONMO.COM – The Octopus News Magazine Online – cephalopod articles and discussion
- Tree of Life Web Project – Cephalopoda
- Mikko's Phylogeny Tree
- Fish vs. Cephalopods
- Will Fast Growing Squid Replace Slow Growing Fish?
- Biomineralisation in modern and fossil cephalopods
- Scientific American: Can a Squid Fly Out of the Water?
- Roger Hanlon's seminar: Rapid Adaptive Camouflage and Signaling in Cephalopods