Hypergiant

A hypergiant (luminosity class 0) is a star with an enormous mass and luminosity, showing signs of a very high rate of mass loss.
Definition
The word "hypergiant" is commonly used as a loose term for the most luminous stars found, even though there are more precise definitions. In 1956, the astronomers Feast and Thackeray used the term super-supergiant (later changed into hypergiant) for stars with an absolute magnitude brighter than MV = −7 (MBol will be larger for very cool and very hot stars, for example at least −9.7 for a B0 hypergiant). In 1971, Keenan suggested that the term would be used only for supergiants showing at least one broad emission component in Hα, indicating an extended stellar atmosphere or a relatively large mass loss rate. The Keenan criterion is the one most commonly used by scientists today.[1] Additionally, hypergiants are expected to have characteristic broadening and red-shifting of their spectral lines producing a distinctive shape known as a P Cygni profile. The use of hydrogen emission is not helpful for defining the coolest hypergiants, and these are largely classified on luminosity since mass loss is almost inevitable for the class.
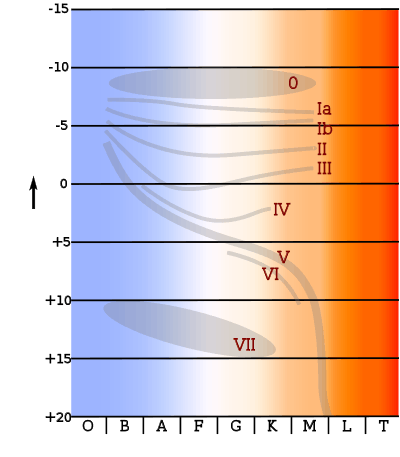
Many astronomers do not use the term hypergiant, except occasionally for specific well-defined groups such as the yellow hypergiants, so it is common to see the term RSG (red supergiant) or B(e) supergiant (blue supergiant with emission spectra) being used to refer to stars that this article defines as hypergiants. There is an MKK luminosity class 0 (zero) for hypergiants, but this is rarely seen in published spectral classifications. More commonly, hypergiants will be classed as Ia-0, Ia+, or even just Iae based solely on the observed spectra. As noted, red supergiants rarely receive these extra spectral classifications. Initial observation of a highly luminous star is insufficient for it to be defined as a hypergiant. That requires the detection of the spectral signatures of atmospheric instability and high mass loss. So it is quite possible for non-hypergiant supergiant stars to have the same or higher luminosity as a hypergiant of the same spectral class.
Formation
Stars with an initial mass above about 25 M☉ quickly move away from the main sequence and increase somewhat in luminosity to become blue supergiants. They cool and enlarge at approximately constant luminosity to become a red supergiant, then contract and increase in temperature as the outer layers are blown away. They may "bounce" backwards and forwards executing one or more "blue loops", still at a fairly steady luminosity, until they explode as a supernova or completely shed their outer layers to become a Wolf–Rayet star. Stars with an initial mass above about 40 M☉ are simply too luminous to develop a stable extended atmosphere and so they never cool sufficiently to become red supergiants. The most massive stars, especially rapidly rotating stars with enhanced convection and mixing, may skip these steps and move directly to the Wolf-Rayet stage.
This means that stars at the top of the HR diagram where hypergiants are found may be newly evolved from the main sequence and still with high mass, or much more evolved post-red supergiant stars that have lost a significant fraction of their initial mass, and these objects cannot be distinguished simply on the basis of their luminosity and temperature. High mass stars with a high proportion of remaining hydrogen are more stable, while older stars with lower masses and a higher proportion of heavy elements have less stable atmospheres due to increased radiation pressure and decreased gravitational attraction. These are thought to be the hypergiants, near the Eddington limit and rapidly losing mass.
The yellow hypergiants are thought to be generally post-red supergiant stars that have already lost most of their atmospheres and hydrogen. A few more stable high mass yellow supergiants with approximately the same luminosity are known and thought to be evolving towards the red supergiant phase, but these are rare as this is expected to be a rapid transition. Because yellow hypergiants are post-red supergiant stars, there is a fairly hard upper limit to their luminosity at around 500,000 - 750,000 L☉, but blue hypergiants can be much more luminous, sometimes several million L☉.
Almost all hypergiants exhibit variations in luminosity over time due to instabilities within their interiors, but these are small except for two distinct instability regions where luminous blue variables (LBVs) and yellow hypergiants are found. Because of their high masses, the lifetime of a hypergiant is very short in astronomical timescales: only a few million years compared to around 10 billion years for stars like the Sun. Hypergiants are only created in the largest and densest areas of star formation and because of their short lives, only a small number are known despite their extreme luminosity that allows them to be identified even in neighbouring galaxies. The time spent in some phases such as LBVs can be as short as a few thousand years.[2][3]
Stability

As the luminosity of stars increases greatly with mass, the luminosity of hypergiants often lies very close to the Eddington limit, which is the luminosity at which the radiation pressure expanding the star outward equals the force of the star's gravity collapsing the star inward. This means that the radiative flux passing through the photosphere of a hypergiant may be nearly strong enough to lift off the photosphere. Above the Eddington limit, the star would generate so much radiation that parts of its outer layers would be thrown off in massive outbursts; this would effectively restrict the star from shining at higher luminosities for longer periods.
A good candidate for hosting a continuum-driven wind is Eta Carinae, one of the most massive stars ever observed. With an estimated mass of around 130 solar masses and a luminosity four million times that of the Sun, astrophysicists speculate that Eta Carinae may occasionally exceed the Eddington limit.[4] The last time might have been a series of outbursts observed in 1840–1860, reaching mass loss rates much higher than our current understanding of what stellar winds would allow.[5]
As opposed to line-driven stellar winds (that is, ones driven by absorbing light from the star in huge numbers of narrow spectral lines), continuum driving does not require the presence of "metallic" atoms — atoms other than hydrogen and helium, which have few such lines — in the photosphere. This is important, since most massive stars also are very metal-poor, which means that the effect must work independently of the metallicity. In the same line of reasoning, the continuum driving may also contribute to an upper mass limit even for the first generation of stars right after the Big Bang, which did not contain any metals at all.
Another theory to explain the massive outbursts of, for example, Eta Carinae is the idea of a deeply situated hydrodynamic explosion, blasting off parts of the star’s outer layers. The idea is that the star, even at luminosities below the Eddington limit, would have insufficient heat convection in the inner layers, resulting in a density inversion potentially leading to a massive explosion. The theory has, however, not been explored very much, and it is uncertain whether this really can happen.[6]
Another theory associated with hypergiant stars is the potential to form a pseudo-photosphere, that is a spherical optically dense surface that is actually formed by the stellar wind rather than being the true surface of the star. Such a pseudo-photosphere would be significantly cooler than the deeper surface below the outward-moving dense wind. This has been hypothesized to account for the "missing" intermediate-luminosity LBVs and the presence of yellow hypergiants at approximately the same luminosity and cooler temperatures. The yellow hypergiants are actually the LBVs having formed a pseudo-photosphere and so apparently having a lower temperature.[7]
Relationships with Ofpe, WNL, LBV, and other supergiant stars
Hypergiants are evolved, high luminosity, high mass stars that occur in the same or similar regions of the HR diagram to stars with different classifications. It isn't always clear whether the different classifications represent stars with different initial conditions, stars at different stages of an evolutionary track, or is just an artifact of our observations. Model details vary[8][9] but there are many areas of agreement. Some of these distinctions are not necessarily helpful in establishing relationships between different types of stars or the differences between them since they have been developed based on differing criteria and for different purposes.
Although most supergiant stars are less luminous than hypergiants of the same temperature, a few fall in the same luminosity range. Ordinary supergiants lack the strong H emission and broadened spectral lines that indicate rapid mass loss in the hypergiants. Lower mass supergiants do not return from the red supergiant phase, either exploding as supernovae or leaving behind a white dwarf.
Luminous blue variables are a class of highly luminous hot stars that display characteristic spectral variation. They often lie in a "quiescent" zone with hotter stars generally being more luminous, but periodically undergo large surface eruptions and move to a narrow zone where stars of all luminosities have approximately the same temperature, around 8,000K. This "active" zone is near the hot edge of the unstable "void" where yellow hypergiants are found, with some overlap. It isn't clear whether yellow hypergiants ever manage to get past the instability void to become LBVs or explode as a supernova.
Blue hypergiants are found in the same parts of the HR diagram as LBVs but do not necessarily show the LBV variations. Some but not all LBVs show the characteristics of hypergiant spectra at least some of the time, but many authors would exclude all LBVs from the hypergiant class and treat them separately. Blue hypergiants that don't show LBV characteristics may be progenitors of LBVs, or vice versa, or both. Lower mass LBVs may be a transitional stage to or from cool hypergiants or are different type of object.
Wolf-Rayet stars are extremely hot stars that have lost much or all of their outer layers. WNL is a term used for late stage (i.e. cooler) Wolf-Rayet stars with spectra dominated by nitrogen. Although these are generally thought to be the stage reached by hypergiant stars after sufficient mass loss, it is possible that a small group of hydrogen-rich WNL stars are actually progenitors of blue hypergiants or LBVs. These are the closely related Ofpe (O-type spectra plus H, He, and N emission lines, and other peculiarities) and WN9 (the coolest nitrogen Wolf-Rayet stars) which may be a brief intermediate stage between high mass main sequence stars and hypergiants or LBVs. Quiescent LBVs have been observed with WNL spectra and apparent Ofpe/WNL stars have changed to show blue hypergiant spectra. High rotation rates cause massive stars to shed their atmospheres quickly and prevent the passage from main sequence to supergiant, so these directly become Wolf-Rayet stars. Wolf Rayet stars, slash stars, cool slash stars (aka WN10/11), Ofpe, Of+, and Of* stars are not considered hypergiants. Although they are luminous and often have strong emission lines, they have characteristic spectra of their own.
Known hypergiants
Hypergiants are difficult to study due to their rarity. Many hypergiants have highly variable spectra, but they are grouped here into broad spectral classes.
Luminous blue variables
Some luminous blue variables are classified as hypergiants, during at least part of their cycle of variation:
- Eta Carinae, inside the Keyhole Nebula (NGC 3372) in the southern constellation of Carina. Eta Carinae is extremely massive, possibly as much as 120 to 150 times the mass of the Sun, and is four to five million times as luminous. Possibly a different type of object from the LBVs, or extreme for a LBV.
- P Cygni, in the northern constellation of Cygnus. Prototype for the characteristic LBV spectral lines.
- S Doradus, in a nearby galaxy called the Large Magellanic Cloud, in the southern constellation of Dorado. Prototype variable, LBVs are still often called S Doradus variables.
- The Pistol Star (V4627 Sgr), near the center of the Milky Way, in the constellation of Sagittarius. The Pistol Star is possibly as much as 150 times more massive than the Sun, and is about 1.7 million times more luminous.
- LBV 1806-20 in the cluster Cl* 1806-20 on the other side of the Milky Way galaxy.
- V4029 Sagittarii
- V905 Scorpii
- HD 269700,[7][10] R116 in the LMC
- HD 6884,[11] (R40 in SMC)
Blue hypergiants
Usually B-class, occasionally late O or early A:
- Zeta¹ Scorpii, the brightest star of the OB association Scorpius OB1 and a LBV candidate.
- V1429 Aquilae, (= MWC 314) in the constellation of Aquila, LBV candidate with a supergiant companion.
- V430 Scuti[12]
- V452 Scuti, poorly studied LBV candidate[13]
- HD 80077, LBV candidate[12]
- Cygnus OB2-12, which some authors consider an LBV because of its extreme luminosity, although it has not shown the characteristic variability.
- HDE 269128 (R81 in LMC), LBV candidate, eclipsing binary system.[14]
- HD 268835 (R66 in LMC)
- V4030 Sagittarii
- V1768 Cygni[12]
- BP Crucis[12]
- HT Sagittae[12]
- V2140 Cygni[12]
- HD 37974[15] (R126 in LMC)
- HD 32034[16] (R62 in LMC)
- HD 269781[16] (in LMC)
- HD 269661[16] (R111 in LMC)
- HD 269604[16] (in LMC)
In Westerlund 1:[17]
- W5 (possible Wolf-Rayet)
- W7
- W13 (binary?)
- W33
- W42a
In Galactic Center Region:[18]
- Star 13, type O, LBV candidate
- Star 18, type O, LBV candidate
Yellow hypergiants
Yellow hypergiants with late A -K spectra.
- Rho Cassiopeiae, in the northern constellation of Cassiopeia, is about 500,000 times as luminous as the Sun.
- V509 Cassiopeiae
- HD 33579 (in LMC)
- IRC+10420 (V1302 Aql)
- IRAS 18357-0604[19]
- HD 7583 (R45 in SMC)
- V766 Centauri (=HR5171A)[20]
- V1427 Aquilae, may just be a closer post-AGB star[21]
- IRAS 17163-3907[22][23]
- V382 Carinae
- Variable A (in M33)
- HD 268757[15] (R59 in LMC)
In Westerlund 1:[17]
- W4
- W8a
- W12a
- W16a
- W32
- W265
Plus at least two probable cool hypergiants in the recently discovered Scutum Red Supergiant Clusters: F15 and possibly F13 in RSGC1 and Star 49 in RSGC2.
Red hypergiants

M type spectra, the largest known stars.
- NML Cygni
- WOH G64
- VX Sagittarii
- S Persei
- RW Cephei
- VY Canis Majoris
- Mu Cephei
- KW Sagittarii
- PZ Cassiopeiae
- V354 Cephei
A survey expected to capture virtually all Magellanic Cloud red hypergiants[24] detected around a dozen M class stars Mv−7 and brighter, around a quarter of a million times more luminous than the sun, and from about 1,000 times the radius of the sun upwards.
See also
References
- ↑ de Jager, C. (1998). "The Yellow Hypergiants". The Astronomy and Astrophysics Review 8 (3): 145–180. Bibcode:1998A&ARv...8..145D. doi:10.1007/s001590050009.
- ↑ Cyril Georgy; Sylvia Ekström; Georges Meynet; Philip Massey; Levesque; Raphael Hirschi; Patrick Eggenberger; André Maeder (2012). "Grids of stellar models with rotation II. WR populations and supernovae/GRB progenitors at Z = 0.014". arXiv:1203.5243v2 [astro-ph.SR].
- ↑ Brott, I.; Evans, C. J.; Hunter, I.; De Koter, A.; Langer, N.; Dufton, P. L.; Cantiello, M.; Trundle, C.; Lennon, D. J.; De Mink, S. E.; Yoon, S. -C.; Anders, P. (2011). "Rotating massive main-sequence stars". Astronomy & Astrophysics 530: A116. arXiv:1102.0766. Bibcode:2011A&A...530A.116B. doi:10.1051/0004-6361/201016114.
- ↑ Owocki, S. P.; Van Marle, Allard Jan (2007). "Luminous Blue Variables & Mass Loss near the Eddington Limit". Proceedings of the International Astronomical Union 3: 71–83. arXiv:0801.2519. Bibcode:2008IAUS..250...71O. doi:10.1017/S1743921308020358.
- ↑ Owocki, S. P.; Gayley, K. G.; Shaviv, N. J. (2004). "A porosity-length formalism for photon-tiring limited mass loss from stars above the Eddington limit". The Astrophysical Journal 616 (1): 525–541. arXiv:astro-ph/0409573. Bibcode:2004ApJ...616..525O. doi:10.1086/424910.
- ↑ Smith, N.; Owocki, S. P. (2006). "On the role of continuum driven eruptions in the evolution of very massive stars and population III stars". The Astrophysical Journal 645 (1): L45–L48. arXiv:astro-ph/0606174. Bibcode:2006ApJ...645L..45S. doi:10.1086/506523.
- ↑ 7.0 7.1 Vink, J. S. (2012). "Eta Carinae and the Luminous Blue Variables". Eta Carinae and the Supernova Impostors. Astrophysics and Space Science Library 384. pp. 221–247. doi:10.1007/978-1-4614-2275-4_10. ISBN 978-1-4614-2274-7.
- ↑ Langer, R. B.; Garcia-Segura, G. (1996). "Stars and Galaxies". The Astrophysical Journal. 11,57.
- ↑ Stothers, N.; Chin, C.-W. (1996). "Evolution of Massive Stars into Luminous Blue Variables and Wolf-Rayet stars for a range of metallicities". Reviews in Modern Astronomy. 468:842–850.
- ↑ Bibcode: 1999A&A...349..537V
- ↑ Van Genderen, C. (1998). "Cyclicities in the Light Variations of Luminous Blue Variables II. R40 developing an S Doradus phase". Astronomy and Astrophysics (Astronomy & Astrophysics) 333: 565. Bibcode:1998A&A...333..565S. More than one of
|author1=
and|last=
specified (help) - ↑ 12.0 12.1 12.2 12.3 12.4 12.5 Clark, J. S.; Najarro, F.; Negueruela, I.; Ritchie, B. W.; Urbaneja, M. A.; Howarth, I. D. (2012). "On the nature of the galactic early-B hypergiants". Astronomy & Astrophysics (pdfarXiv:1202.3991v1. Bibcode:2012A&A...541A.145C. doi:10.1051/0004-6361/201117472. ) 541: A145.
- ↑ Miroshnichenko, A. S.; Chentsov, E. L.; Klochkova, V. G. (2000). "AS314: A dusty A-type hypergiant". Astronomy and Astrophysics Supplement Series 144 (3): 379. Bibcode:2000A&AS..144..379M. doi:10.1051/aas:2000216.
- ↑ Bibcode: 2000ASPC..204...43W
- ↑ 15.0 15.1 "Light variations of α Cygni variables in the Magellanic Clouds". The Journal of Astrophysical Data. Retrieved 2012-05-21.
- ↑ 16.0 16.1 16.2 16.3 Neugent; Philip Massey; Brian Skiff; Georges Meynet (1970). "Yellow and Red Supergiants in the Magellanic Clouds". arXiv:1202.4225 [astro-ph.SR].
- ↑ 17.0 17.1 Clark, J. S.; Negueruela, I.; Crowther, P. A.; Goodwin, S. P. (2005). "On the massive stellar population of the super star cluster Westerlund 1". Astronomy and Astrophysics 434 (3): 949. arXiv:astro-ph/0504342. Bibcode:2005A&A...434..949C. doi:10.1051/0004-6361:20042413.
- ↑ Stolovy, S. R. (2010). "Isolated Wolf-Rayet Stars and O Supergiants in the GalacticCenter Region Identified via Paschen-a Excess". Astronomy & Astrophysics (pdfarXiv:1009.2769v3.pdf. ).
- ↑ Clark, J. S.; Negueruela, I.; Gonzalez-Fernandez, C. (2013). "IRAS 18357-0604 - an analogue of the galactic yellow hypergiant IRC +10420?". arXiv:1311.3956v1 [astro-ph.SR].
- ↑ Schuster, M. T.; Humphreys, R. M.; Marengo, M. (2006). "The Circumstellar Environments of NML Cygni and the Cool Hypergiants". The Astronomical Journal 131: 603. arXiv:astro-ph/0510010. Bibcode:2006AJ....131..603S. doi:10.1086/498395.
- ↑ Jura, M.; Velusamy, T.; Werner, M. W. (2001). "What Next for the Likely Presupernova HD 179821?". The Astrophysical Journal 556: 408. arXiv:astro-ph/0103282. Bibcode:2001ApJ...556..408J. doi:10.1086/321553.
- ↑ "'Fried Egg' Nebula Cracks Open Rare Hypergiant Star". Space.com. 28 September 2011. Retrieved 2012-03-21.
- ↑ "Feast your Eyes on the Fried Egg Nebula". European Southern Observatory. 28 September 2011. Retrieved 2012-03-21.
- ↑ Levesque, E. M.; Massey, P.; Olsen, K. A. G.; Plez, B.; Meynet, G.; Maeder, A. (2006). "The Effective Temperatures and Physical Properties of Magellanic Cloud Red Supergiants: The Effects of Metallicity". The Astrophysical Journal 645 (2): 1102. arXiv:astro-ph/0603596. Bibcode:2006ApJ...645.1102L. doi:10.1086/504417.
|
|